Visualization and functional dissection of coaxial paired SpoIIIE channels across the sporulation septum
Figures
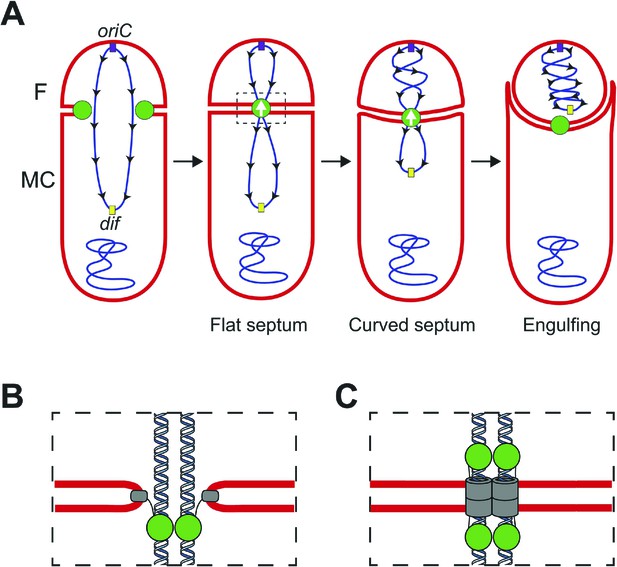
Chromosome translocation during B. subtilis sporulation.
(A) The sporulation septum traps the oriC-proximal region of the forespore chromosome in the forespore (F), the rest in the mother cell (MC). SpoIIIE (green) localizes at the leading edge of the constricting septum, and assembles a translocation complex at the septal midpoint. The SpoIIIE complex maintains separation of the daughter cell membranes in the presence of trapped DNA. The direction of translocation (white arrow) is determined by the orientation-specific interaction between the SpoIIIE γ domain and the skewed chromosomal recognition sequences known as SRS (black arrowheads on the chromosome, indicating the direction that SpoIIIE motor domains move on the DNA). Engulfment commences during DNA translocation, producing a curved septum and movement of the mother cell membrane around the forespore. (B) The aqueous channel model for SpoIIIE, showing two chromosome arms. Green represents the SpoIIIE channels formed by motor domains, grey the transmembrane domains, and red the membrane. (C) The paired channel model for SpoIIIE, in which each chromosome arm passes through a proteinaceous channel with subunits in both cells.
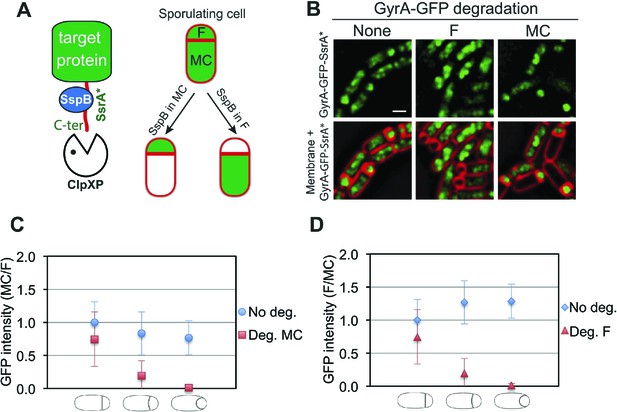
Cell-specific degradation of proteins during sporulation.
(A) Cell-specific protein degradation system (see text). Red indicates the cell membranes, green the target protein. F = forespore, MC = mother cell. (B) Fluorescence microscopy of GyrA-GFP-SsrA* (green) during sporulation, without degradation (strain JLG917), forespore degradation (F, JLG919) and mother cell degradation (MC, JLG1281). Membranes are stained with FM4-64 (red). Scale bar, 1 μm. (C and D) Quantification of the loss of GyrA-GFP-SsrA* fluorescence after degradation in the (C) mother cell and (D) forespore. No degradation controls express GyrA-GFP-SsrA* but not sspBEc (blue circles and diamonds). The ratio of the mean GFP intensity in the (C) mother cell/forespore (red squares) and (D) forespore/mother cell (red triangles) were calculated for sporangia with flat, slightly curved and engulfing septa 2.5 hr after the initiation of sporulation (t2.5). 25–66 sporangia were analyzed for each cell type.
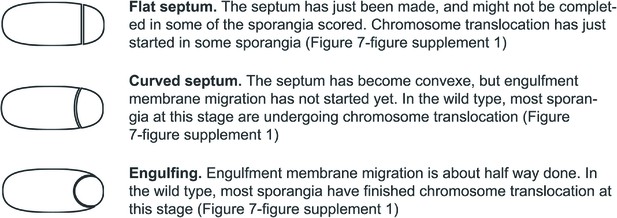
Description of the different stages of engulfment analyzed in this study.
https://doi.org/10.7554/eLife.06474.005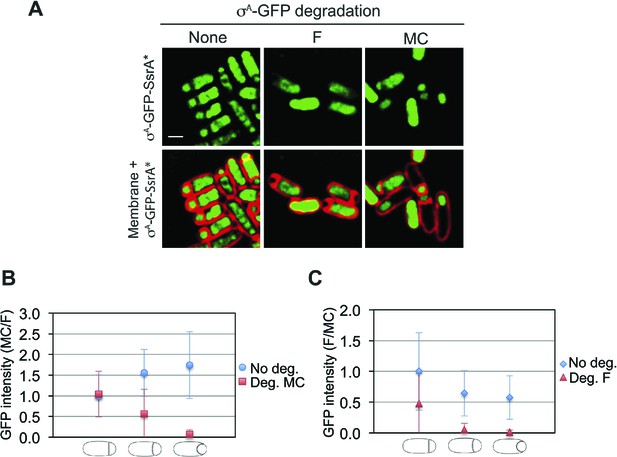
Cell-specific degradation of σA-GFP-SsrA*.
(A) Cell-specific degradation of a σA-GFP-SsrA* fusion protein during sporulation. When sspBEc is not expressed (strain JLG247), GFP signal is detected in both forespore and mother cell. Expressison of sspBEc in the forepore (strain JLG261) leads to GFP disappearance in the forespore, while expression of sspBEc in the mother cell (strain JLG259) leads to GFP disappearance in the mother cell. Sporangia were harvested 2.5 h after resuspension (t2.5) and images of σA-GFP-SsrA* (green) and membrane (red) were taken. Membrane was stained with FM4-64 (red). Scale bar is 1 μm. (B and C) Quantification of the loss of σA-GFP-SsrA* fluorescence after degradation in the (B) mother cell and (C) forespore. No degradation controls express σA-GFP-SsrA* but not sspBEc (blue circles and diamonds). The ratio of the mean GFP intensity in the (B) mother cell/forespore (red squares) and (C) forespore/mother cell (red triangles) cell were calculated for sporangia with flat, slightly curved and engulfing at t2.5. 20–46 sporangia were analyzed for each cell type.
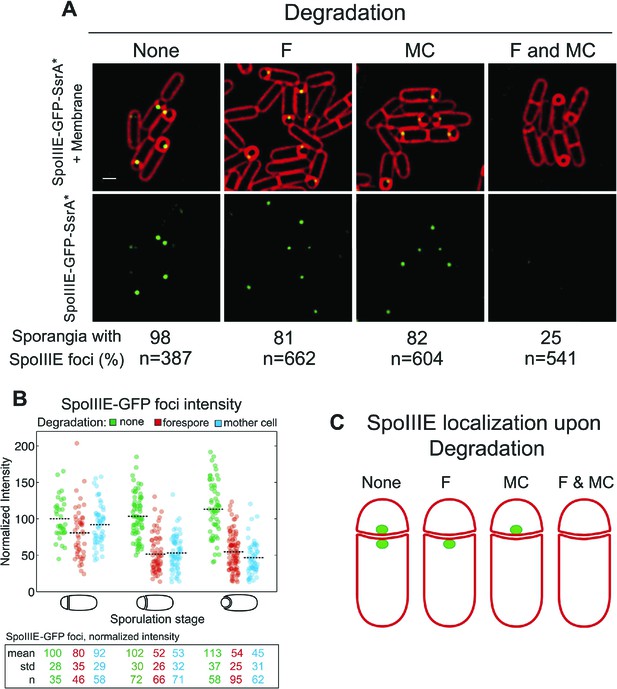
Cell-specific SpoIIIE degradation.
(A) Visualization of SpoIIIE-GFP-SsrA* (green) and FM4-64 stained membranes (red) by deconvolution fluorescence microscopy at t2.5. SpoIIIE was not degraded (None, JLG451) or degraded in the forespore (F, JLG452); mother cell, (MC, JLG453) or both (F and MC, JLG454). Percent sporangia with detectable SpoIIIE foci and the number (n) of scored sporangia are indicated for each strain. Strains containing SpoIIIE-GFP-SsrA* without SspBEc or expressing SspBEc with untagged-SpoIIIE supported wild-type sporulation, chromosome translocation and membrane fission (Supplemental data). Scale bar, 1 μm. (B) Fluorescence intensity of SpoIIIE-GFP foci without degradation (green) or after degradation in the forespore (red) or mother cell (blue) in sporangia with flat, slightly curved and engulfing septa. The mean focus intensity of sporangia with flat septa in the non-degradation strain was normalized to 100. Each dot represents the intensity of a single focus; black dotted lines represent the mean of each data set. Between 35 and 95 foci were analyzed for each data set. (C) Model for SpoIIIE organization at the septal midpoint based on cell-specific degradation. The translocation complex contains SpoIIIE molecules on both sides of the septum so cell-specific degradation will only remove a fraction of the molecules. Degradation in both cells will remove all molecules.
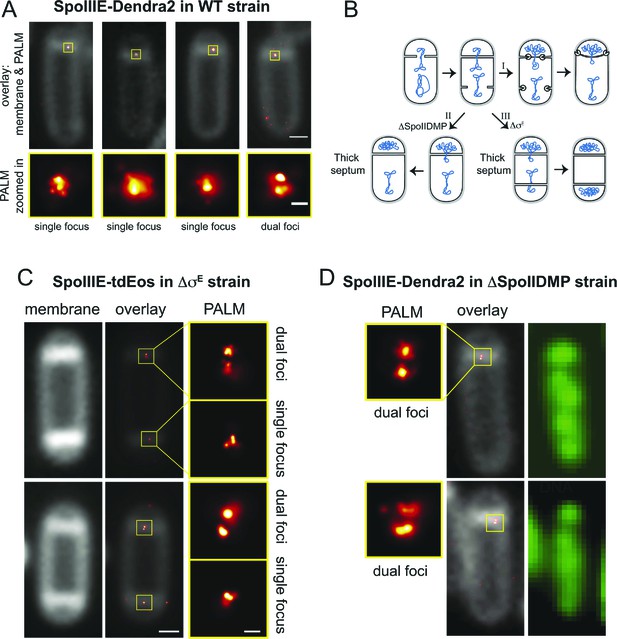
Direct visualization of two SpoIIIE clusters at the septal midpoint.
(A) PALM image of single focus and a rare dual focus of SpoIIIE-Dendra2 in wild type B. subtilis (TCF25), with FM5-95 stained membranes (white). The bottom PALM images are zoomed in from the yellow boxes. Bar is 500 nm and 50 nm for overlaid and PALM images, respectively. Membrane is diffraction-limited image. (B) Schematic diagram of septal thinning. (I) After septation, septal peptidoglycan is degraded by a complex containing the SpoIID, SpoIIM and SpoIIP proteins (pacman) and the second potential division site is blocked. (II) Elimination of SpoIIDMP in the ΔspoIIDMP (spoIID, spoIIM, spoIIP) strain inhibits septal thinning without impairing DNA segregation. (III) Elimination of σE in the ΔσE (spoIIGB) strain inhibits septal thinning and produces disporic cells without impairing DNA segregation. (C) PALM images of SpoIIIE-tdEos in ΔσE strain (JS03). Classification of PALM images as dual foci or single foci were defined according to the parameters of our cluster analysis. Scale bar is 500 nm and 50 nm for overlaid and PALM images, respectively. Membrane is diffraction-limited image. (D) PALM images of SpoIIIE-tdEos in ΔSpoIIDMP strain (JLG571). The diffraction-limited images of the membranes (white) and the DNA (green) were obtained by staining with FM5-95 and DAPI, respectively. The relative forespore DAPI intensity was ∼25% in both sporangia. SpoIIIE dual foci are shown in the left panels. In-gel fluorescence and western blots of the different SpoIIIE fusion proteins used here can be found in Figure 4—figure supplement 7.
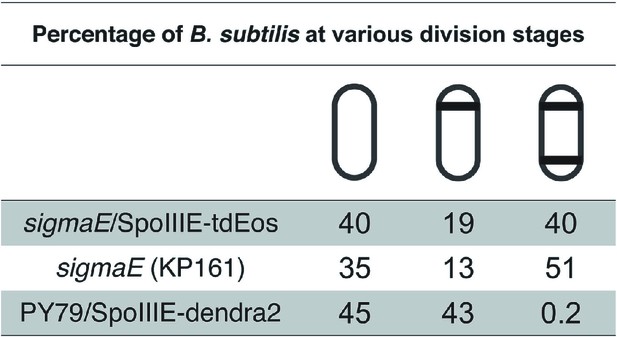
Classification of cells in vegetative, monosporic and disporic cells at t1.75.
Mutation in σE produces a much higher percentage of disporic cells compared to the σE+ strain PY79. More than 700 cells were classified per strain. The strains used were KP161, TCF25 and JS03.
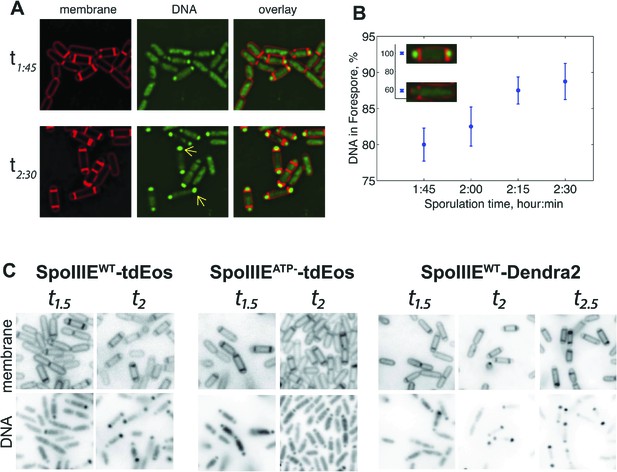
SpoIIIE segregates the chromosomes in the ΔσE strain.
(A) Mutation in σE does not impair DNA translocation. Forespores show brighter DNA signal (single arrowhead) at t2.5 compared to t1.75 of sporulation time showing chromosome translocation from the mother cell into the forespore. ΔσE cells expressing SpoIIIE fused to tdEos (JS03) were stained with DAPI and FM5-95 to visualize DNA (Green) and membrane (red), respectively. DAPI signal was false-colored green for a better contrast. (B) Quantification of DNA translocation at different sporulation times. The amount of DNA in the forespore increases as sporulation progresses (from ∼60–100%, inset). Our PALM experiments using ΔσE strains (Figures 4, 5) were done at t1.75 to maximize the percentage of cells are actively translocating DNA. Error bar represents standard error of the mean from 20 cells for each of the time points. (C) Cells were sporulated at 37°C and samples were taken at indicated times and stained with DAPI and FM5-95 to visualize DNA and membrane (red), respectively. The amount of DAPI (labeled-DNA) in the forespore increased from t1.5 to t2 in ΔσE sporangia expressing wild type SpoIIIE (SpoIIIEWT) fused to tdEos (JYS03), indicating that the forespore received the chromosomal complement from the mother cell. In contrast, ΔσE cells expressing SpoIIIE ATPase mutant (SpoIIIEATP−) fused to tdEos (JYS04) did not translocate DNA. SpoIIIEWT fused to Dendra2 (JYS00) also translocated DNA in ΔσE strain. Altogether, these results indicate that SpoIIIE translocates chromosome during sporulation in ΔσE strain and the fusion protein does not impair the ability of this translocation.
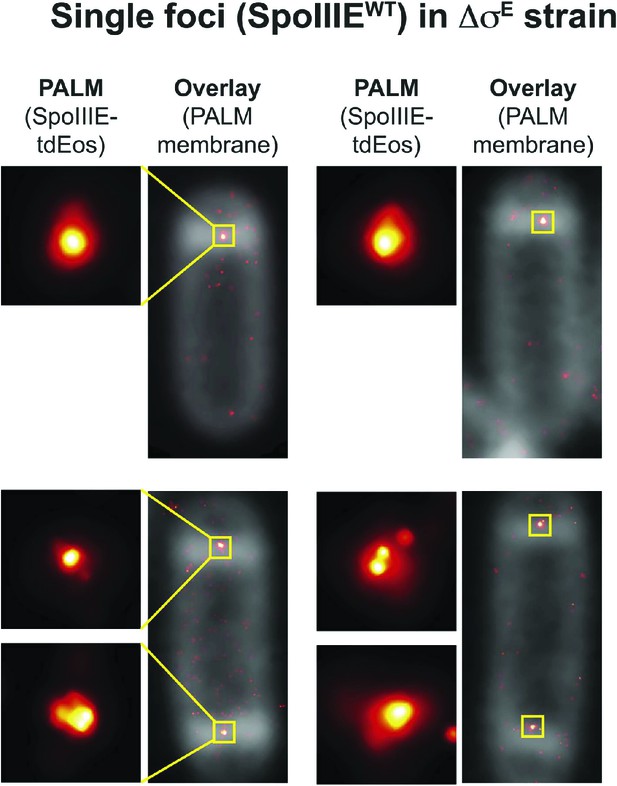
Examples of single foci in the ΔσE strain when SpoIIIEWT is fused to tdEos.
https://doi.org/10.7554/eLife.06474.011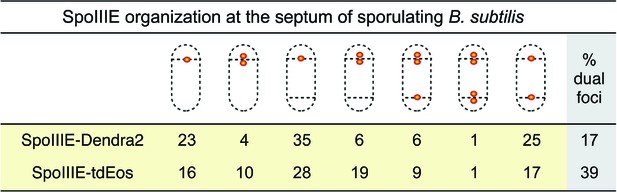
Distribution of single and double foci in monosporic and disporic sporangia.
Classification of monosporic and disporic sporangia in relation to the presence of either single focus or dual foci at the sporulation septum of cells expressing either SpoIIIE fused to tdEos (JS03) or Dendra2 (JS00) in the ΔσE strain.
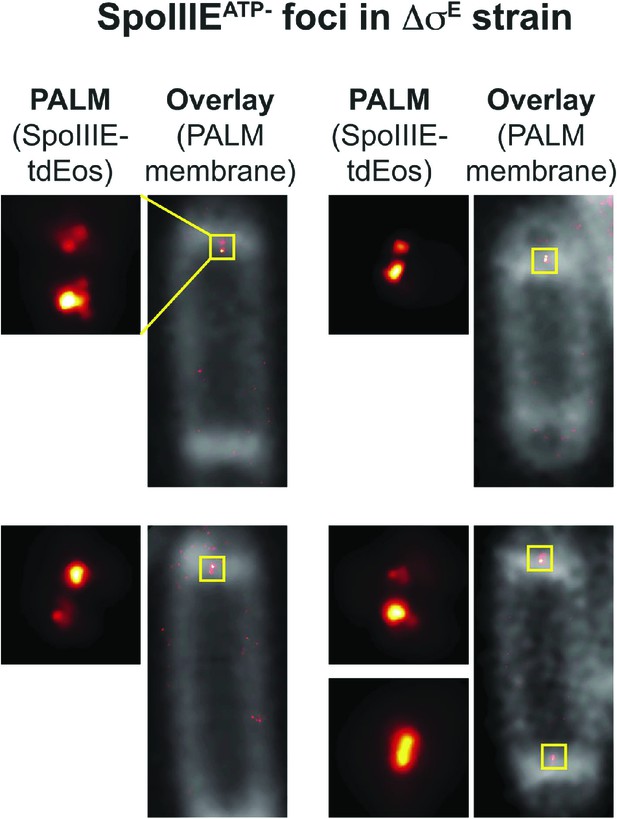
SpoIIIE ATPase mutant (SpoIIIEATP−) fused to tdEos in ΔσE strain also organizes into dual foci.
To demonstrate that SpoIIIEWT dual foci (Figure 4) are observed when the chromosome is trapped in the septum and discard that are only at septa where chromosome transport is complete we imaged SpoIIIEATP− fused to tdEos by PALM. Single mutation G467S in the conserved region of the SpoIIIE ATPase motor domain impairs DNA translocation without affecting SpoIIIE foci assembly, and the forespore chromosome remains trapped in the septum (Sharp and Pogliano, 1999; Fleming et al., 2010). qPALM shows that SpoIIIEATP− also assembles into dual foci (PALM: left panels and, first and second right panels) and single foci (third right panel) similar to the SpoIIIEWT (Figure 4). As expected sporangia expressing SpoIIIEATP− did not translocate DNA (Figure 4—figure supplement 2C).
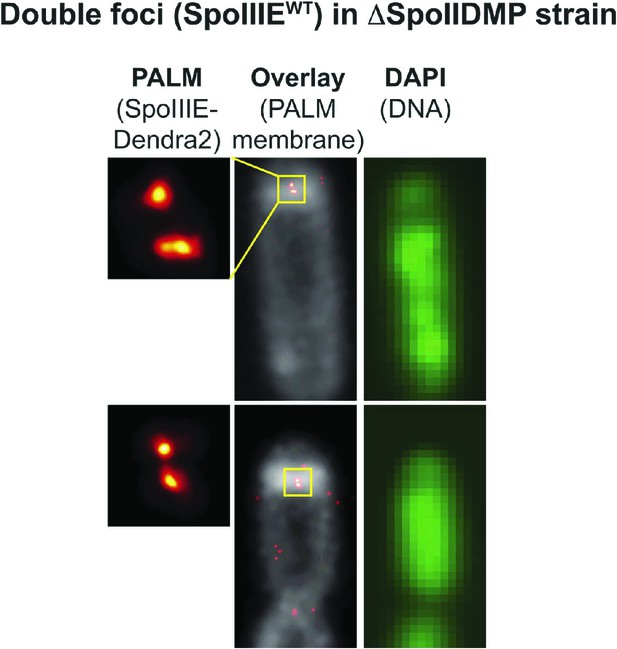
SpoIIIE organizes into dual foci at the septum of sporangia where chromosome transport is incomplete.
To demonstrate that dual foci represent SpoIIIE complexes that are actively translocating DNA, we imaged SpoIIIEWT in a different Bacillus strain, spoIIDMP triple mutant strain, which mantains a thick sporulation septum due to the inhibition of septal thinning (Pogliano et al., 1999; Abanes De Mello et al., 2002; Chastanet and Losick, 2007; Gutierrez et al., 2010). In contrast to the ΔσE mutant, the completion of the second polar septum is inhibited in the spoIIDMP triple mutant, leading to the formation of fewer disporic sporangia. This feature allows the estimation of the degree of chromosome translocation using DNA-specific dyes and measuring the dye-intensity in the forespore vs the total intensity (forespore plus mother cell), a standard procedure in the field (Becker and Pogliano, 2007; Ptacin et al., 2008). The relative forespore DAPI intensity of sporangia showing dual foci in the spoIIDMP mutant ranged between 0.1 and 0.32, suggesting that they were actively translocating DNA. These observations suggest that dual foci represent the organization of the SpoIIIE complex during the process of DNA translocation. Criteria for complete and incomplete DNA translocation is described in the chromosome translocation section of the results and in Figure 7—figure supplement 1.
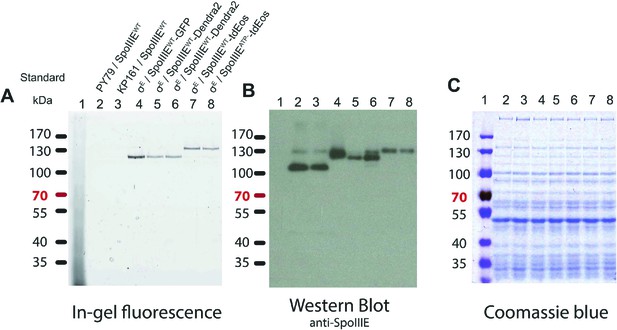
In-gel fluorescence and western blot of the SpoIIIE fusion proteins used in this study.
Our single SpoIIIE molecule quantification relies on the fluorescent protein fused to SpoIIIE. Therefore, it is crucial that all SpoIIIE molecules are fused to the fluorescent protein and also all the fluorescent proteins fused to SpoIIIE. (A) Fluorescent proteins (GFP, Dendra2 and tdEos) fused to SpoIIIE migrate as a single band in 7% semi-denaturing in-gel fluorescence PAGE (Drew et al., 2006). As expected the tandem dimer Eos (tdEos) fusion proteins migrate slightly higher than the GFP and the Dendra2 fusion proteins. (B) SpoIIIE fused to fluorescent proteins migrates as a higher molecular weight compared to the native SpoIIIE (lanes 2 and 3), indicating that all the SpoIIIE molecules are fused to the fluorescent proteins. The molecular weight of the fusion proteins corresponded to the in-gel fluorescence. The faint band at ∼130 kDa is unspecific. (C) Total protein visualized by Coomassie-Blue staining.
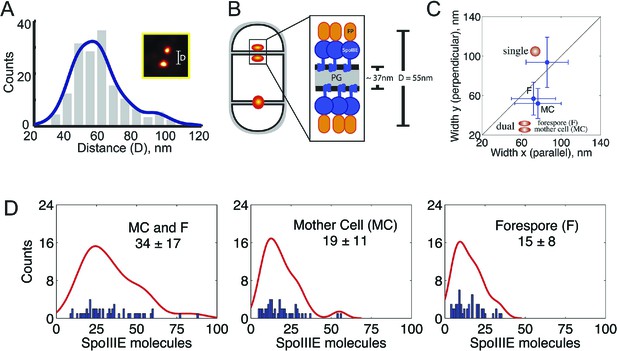
quantitative PALM (qPALM) of SpoIIIE complexes.
(A) The distances (D) between the centers of each cluster from SpoIIIE-tdEos dual foci in ΔσE strain (JYS03). The average distance between clusters, indicated by the main peak value of the kernel density estimator (blue line), is 55 nm. (B) Schematic diagram of SpoIIIE (blue) at the division septum in ΔσE strain. To estimate the thickness of the peptidoglycan (PG) we subtracted twice the width of the lipid bilayer (3 nm; Lewis and Engelman, 1983) and twice the height of SpoIIIE (6 nm), based on the FtsK crystal structure (Massey et al., 2006) from 55 nm, to give 37 nm. FP, fluorescent protein. (C) Dimensions of single SpoIIIE-Dendra2 foci and resolved clusters in dual foci. Focus widths in foci parallel (x) and perpendicular (y) to the septum were calculated as described in supplementary methods. For single foci the parallel- and perpendicular-width (in nm) are 86 ± 10 and 94 ± 12 respectively, for dual foci, the forespore proximal cluster is 64 ± 11 and 57 ± 8, the mother cell proximal cluster 77 ± 12 and 52 ± 7. Error bars, standard deviation from Nsingle = 221, Ndual foci = 51. (D) Distribution of the number of SpoIIIE-Dendra2 molecules determined by qPALM at the septum in the mother cell, in the forespore and in both. Data (blue bars) are represented by Kernel Density Estimator (red solid lines). Mean and standard deviations from Nfoci = 51 are shown. More details about qPALM and the algorithm employed for quantification can be found in ‘Materials and methods’.
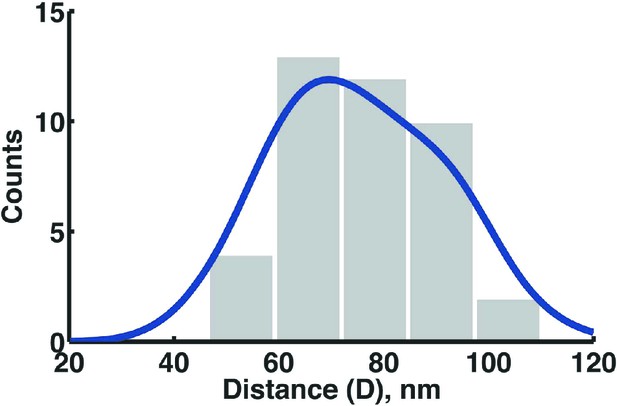
Distance distribution between the centers of each of the clusters from the SpoIIIE-Dendra2 dual foci in ΔσE strain.
The main peak value of the kernel density estimator (blue line) is 68 nm, which is slightly higher than the measurement with SpoIIIE-tdEos strain (55 nm, Figure 5A), most likely because Dendra2 is dimmer and thus its localization is less accurate than mEos2.
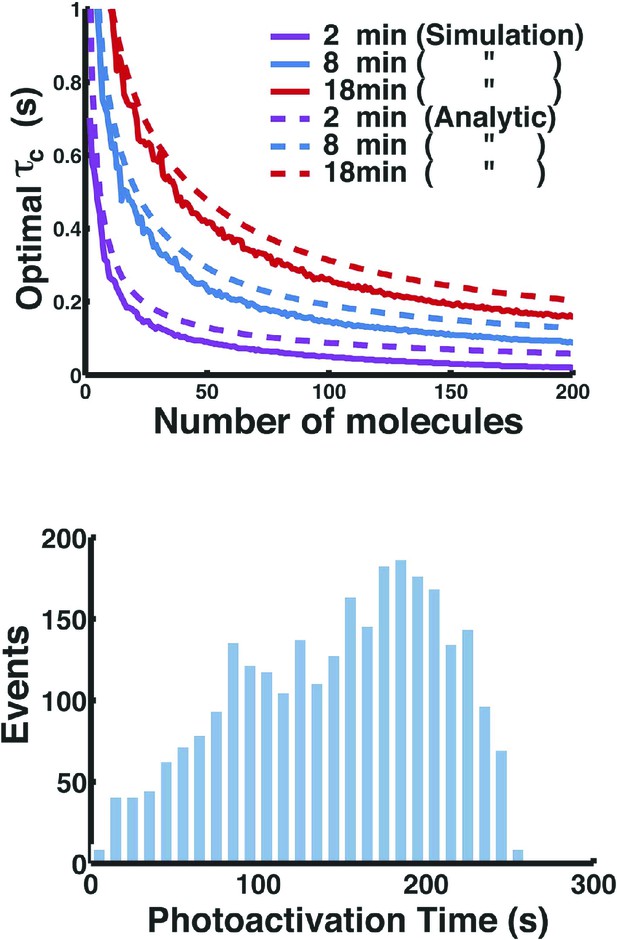
Fermi-photoactivation.
(Top) Approximate analytic solution of the optimal τc agrees well with the exact solution obtained from full stochastic simulations. The experimental time tF and the number of molecules Nmol were varied, whereas the kinetic rates were fixed to the rates of Dendra2 obtained from in vitro single-molecule experiments (Lee et al., 2012). (Bottom) Photoactivation time of Dendra2 fused to SpoIIIE using Fermi-photoactivation scheme corresponding to the Figure 5D. Details about the quantification of the number of molecules using PALM can be found in ‘Materials and methods’.
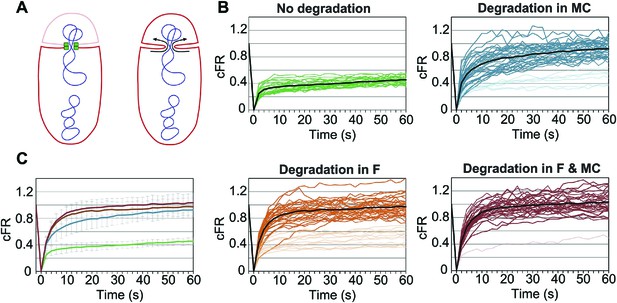
Role of forespore and mother cell SpoIIIE in membrane fission.
(A) Membrane organization in wild type (left) and ΔSpoIIIE (right) strains. SpoIIIE (green) blocks the FM4-64 (red) diffusion from the mother cell to the forespore, which remains bleached (pale red forespore). Arrows show diffusion of FM4-64 to the forespore. (B) Corrected fluorescence recovery (cFR) of individual sporangia without degradation (JLG808) or after degradation in the forespore (F, JLG821), mother cell (MC, JLG823) or both (F and MC, JLG825). When calculating the average cFR (black lines) we excluded curves (pale lines) that resembled those without degradation because they likely represent sporangia in which SpoIIIE is not yet degraded. (C) Average cFR in sporangia without SpoIIIE degradation (green, n = 27) or degradation in the mother cell (blue, n = 35), the forespore (orange, n = 33) or both (red, n = 33). Error bars, standard deviation.
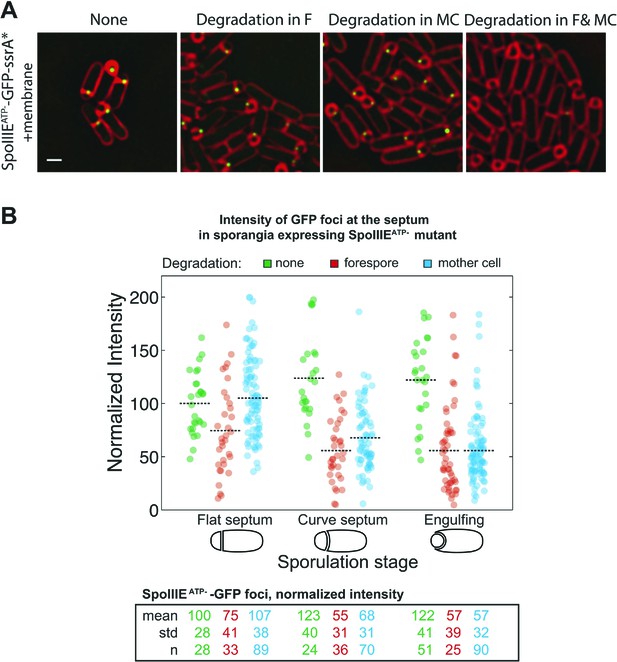
Cell-specific degradation of SpoIIIEATP− mutant.
(A) Visualization of SpoIIIEATP−-GFP-SsrA* (green) by deconvolution fluorescence microscopy. SpoIIIE was not degraded (strain JLG808) or selectively degraded in the mother cell (strain JLG823), in the forespore (strain JLG821) or in both (strain JLG825). The SpoIIIE foci disappear when SpoIIIE is degraded simultaneously in forespore and mother cell, but not when degraded only in one cell. Membranes were stained with FM4-64 (red). Scale bar is 1 μm. (B) Normalized fluorescence intensity of SpoIIIEATP−-GFP-SsrA* foci without degradation (green; JLG808), or after degradation in the forespore (red; JLG821) or mother cell (blue; JLG823). Foci intensities were measured in sporangia with flat septum, curved septum and engulfing. Each dot represents the focus intensity from a single sporangium, and black dotted lines represent the mean. The mean foci intensity of sporangia with flat septa in the non-degradation strain was normalized to 100. Similarly to wild type SpoIIIE (Figure 3B), the foci intensity decreases to approximately half when SpoIIIEATP−-GFP is degraded either in the forespore shortly after polar septation (curve septa). The mean foci intensities, standard deviations, and number (n) of analyzed sporangia are indicated in the table below the graph.
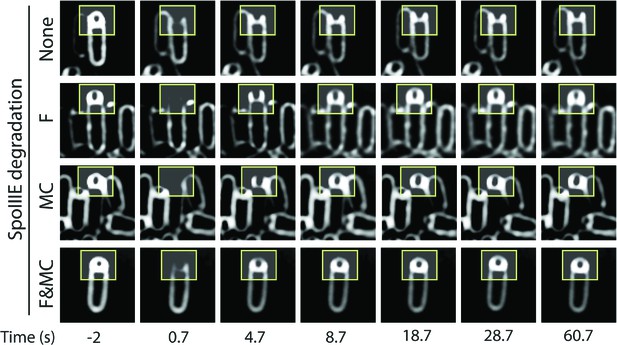
Fluorescence recovery after photobleaching (FRAP) of forespore membranes.
Images show the FRAP the forespore membranes of representative sporangia when SpoIIIEATP− is not degraded (strain JLG808) or selectively degraded in the forespore (F; strain JLG821), in the mother cell (MC; strain JLG823), or degraded in both (MC and F; strain JLG825). The first column (−2 s) is before photobleaching the forespore area (indicated by yellow box). Snapshots after photobleaching were taken at the indicated times.
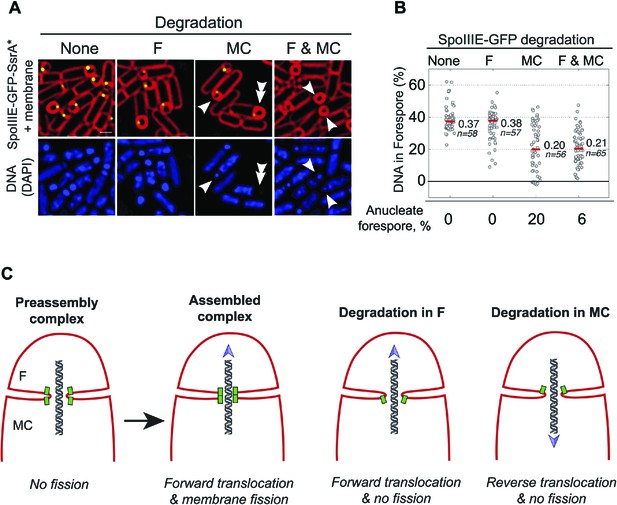
Role of forespore and mother cell SpoIIIE in chromssome translocation.
(A) Visualization of DAPI-stained DNA (blue) after cell-specific degradation of SpoIIIE-GFP-SsrA* (green). Membranes are stained with FM4-64 (red). Single arrowheads show forespores containing small amounts of DNA; double arrowhead shows anucleate forespores. Scale bar, 1 μm. (B) Forespore DAPI intensity normalized to the total intensity (forespore plus mother cell) of sporangia about to complete engulfment in the indicated degradation strains. Each dot shows the normalized DAPI intensity of one forespore. Median (red line) and the number of sporangia (n) are indicated. Sporangia containing <0.05 DNA in the forespore were considered anucleate. (C) Models for SpoIIIE and membrane organization before and after cell-specific SpoIIIE degradation. During division, SpoIIIE assembles channels that separate daughter cell membranes and mediate forward chromosome translocation. Selective degradation of SpoIIIE either in the mother cell or the forespore reverses membrane fission, with the remaining molecules exporting DNA from their respective cell.
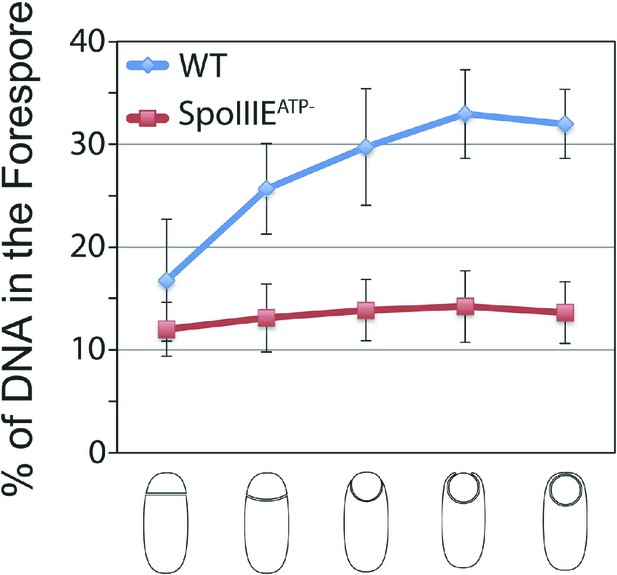
Chromosome translocation at different stages of engulfment.
The graph shows the normalized forespore DAPI intensity of wild type (PY79) and SpoIIIEATP− mutant strain (KP541) for sporangia at different stages of engulfment. Normalized forespore DAPI intensity was calculated as [Forespore DAPI intensity/(Foresore DAPI intensity + Mother cell DAPI intensity)], for details see ‘Meterials and methods’. As engulfment progresses, the normalized DAPI intensity of the wild type sporangia increases gradually until it reaches a plateau in cells undergoing engulfment. SpoIIIE degradation in either mother cell or forespore happens before engulfment starts (Figure 3B), and therefore before chromosome translocation is completed. As expected, the DAPI intensity does not increase in the translocation-defective SpoIIIEATP− mutant sporangia. The average and standard deviation of at least 25 different sporangia is represented for every engulfment stage.
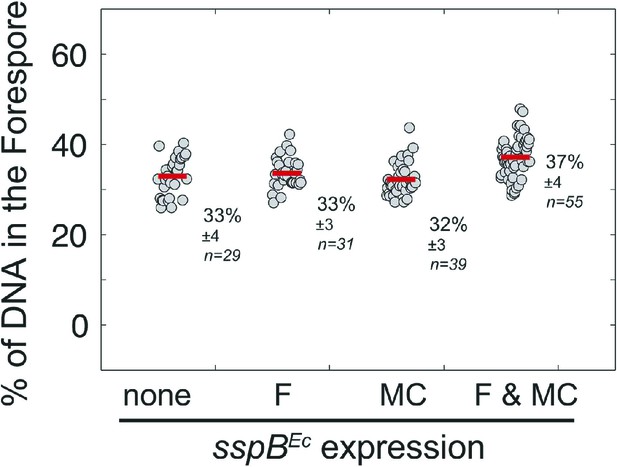
Effect of sspBEc expression on chromosome translocation.
The normalized forespore DAPI intensity was calculated for sporangia about to complete engulfment, in strains not expressing sspBEc (PY79) or expressing sspBEc in the forespore (JLG170), mother cell (JLG180), or both (JLG323). SpoIIIE is not tagged with SsrA* in those strains and therefore no degradation is expected. In these strains DNA translocation is not impaired. Each dot represents the normalized DAPI intensity of a sporangium. Median (red line) and the number of sporangia (n) are indicated.
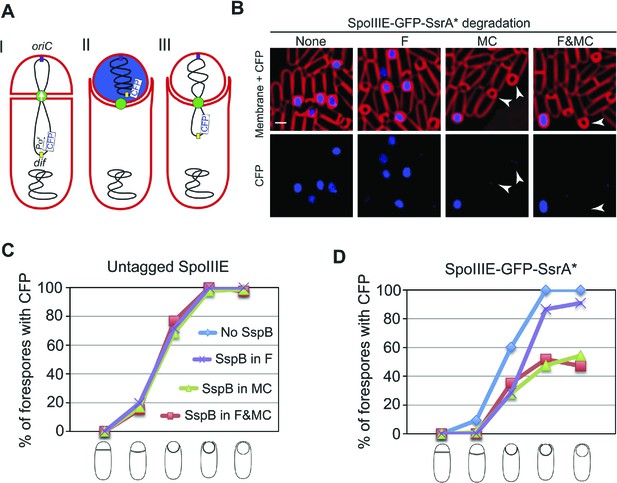
Alternative approach to measure chromosome translocation upon cell-specific degradation of SpoIIIE.
(A) We inserted a gene encoding CFP under the control of a σF-dependent promoter (PspoIIQ) in the cotC locus of Bacillus subtilis chromosome (I). cotC (168°) is close to the dif site (166°) and therefore is one of the last regions of the chromosome to be translocated into the forespore. Since the CFP gene is expressed from a σF-dependent promoter, it will not be transcribed until it reaches the forespore. Therefore, we can use the presence of CFP in the forespore as an indicator that chromosome translocation has been completed (II). If chromosome translocation is impaired and the cfp gene does not reach the forespore, no CFP signal will be detected (III). (B) Deconvolution fluorescence microscopy of sporangia containing cfp under the control of spoIIQ promoter inserted in the cotC locus, in strains in which SpoIIIE is not degraded (JLG981), degraded in the forespore (JLG1001), degraded in the mother cell (JLG1002) or degraded in both (JLG1003). When SpoIIIE is not degraded or degraded only in the forespore most sporangia show CFP signal in the forespore, indicating that translocation has been completed. However, when SpoIIIE is degraded in the mother cell or in both the forespore and mother cell simultaneously, many sporangia in late stages of engulfment do not show CFP signal in the forespore (arrowheads), indicating that chromosome translocation is not completed. Cells were imaged at t2.5. Scale bar is 1 μm. (C) Percentage of forespores showing CFP signal in strains containing SsrA* untagged SpoIIIE for sporangia in different stages of engulfment. The adaptor protein SspBEc was not expressed (EBS606) or expressed in the forespore (JLG978), mother cell (JLG979), or both (JLG980). Contrary to the SsrA* tagged SpoIIIE strains (from Firugre S6D and F) all sporangia about to complete engulfment show GFP signal in the forespore, indicating that chromosome translocation is already completed at this stage. Expression of sspBEc does not affect the rate at which CFP signal appears in the forespore. Between 25 and 216 sporangia were analyzed, depending on the strain and cell type. (D) Percentage of sporangia in different stages of engulfment showing CFP signal in the forespore when SpoIIIE is not degraded (JLG981) or degraded in the forespore (JLG1001), mother cell (JLG1002) or both (JLG1003). Degradation of SpoIIIE in the mother cell or in both the mother cell and forespore simultaneously reduces the percentage of sporangia showing CFP signal in late stages of engulfment by ∼50%. Degradation of SpoIIIE in the forespore produces a delay in the appearance of CFP signal compared to the control, but >90% of the forespores are blue by the completion of engulfment. Between 32 and 306 sporangia were analyzed, depending on the strain and cell type.
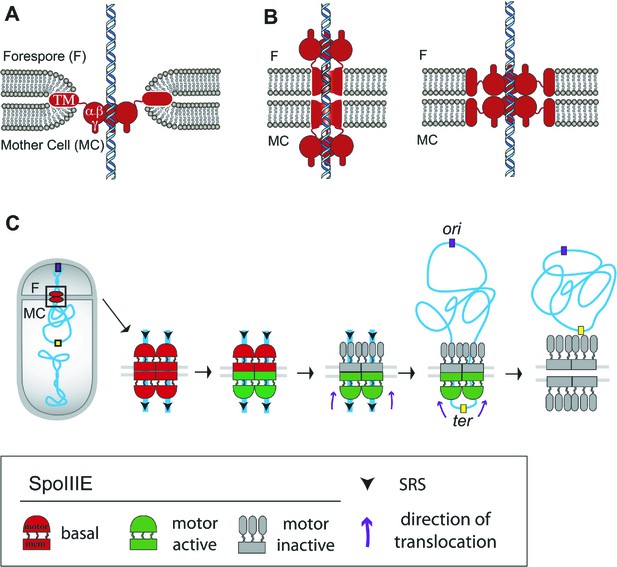
Architecture of the SpoIIIE complex during DNA translocation.
(A and B) Septal cross sections showing SpoIIIE (red), DNA (blue) and lipids (grey) and displaying just one DNA strand traversing the septum for simplicity, although at least two arms of the circular chromosome must cross the septum. Our results indicate that the translocation channel has approximately equal numbers of SpoIIIE motor domains in each cell, consistent with the models shown in B, but not with the simple aqueous channel model in A. They further indicate that the SpoIIIE membrane domains are arranged in such a way that they form a continuous protein barrier at the septum that blocks diffusion within the lipid bilayer and that molecules in each cell are required to form this barrier. We envision two alternative organizations to achieve this. First, the transmembrane domains might form channels in each septal membrane that interact within the septal space and conduct the DNA across the septum, with the motor domains projecting into the cytoplasm (left). Second, the transmembrane domains might form a contiguous external ring that blocks lipid diffusion, with motor domains projecting towards the lumen of an aqueous channel (right). The distance between the centers of the forespore and mother cell clusters (55 nm) is larger than the thickness of the septum (40 nm, Tocheva et al., 2013) leading us to prefer the paired channel model (left). (C) Each chromosome arm is translocated by a different SpoIIIE channel that is comprised of opposing motor domains that have the ability to export DNA from their respective cell. Translocation directionality is established by the interaction between SRS and the SpoIIIE γ domain, which activates the mother cell motor (green), and inactivates the forespore motor (grey). Consequently, the mother cell-SpoIIIE exports DNA until the final loop (ter) reaches the septum. We propose that translocation of the loop generates tension that destabilizes and opens the channels forming a larger pore to pass the loop.
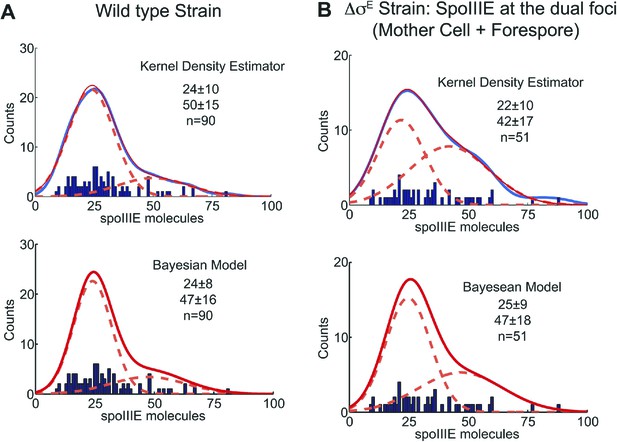
Statistical analysis of qPALM data.
A detailed analysis of qPALM data reveals the existence of two populations of sporangia with different numbers of SpoIIIE monomers at the septum. (A and B) Comparison between two different analyses—Gaussian Kernel Density Estimator (GKDE) and Variational Bayesian Gaussian Mixture Model (VBGMM)—for quantification of SpoIIIE in (A) the wild type strain and (B) the ΔσE strain. Both analyses lead to the finding of two populations with the peak values ∼24 and 50 (red dotted lines). (Top panels from A and B) GKDE (blue curves) of the SpoIIIE counting data (blue bars) were fitted well by a mixture of two Gaussian functions (red solid curves). See ‘Materials and methods’ for details on the analyses.
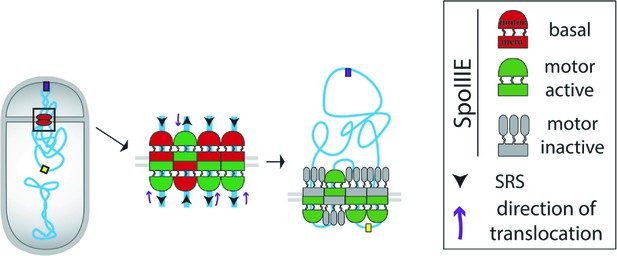
Model for chromosome translocation if four DNA strands cross the septum.
Septum containing four arms of DNA still can translocate the entire chromosome toward the forepore. The orientation of SRSs along the chromosme and their interaction with SpoIIIE is such that three arms are translocated toward the forespore by the mother cell-SpoIIIE while one arm is translocated into the mother cell by the forespore-SpoIIIE. Therefore, the net translocation is toward the forespore.
Tables
Summary of SpoIIIE quantification obtained by qPALM and diffraction-limited images in the wild type strain, cell-specific degradation system and the thick septum strain (ΔσE)
SpoIIIE-dendra2 quantification by qPALM, absolute numbers | SpoIIIE-GFP intensity, % | |||||||
---|---|---|---|---|---|---|---|---|
Wild type | ΔσE (dual foci) | Cell-specific degradation | ||||||
Strain | SpoIIIEWT | SpoIIIEATP− | MC + F | MC | F | None | F | MC |
Mean (SD) | 31 (18) | 31 (14) | 34 (17) | 19 (11) | 15 (8) | 100 (29) | 51 (25) | 52 (31) |
N | 91 | 128 | 51 | 51 | 51 | 72 | 66 | 123 |
Additional files
-
Supplementary file 1
Strains and plasmids.
(A) Strains used in this study. (B) Plasmid used in this study. (C) Oligonucleotides used in this sudy. (D) Sequence of amino acid residues of the linkers within the SpoIIIE-GFP-SsrA*. (E) Spore titers of strains containing the different spoIIIE fusion proteins used in this study.
- https://doi.org/10.7554/eLife.06474.030
-
Source code 1
qPALM analysis MATLAB package.
- https://doi.org/10.7554/eLife.06474.031