Dynamic BMP signaling polarized by Toll patterns the dorsoventral axis in a hemimetabolous insect
Figures
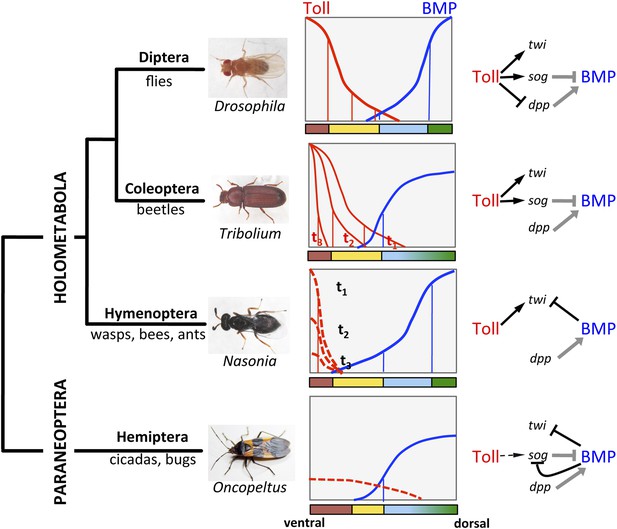
The evolution of Toll's role in dorsoventral (DV) patterning in insects.
In holometabolous insects Toll signaling is activated by ventral eggshell cues and forms an activity gradient (red) that is essential at the very least for specifying the ventral-most cells on the DV axis, giving rise to the mesoderm (brown), by activating the gene twist (twi) (black arrow). In the fly Drosophila Toll signaling not only determines the mesoderm, but also the neuroectoderm (yellow) and restricts BMP signaling to the dorsal side through several parallel mechanisms, including the activation of the BMP inhibitor short gastrulation (sog) (black arrow) and repression of the major BMP ligand decapentaplegic (dpp) (black T-bar) (Hong et al., 2008; Reeves and Stathopoulos, 2009). On the dorsal side a BMP gradient (blue) is established (gray arrow and T-bar indicate BMP ligand production and inhibition, respectively) that specifies non-neurogenic ectoderm (blue) and extraembryonic tissue (green) (O'Connor et al., 2006). Toll signaling is dynamic in Tribolium and polarizes BMP signaling only by activating sog (Nunes da Fonseca et al., 2008). BMP signaling in turn has an increased role in ectodermal patterning compared to flies (van der Zee et al., 2006). In contrast to both Drosophila and Tribolium Toll signaling in the wasp Nasonia appears to be restricted to a narrow ventral region where it is only transiently active. Here, Toll signaling is required to induce mesodermal and mesectodermal fates. But the size of the mesodermal region as well as the fate and position of all other regions along the DV axis are determined by a BMP signaling gradient emanating from the dorsal side by an unknown (Toll-independent) mechanism (black T-bar indicates repression of twi) (Özüak et al., 2014a, 2014b). Thus, in the holometabolous insects BMP signaling gets increasingly more important towards basally branching groups, while Toll's role is diminished, but remains essential for ventral-most cell fates. Here we provide evidence that the bug Oncopeltus, representing the Hemiptera within the sister group of Holometabola (Paraneoptera), uses Toll signaling only as spatial cue (dashed black arrow) to polarize a dynamic BMP signaling network that establishes a gradient responsible for patterning the cell fates along the DV axis. The key regulatory element of this network is the transcriptional repression of sog by BMP signaling. A reaction-diffusion model which incorporates this regulatory element shows that the formation of stable BMP gradients requires only weakly polarized Toll signaling (Box 1).
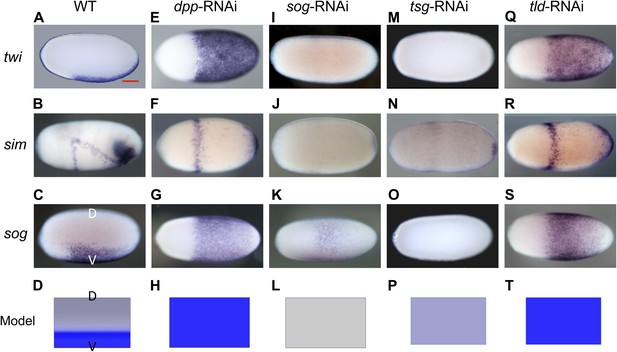
Knockdown (KD) of BMP signaling components results in completely ventralized (dpp-, tld-RNAi) or completely dorsalized (sog-, tsg-RNAi) embryos.
Expression of twi (A, E, I, M, Q), sim (B, F, J, N, R) and sog (C, G, K, O, S) in wild type (wt) embryos (A–C), dpp-RNAi embryos (E–G), sog-RNAi embryos (I–K), tsg-RNAi embryos (M–O) and tld-RNAi embryos (Q–S) monitored by whole mount in situ hybridization (ISH). The view is lateral with the dorsal side pointing up (A–C), ventral (K), or not determined as the expression is DV-symmetric (E–G, I, J, M–O, Q–S). Embryos are at the blastoderm stage (∼26–32 hpf: A, C, E–G, I–K, M, O, Q, S), or at the beginning of anatrepsis (posterior invagination of the embryo, ∼33–37 hpf) (B). Scale bar (A) corresponds to 200 µm. For phenotype frequencies and confirmation of KD see Figure 2—figure supplement 2 and Figure 5—figure supplement 1. (D, H, L, P, T) Simulations of the reaction diffusion system described in Box 1 on a two-dimensional cylinder (Figure 10). Depicted is one half of the cylinder surface stretching from the dorsal (D) to the ventral (V) midline. Blue: sog expression (η). Gray: BMP concentration (b). (D) In wt sog expression is confined to a ventral stripe. (H) Loss of BMP (b = 0) leads to uniform derepression of sog. (L) Loss of sog (s = 0) leads to uniformly high levels of BMP. (P) Loss of Tsg was modeled by assuming that no Sog-BMP complexes are formed (k+ = 0). This results in high BMP signaling throughout the embryo. (T) Loss of Tld was modeled by reducing the degradation constant of Sog (αs) by 90%. As Sog-BMP complexes are not degraded, BMP is not released, causing uniform derepression of sog.
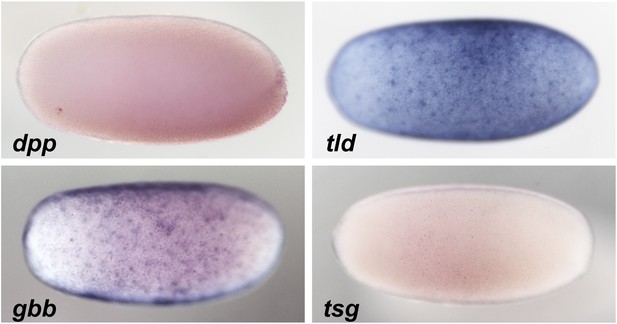
Expression of BMP signaling components during blastoderm.
Anterior is to the left. DV orientation cannot be determined at this stage. The expression of dpp and tsg cannot be detected by ISH during blastoderm stages between 26 to 28 hpf. gbb and tld show uniform expression along the embryonic circumference.
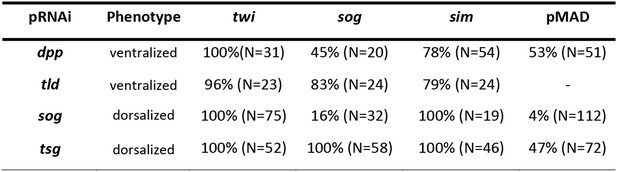
Phenotype frequencies after parental RNAi.
The KD of dpp and tld by parental RNAi caused partial or complete ventralization. No wt embryos were observed. Completely ventralized embryos show a uniform expansion of twi, sog, or the ventral-anterior sim domain (analyzed by ISH) and lack detectable pMAD staining (analyzed by immunostaining). In partially ventralized embryos marker gene expansion was not complete and residual pMAD staining could be detected. Each marker was analyzed separately. The KD of sog and tsg caused partial or complete dorsalization. Completely dorsalized embryos lack twi, sog or sim (except for the terminal domain) and exhibit uniform levels of nuclear localized pMAD levels. Partially dorsalized embryos show residual twi, sog or sim expression and their nuclear pMAD levels are not completely uniform. No wt-like patterns were observed. The table shows % of complete ventralization or complete dorsalization.
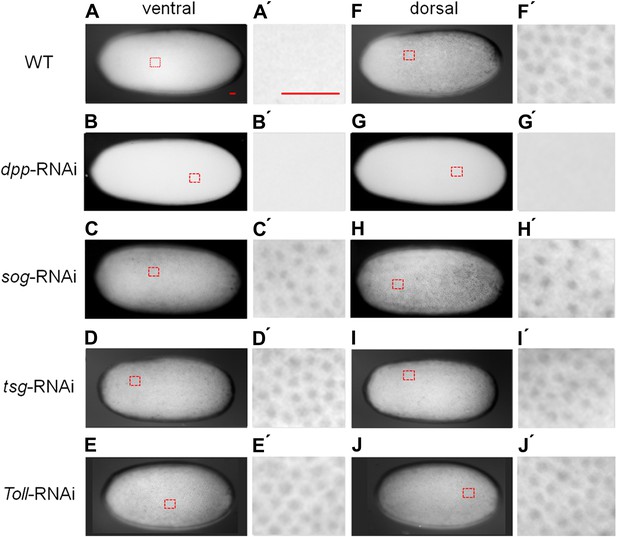
BMP signaling activity is uniformly abolished or expanded in ventralized or dorsalized phenotypes, respectively.
pMAD distribution in blastoderm stage (26–32 hr post fertilization, hpf) wt (A, A′, F, F′), dpp-RNAi (B, B′, G, G′), sog-RNAi (C, C′, H, H′), tsg-RNAi (D, D′, I, I′) and Toll1-RNAi (E, E′, J, J′) embryos. For each embryo a ventral and a dorsal view, or views from opposite sides if DV polarity is lacking (B–G, D-J) are shown. Magnified surface views to the right of each embryo (x') reveal the presence or absence of pMAD in individual nuclei. The scale bar (A, A′) corresponds to 50 µm. For identifying the polarity of the DV axis and for BMP signaling activity during later development see Figure 3—figure supplement 1.
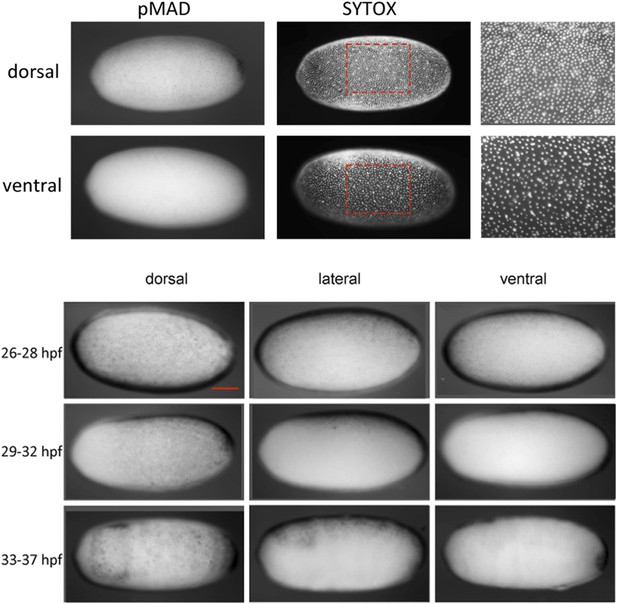
Nuclear density and late pMAD distribution identify the dorsal side of Oncopeltus blastoderm embryos.
Top: the embryo was stained for pMAD and nucleic acids (SYTOX) to visualize the distribution of nuclei. Differences in nuclear density can be used to detect the polarity of the DV axis. At the dorsal side nuclear density is higher than at the ventral side. pMAD nuclear accumulation is found in regions of higher nuclear density, indicating that pMAD can be used as a marker for the dorsal region. Bottom: pMAD distribution at mid-blastoderm (∼26–28 hpf), late blastoderm (∼29–32 hpf) and at the beginning of the posterior invagination (anatrepsis) (∼33–37 hpf). For each embryo a dorsal, lateral and ventral view is shown. The pMAD distribution is progressively confined to the dorsal side. At the beginning of anatrepsis pMAD is restricted to a dorsal domain comprising approximately 30% of the embryonic circumference. The scale bar corresponds to 200 µm.
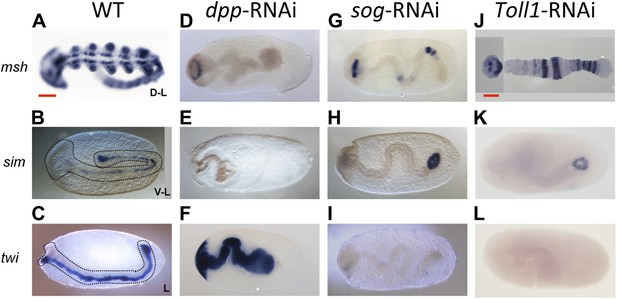
Late phenotypes of dpp, sog and Toll1 KD embryos.
Expression of msh (top row), sim (center row), and twi (bottom row) in wt (A–C), dpp-RNAi (D–F), sog-RNAi (G–I) and Toll1-RNAi (J–L) embryos monitored by ISH. The anterior of the embryo is on the left. Embryos are at the germ band stage (∼40–48 hpf). msh: in wt germ band stage embryos msh is expressed in the dorsal-most part of the CNS and in the mesoderm of the limb buds (dorsal-lateral view). dpp-RNAi germ band embryos lack msh expression except for an anterior domain. sog- or Toll1-pRNAi embryos have a tube-like appearance lacking mesoderm and limb buds. Along these tubes msh is either not expressed or it is expressed at uniform levels around the entire circumference. This indicates that the ectoderm of sog- and Toll1 KD embryos is dorsalized either at the level of the dorsal non-neurogenic or the dorsal-most neurogenic ectoderm. sim: in wt germ band stage embryos sim is expressed along the ventral midline (ventral-lateral view). Upon dpp-, sog- or Toll1-pRNAi, sim expression is lacking except for a ring of expression at the posterior tip of the growth zone in sog-RNAi and Toll1-RNAi embryos. This indicates that the ventral neuroectoderm is lost in these KD embryos. twi: in germ band stage embryos twi is expressed in the invaginated mesoderm, which forms initially a cord within the embryo (lateral view). In dpp-RNAi embryos twi is expressed in the entire germ band indicating complete mesodermalization. In sog-and Toll1-RNAi embryos twi is not expressed. This, in addition to the loss of sim expression, indicates that sog and Toll1 KD embryos consistently lack ventral cell fates along their entire AP axis. Scale bar corresponds to 200 µm.
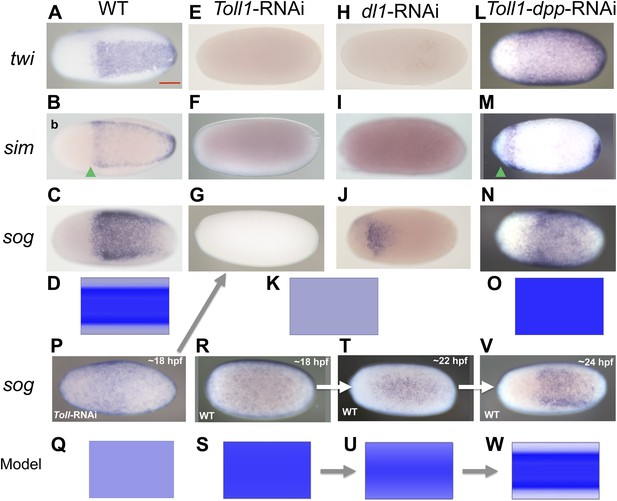
BMP signaling is epistatic to Toll signaling in Oncopeltus.
Expression of twi (A, E, H, L), sim (B, F, I, M), sog (C, G, J, N, P, R, T, V) in wt embryos (A–C, R, T, V), Toll1-RNAi embryos (E–G, P), dl1-RNAi embryos (H–J) and Toll1-dpp-RNAi embryos (L–N) monitored by ISH. The view is ventral (A–C, J, T, V), or not determined as the expression is DV symmetric (E–G, H, I, L–N, P, R). Embryos are at the blastoderm stage (A–C, E–G, H–J, L–N: 26–32 hpf; P–V see figure labels). Green arrowheads mark the anterior border of sim expression. The scale bar (A) corresponds to 200 µm. For phenotype frequencies and confirmation of KD see Figure 5—figure supplement 1. (D, K, O, Q, S, U, W) Simulations of the reaction diffusion system described in Box 1 on a two-dimensional cylinder (Figure 10). Depicted is the ventral part of the cylinder. Blue: sog expression level (η). Gray: BMP concentration (b). (D) wt: sog expression is confined to a ventral stripe. (K) Upon loss of active NF-κB/Dorsal (d = 0) due to either KD of Toll1 or KD of dl1, early activation of sog (P) is insufficient to initiate patterning resulting in uniformly high BMP signaling. (O) Upon simultaneous loss of Dorsal (d = 0) and BMP (b = 0) sog activation is possible despite lack of NF-κB/Dorsal; however, activation is uniform. (Q) sog activation at early stages in the absence of Toll signaling (d = 0). This reflects ηo, NF-κB/Dorsal-independent sog activation (Box 1). (S, U, W) Developmental progression of sog activation (η) during blastoderm stages.
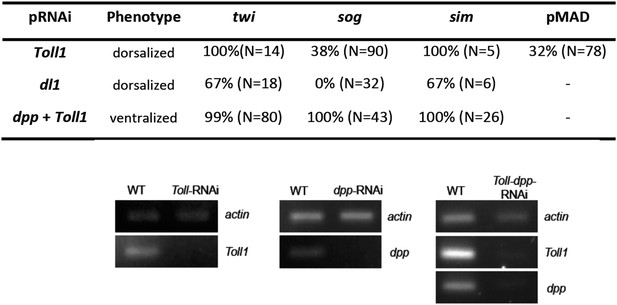
Phenotype frequencies and transcript levels after RNAi.
Top: the KD of Toll and dl1 caused partial or complete dorsalization. Completely dorsalized embryos lack twi, sog or sim (except for the terminal domain) expression and exhibit uniform levels of nuclear localized pMAD levels. Partially dorsalized embryos show residual twi, sog or sim expression and their nuclear pMAD levels are not completely uniform. No wt-like patterns were observed. The table shows % of complete ventralization or complete dorsalization. The double KD of dpp plus Toll by parental RNAi caused complete ventralization. Completely ventralized embryos show a uniform expansion of twi, sog, or the ventral-anterior sim domain (analyzed by ISH). Each marker was analyzed separately. Bottom: expression of actin 5C, dpp and/or Toll1 in Toll1-RNAi, dpp-RNAi, Toll1-dpp-RNAi compared to wt (left column) detected by gel electrophoresis after semi-qPCR.

Toll signaling affects AP patterning.
Expression of msh is monitored by ISH in blastoderm embryos. The view is lateral (A), or not determined as the expression is DV symmetric (B–D). The red arrowheads mark the posterior border of msh expression which is positioned at approximately 60% egg length (0% posterior pole) in wt (A) and tsg KD (D) embryos. In Toll1 and dl1 KD embryos, the msh domain expands to the anterior tip of the embryo and its posterior border is shifted anteriorly (to approximately 80% egg length).
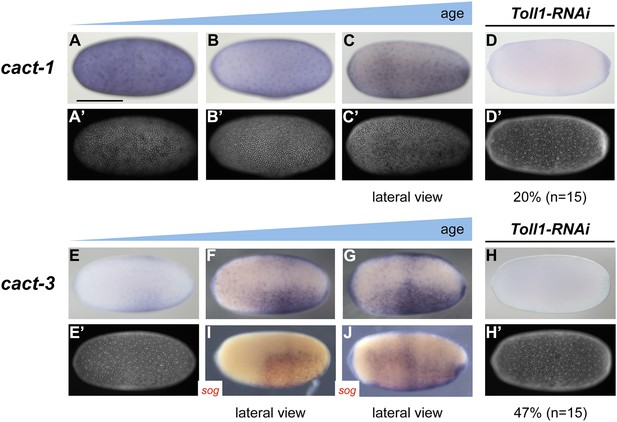
Expression of cact1 and cact3.
Expression of cact1 (A–D) and cact3 (E–H) are monitored by ISH with embryos at early to late blastoderm stages (20–32 hpf). (A′–D′, E′, H′) SYTOX Green staining shows nuclear density to determine developmental stage. (A, B) cact1 expression is initiated evenly. (C) With proceeding development cact1 expression vanishes from the dorsal side. (D) 20% of Toll1 KD embryos lack cact1 expression. The remainder show reduced expression compared to wt. (E) cact3 expression is initiated uniformly along the DV axis between 20% and 60% egg length. (F, G) In older blastoderm stages cact3 is expressed in a broad domain encompassing 60–80% of the egg circumference. (H) 47% of Toll1 KD embryos lack cact3 expression. The remainder show reduced expression compared to wt. (I, J) Double ISH for cact3 (blue) and sog (red) confirms that cact3 is expressed ventrally and that its domain expands more dorsally than the sog domain.

Temporal progression of pattern formation.
Simulated concentration profiles of NF-κB/Dorsal, Sog and BMP are plotted at successive time points, starting from a broad peak of NF-κB/Dorsal (left to right: t = 0 hr, 4 hr, 6 hr and steady state; see Table 1 for parameter values). The x-axis shows the circumference of the embryo, with the dorsal and ventral sides marked as D and V, respectively.
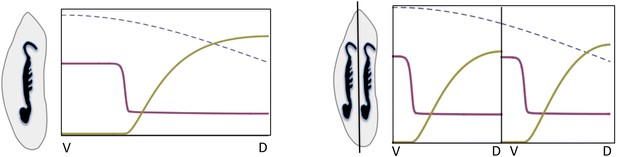
Embryonic twinning in Euscelis.
Simulation of wt (left) showing Sog (red) and BMP (green) protein concentration profiles along the DV axis at steady state given initial NF-κB/Dorsal protein levels (blue: dashed line). A schematic drawing on the left shows a wt Euscelis embryo at the germ band stage. Simulations after the DV axis is split in two halves (right) result in the formation of one BMP gradient in each half. Thus, the proposed model can account for the embryonic twinning observed in Euscelis after production of dorsal and ventral egg fragments shown schematically on the left (Sander, 1971).
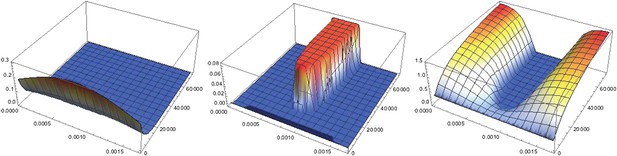
Dynamics of pattern formation.
Each plot shows the concentration of a particular protein species in space (x running from 0 to lx along the front of the plot parameterizing the circumference of the cylinder) and time (running towards the back). Left: the concentration of NF-κB/Dorsal shows a broad Gaussian profile that decays to zero with time. Center: starting from a uniform distribution a region of high Sog concentrations forms where the initial distribution of NF-κB/Dorsal had its maximum. Right: BMP is depleted where Sog levels are high. Initial conditions are b(x, t = 0) = 0.32, s(x, t = 0) = 0.01, c(x, t = 0) = 0.14, d(x, t = 0) = . Throughout the text , except in the twinning figure (Box 1), where was used to ensure a sufficient amount of NF-κB/Dorsal in both halves of the embryo.
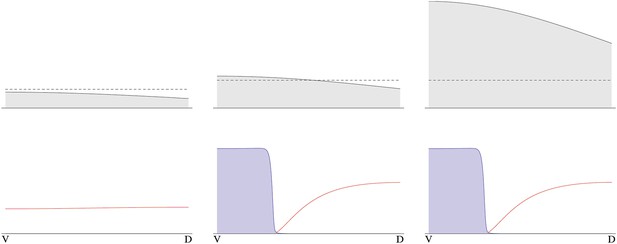
Pattern formation from different initial levels of NF-κB/Dorsal.
The initial concentration gradient of NF-κB/Dorsal is shown on top (gray). Initial amplitudes of NF-κB/Dorsal are from left to right, the dashed line indicates the threshold NF-κB/Dorsal concentration required for patterning. Below, steady-state levels of free BMP (red) and free Sog are shown (blue, rescaled to facilitate plotting on the same plot as BMP).
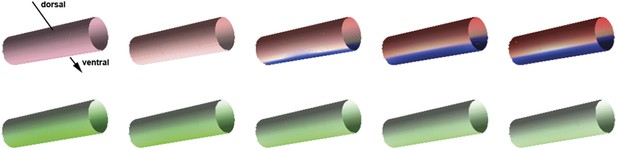
Pattern formation in two dimensions.
Starting from a distribution of NF-κB/Dorsal with a broad maximum running in parallel to the cylinder's axis (bottom, shown in green), a stripe of high Sog concentration develops (top, Sog shown in blue, BMP shown in red). The figures show concentrations at times 0, 1000, 2000, 3000, 4000 from left to right.
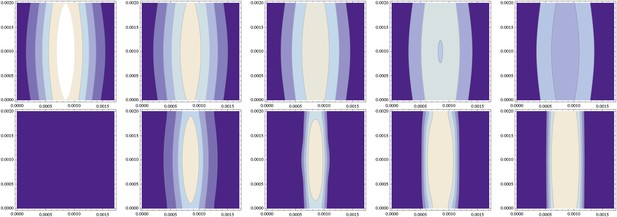
Independence from the stripe of initial conditions (same data as Figure 10).
(top) The initial distribution of NF-κB/Dorsal from Figure 10 varies along the cylinder's axis (y-direction of this contour plot, with the x-direction describing the circumference) in both standard deviation and amplitude by about 10%; , and decays over time (time points 0, 1000, 2000, 3000, 4000 shown from left to right). (bottom) The resulting distribution of Sog (and correspondingly of BMP) becomes uniform along the cylinder axis.
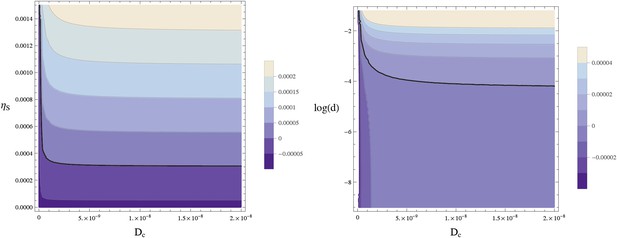
Stability of the homogeneous fixed point.
This contour plot shows the largest eigenvalue w of A − Dk2 for k = 2π/lx. The thick line separates the parameters leading to a stable homogeneous fixed point (w < 0) from an instable homogeneous fixed point (w > 0). (left) w is plotted as a function of the diffusion constant of the Sog-BMP complex and the rate of sog expression at zero BMP, . (right) The same data are plotted against log(d) using . The homogeneous fixed point becomes unstable for sufficiently large values of the diffusion constant of the complex Dc and the concentration of NF-κB/Dorsal d. The remaining parameters are as given in Table 1.
Tables
Model parameters.
η | 2.8 × 10−4 |
η1 | 2 × 10−3 |
b0 | 0.2 |
d0 | 1 |
ηb | 4 × 10−5 |
αs | 2 x 10−3 |
αb | 5 × 10−5 |
αc | 2 × 10−4 |
K+ | 5 |
k− | 5 × 10−5 |
Ds | 1.5 × 10−13 |
Db | 7.8 × 10−13 |
Dc | 2.5 × 10−9 |
lx | 0.0017 |
-
Units are arbitrary but are suggested to be seconds for time and meters for length.
Range of model parameter values where a single stripe is formed
6 × 10−4–1.4 × 10−3 | |
b0 | 0.1–0.5 |
ηb | 8 × 10−6–10−4 |
αs | 1.6 × 10−3–1.2 × 10−2 |
αb | 0–10−4 |
K+ | 0.05–200 |
k− | 0–0.5 |
Ds | 0–10−9 |
Db | 0–10−11 |
Dc | 7 × 10−10–10−6 |
-
Each parameter is varied keeping the other parameters fixed at the values specified in Table 1. One exception is the parameters , which affect pattern formation jointly through the parameter which is set to 1.2 × 10−3 (except in the first line, where this parameter itself is varied).
Additional files
-
Supplementary file 1
PCR primers for production of ISH probes and dsRNA.
- https://doi.org/10.7554/eLife.05502.024