Interneurons and oligodendrocyte progenitors form a structured synaptic network in the developing neocortex
Figures
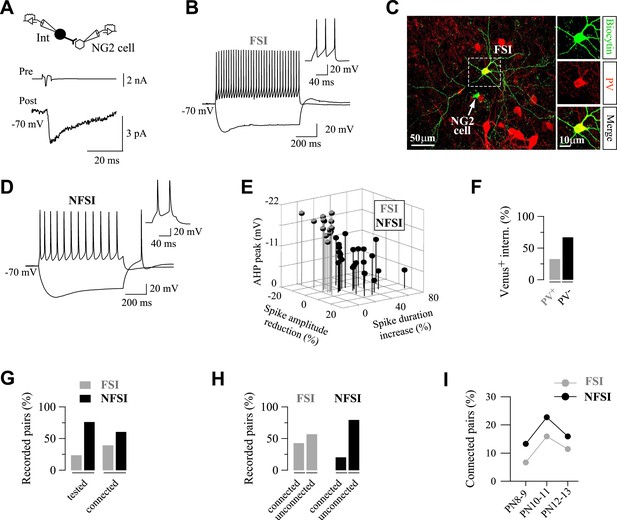
FSIs are highly connected to NG2 cells.
(A) Paired recording between a presynaptic fast-spiking interneuron (FSI) and a NG2 cell. An action current evoked in the presynaptic interneuron (upper trace) elicits PSCs recorded in the NG2 cell (bottom trace; average of 200 traces). (B, D) Current-clamp recordings of the FSI recorded in A (B), and a non-fast-spiking interneurons (NFSI) (D) connected to a NG2 cell during injections of −150 pA and 200 pA. Note differences on spike properties between the two cells (insets, right). (C) The connected FSI was loaded with biocytin and was immunoreactive for PV (stacks of 17 Z-sections; each 2 µm). (E) 3D plot of the three major electrophysiological parameters distinguishing connected FSIs (gray) from NFSIs (black). (F) Histograms showing the fraction of Venus+ interneurons that are PV+ (n = 6 mice). (G) Percentages of tested and connected FSI (gray) and NFSI (black) with respect to all tested interneurons. (H) Percentages of connected and unconnected FSIs (gray) and NFSIs (black) with respect to each group of interneurons separately. (I) Connection probability of FSI and NFSI as a function of three postnatal stages (45, 44, and 44 tested pairs at PN8-9, PN10-11, and P12-13, respectively).
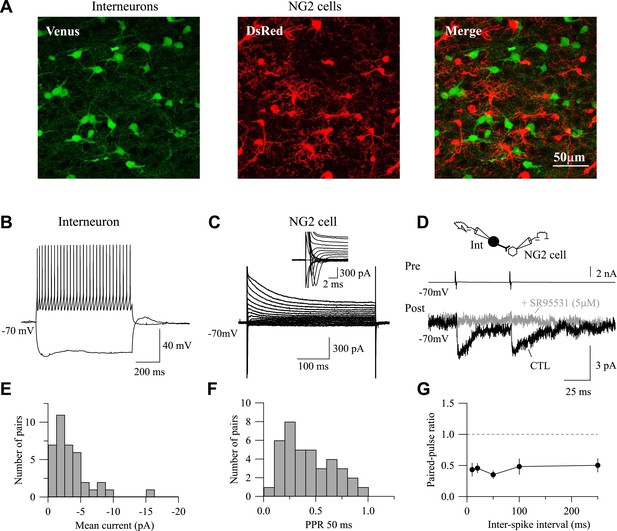
Paired recordings in double VGAT-Venus;NG2-DsRed transgenic mouse.
(A) Confocal images of the VGAT-Venus;NG2-DsRed transgenic mouse in which interneurons and NG2 cells were identified by the expression of the fluorescent proteins Venus (green) and DsRed (red), respectively (stacks of 15 Z-sections; each 0.5 µm). (B–D) Paired recording between a presynaptic Venus+ interneuron and a DsRed+ NG2 cell. Current-clamp recording of the interneuron recorded with a KGlu-based intracellular solution during injections of −150 pA and 200 pA (B) and voltage-clamp recording of the NG2 cell held at −70 mV during voltage steps from +40 mV to −120 mV (C). Note the presence of INa+ (C, inset). Two action currents evoked in presynaptic interneurons elicited PSCs recorded in NG2 cells (D, black trace; average of 100 traces) that are completely abolished by the GABAA receptor antagonist SR95531 (5 µM; gray trace; average of 100 traces). (E, F) Distribution of mean current amplitudes (E) and paired-pulse ratios (F, PPR) for 38 connected pairs. (G) Interneuron-NG2 cell connections do not show any recovery from depression within at least 250-ms interstimulus interval (n = 5–10 per interstimulus interval).
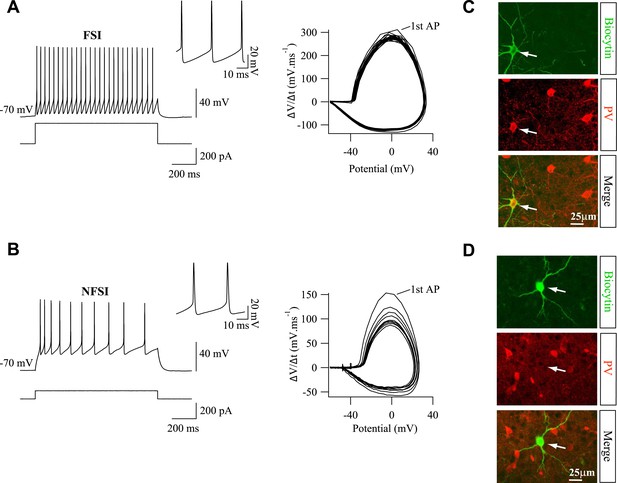
PV marker is expressed in electrophysiologically identified FSIs, but not in NFSIs.
(A, B) Current-clamp recordings of a FSI (A) and a NFSI (B) during current injections (left). Note the differences on spike properties between the two cells (insets, right). Phase plots (ΔV/Δt vs potential; right) illustrate differences on spiking pattern for the same discharge (Daw et al., 2007). During a train discharge, action potentials are relatively homogeneous for FSIs, but they change drastically for NFSIs as revealed by the variance of successive loops in the phase plot. (C, D) PV immunostaining of biocytin-loaded FSI (C; n = 12) and NFSI (D; n = 7). Note the lack of PV labeling for NFSI (stacks of 27 and 17 Z sections, respectively, each 0.5 µm).
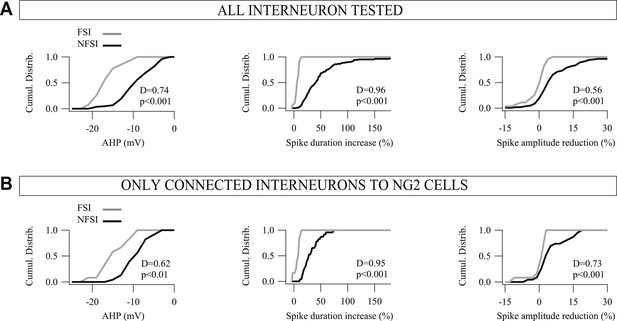
Cumulative distributions for the three main electrophysiological parameters used to differentiate FSIs from NFSIs.
(A, B). Comparison of cumulative distributions for AHP (left), spike duration increase (middle), and spike-amplitude reduction (right) between FSIs (gray) and NFSIs (black) for 132 interneurons (A) and for 38 connected interneurons to NG2 cells (B). Note the restricted distributions of these parameters for FSIs compared to NFSIs for both tested and connected neurons. No significant differences were observed between tested and connected FSIs as well as between tested and connected NFSIs (p > 0.05). Altogether, these results support the idea that FSIs constitute a homogeneous population, whereas NFSIs, connected or not to NG2 cells, form a heterogeneous group of cells that comprises different subtypes of interneurons.
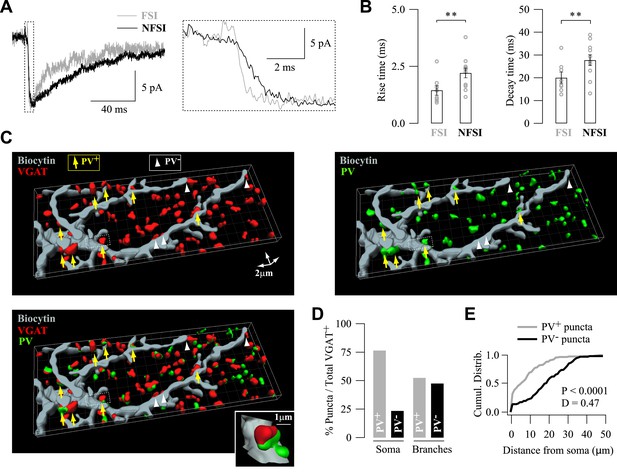
Specific subcellular distribution of FSI and NFSI synaptic contacts and postsynaptic GABAARs on NG2 cells.
(A) Different kinetics of unitary postsynaptic NG2 cell currents depends on the identity of the presynaptic interneuron. Superimposed postsynaptic currents evoked in a FSI-NG2 cell connection (gray) and a NFSI-NG2 cell connection (black). Note the faster rise (inset, right) and decay times for the FSI-NG2 cell connection (t10–90 = 0.91 ms and τ = 12.7 ms for the FSI-NG2 cell connection and 2.25 ms and 23.2 ms for the NFSI-NG2 cell connection). Only traces showing a response in the first presynaptic action current were averaged. (B) Histogram comparing rise (left) and decay times (right) for different FSI-NG2 cell and NFSI-NG2 cell connections. (C) 3D reconstruction of a biocytin-loaded NG2 cell (gray), VGAT (red), and PV (green) labeling at PN10 (see original immunostainings in Figure 2—figure supplement 2A). VGAT+/PV+ puncta were localized at proximal branches and soma (yellow arrows), whereas VGAT+/PV− puncta were mainly localized at distal branches (white arrowheads). Only soma and two main branches of the NG2 cell are shown. Inset illustrates a VGAT+/PV+ puncta. (D) Percentage of PV+ and PV− puncta in somata and branches of NG2 cells (n = 4 cells; 6–8 branches per cell). (E) Cumulative distributions of PV+ and PV− puncta in respect to their distance from the soma. Note the restricted distribution for PV+ puncta. **p < 0.01.
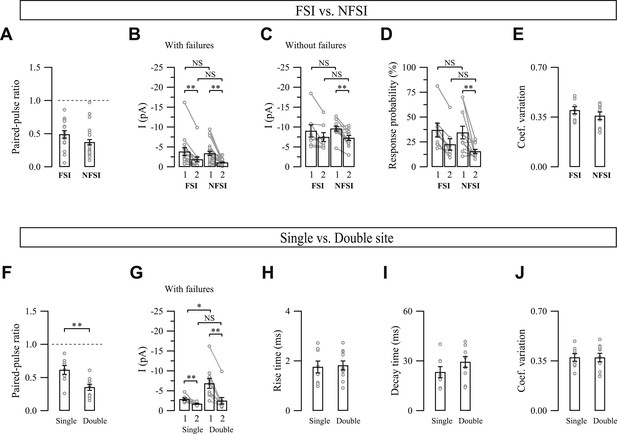
Properties of unitary synaptic interneuron-NG2 cell connections.
(A–E) Comparison of synaptic properties between FSI-NG2 cell and NFSI-NG2 cell connections. Note that no differences exist between these two types of connections though some differences exist when comparing PSC1 (1) and PSC2 (2) within each type of connection. (F–J) Comparison of synaptic properties between connections showing single- and double-vesicular release sites. Note that the PPR (F) and mean amplitudes with failures (G) of PSC1 (1) and PSC2 (2) were different between these two types of connections, whereas rise (H) and decay (I) times and the coefficient of variation (J) were similar. NS: not significant; *p < 0.05, **p < 0.001. For readability, NS tests were indicated only in panels B–D and G.
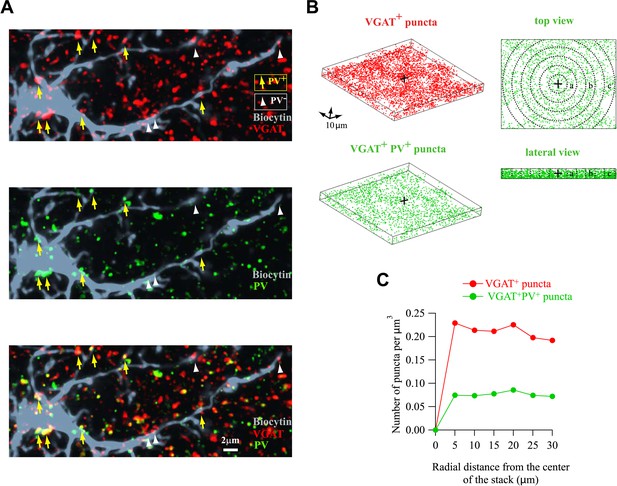
Homogeneous distribution of VGAT+/PV+ puncta around NG2 cells.
(A) Original immunostainings against VGAT (red) and PV (green) around the biocytin-labeled NG2 cell (gray) shown in Figure 2C. Note the accurate match of colocalized puncta between these images and 3D reconstructions in Figure 2C. (B) Distribution of all VGAT+ (red) and VGAT+/PV+ (green) puncta around a biocytin-labeled NG2 cell (left). Top and lateral views illustrate how the density of puncta was calculated in defined volumes from the center of the field (right, dashed lines; see ‘Materials and methods’). (C) Distribution of the density of all VGAT+ (red) and VGAT+/PV+ (green) puncta for each volume in respect to the center of the field. Note the homogeneous distribution of both all VGAT+ and VGAT+/PV+ puncta, confirming that FSI inputs are not unequally located around the soma of the NG2 cell.
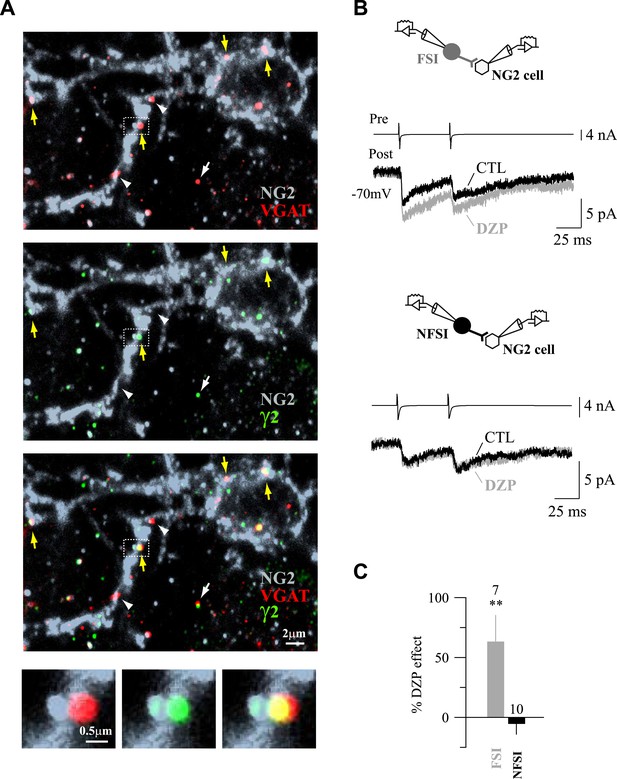
Expression of γ2 subunit of GABAARs at FSI-NG2 cell synapses.
(A) Confocal images of VGAT+ (red) and γ2+ (green) puncta on NG2+ membranes (gray) of a NG2 cell at PN10 (objective 63×; stack of 8 Z sections, each 0.32 µm). As expected from previous data (Balia et al., 2015), numerous VGAT+/γ2+ (yellow arrows) and VGAT+/γ2− (white arrowheads) puncta on NG2+ soma and branches were observed. Note that neuronal VGAT+/γ2+ puncta are clearly distinguished from those present on NG2 cells at this developmental stage (white arrow). (B) DZP effect on PSC amplitudes in two NG2 cells connected, respectively, to a FSI (top) and a NFSI (bottom). (C) Histogram comparing DZP effect on PSCs evoked by FSIs and NFSIs. The number of tested cells is indicated on top of histogram bars. **p < 0.01.
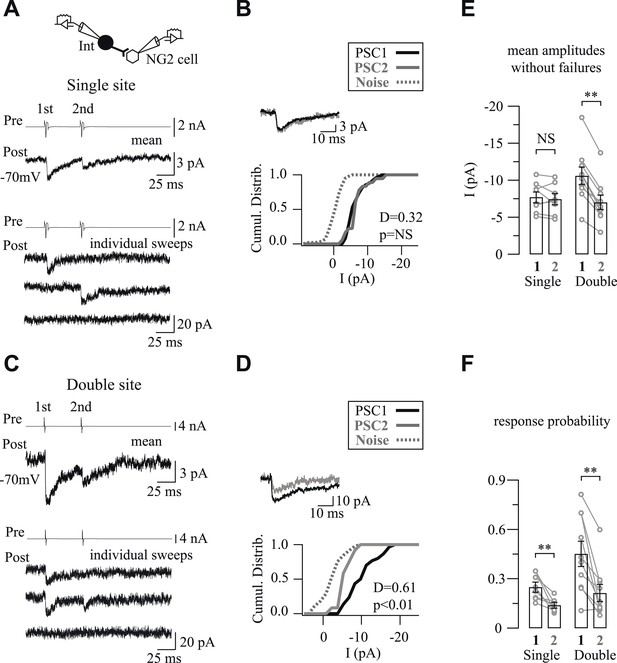
One or two release sites per interneuron-NG2 cell connection.
(A, C) Unitary connections with single (A) or double (C) vesicular release. Mean (middle traces) and individual (bottom traces) PSCs recorded in NG2 cells in response to two action currents evoked in presynaptic interneurons (top traces). (B, D) Cumulative distributions of PSC1 and PSC2 without failures for connections shown in A and C. Insets show superimposed mean PSC1 and PSC2 without failures. (E, F) Histograms of amplitudes without failures (E) and response probabilities (F) of PSC1 and PSC2 for connections with single and double vesicular release.
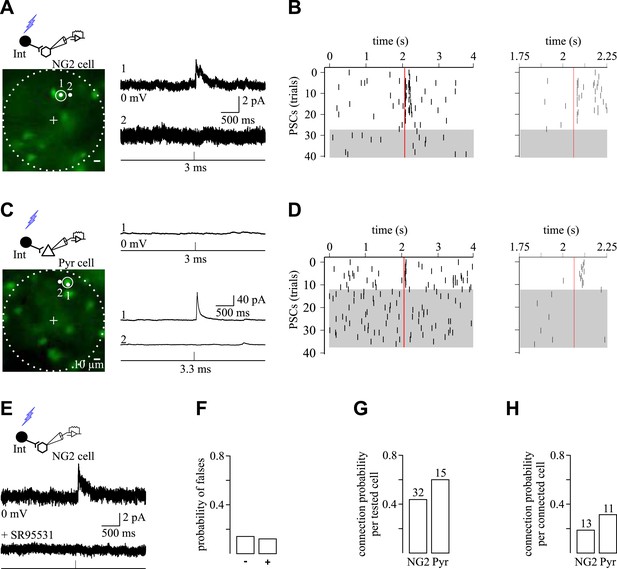
Spatial selectivity of holographic photostimulation to detect unitary interneuron-NG2 cell connections.
(A, C) Excitation fields (dashed circles) in epifluorescent images of Venus+ interneurons. Recorded NG2 cell (A) and pyramidal neuron (C) are in the center (+, non-visible). A 3-ms photostimulation of an interneuron (A; spot 1) induces unitary PSCs in a NG2 cell held at 0 mV and recorded with a CsMeS-based intracellular solution (top trace; average of 28 traces). The spatial selectivity of this connection is confirmed by displacing the illumination spot near the targeted soma (spot 2; bottom trace; average of 12 traces). (B) Raster plot of GABAergic synaptic events from the recorded NG2 cell illustrated in A. Each tick corresponds to a PSC. Note that random and sparse spontaneous synaptic currents are observed 2 s before and after interneuron photostimulation (red vertical line), whereas photo-evoked synaptic events reproducibly occur within 100 ms after the photostimulation. Photo-evoked events disappear when the 5-µm spot is moved to spot 2 (gray box). Note that in some single traces more than one postsynaptic event was elicited upon photo-stimulation (insets right) and that the average response in A displays two peaks. In these examples, the targeted presynaptic interneuron probably elicited more than one action potential. (C) A 3-ms photostimulation of an interneuron (C; spot 1) does not induce unitary synaptic currents in a pyramidal neuron held at 0 mV and recorded with a CsMeS-based intracellular solution (top trace). The excitation time was increased to test for a possible false negative connection. An increase in the excitation time of the interneuron to 3.3 ms induces unitary PSCs in the pyramidal neuron (spot 1; middle trace; average of 11 traces) that disappear when the spot is displaced 5 µm apart, confirming the photostimulation selectivity (spot 2; bottom trace; average of 26 traces). (D) Raster plot of GABAergic synaptic events from the recorded pyramidal neuron illustrated in C. Note that random spontaneous synaptic currents are observed 2 s before and after interneuron photostimulation (3.3 ms; red vertical line), whereas synaptic events reproducibly occur within 100 ms after photostimulation (inset right). These events disappear when the 5-µm spot is moved to position 2 (gray box). Failures of response were rarely observed in pyramidal neurons. (E) Averaged unitary PSC photo-induced in a recorded NG2 cell (middle trace; average of 9 traces) and completely abolished by 5 µM SR95531 (bottom trace; average of 13 traces). (F) Probability of encountering false negative and false positive connections. Unspecific connections were discriminated by changing the pulse duration of the laser and the position of the spot as in A and C. (G, H) Connection probabilities for all tested cells (G) and for cells showing at least one connection (H).
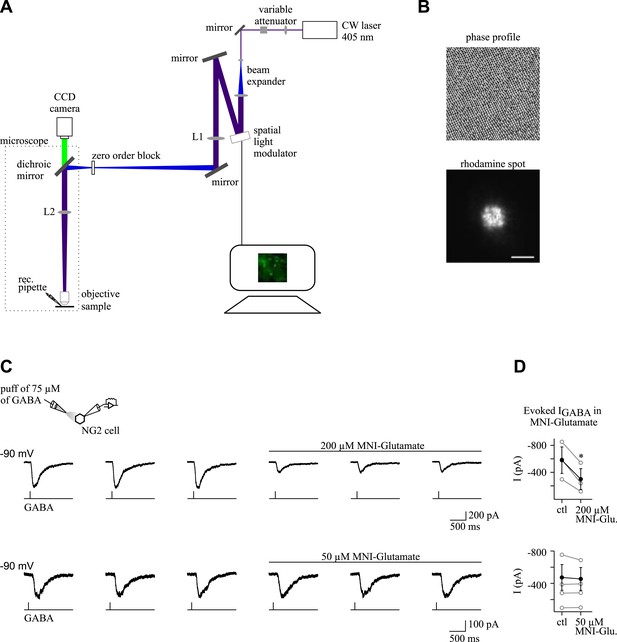
Optical set-up and effect of MNI-glutamate on GABAA receptor-mediated currents in NG2 cells.
(A) Optical set-up for holographic photolysis. The focal length of the lenses are f1 = 350 mm (L1) and f2 = 180 mm (L2; see ‘Materials and methods’ for details). (B) Phase hologram (left) used to create a 5-µm spot (right). This illumination spot used in the present study was visualized by exciting a thin layer of rhodamine (right). Scale bar: 5 µm. (C) GABA (75 µM) was prepared in the extracellular solution and applied by pressure from a patch pipette (250 ms, each 10–15 s; 0.52 bars). The application pipette was positioned at 50–100 µm from cells recorded in CsCl-based intracellular solution. Inward currents were elicited by local applications of GABA at a holding potential of −90 mV in control conditions and in the presence of MNI-glutamate. Note the amplitude reduction of elicited currents in 200 µM, but not in 50 µM of MNI-glutamate. (D) Plots of current amplitudes elicited in different NG2 cells in control conditions (ctl) and in the presence of MNI-glutamate (MNI-Glu). Mean amplitudes are shown in black (*p < 0.05).
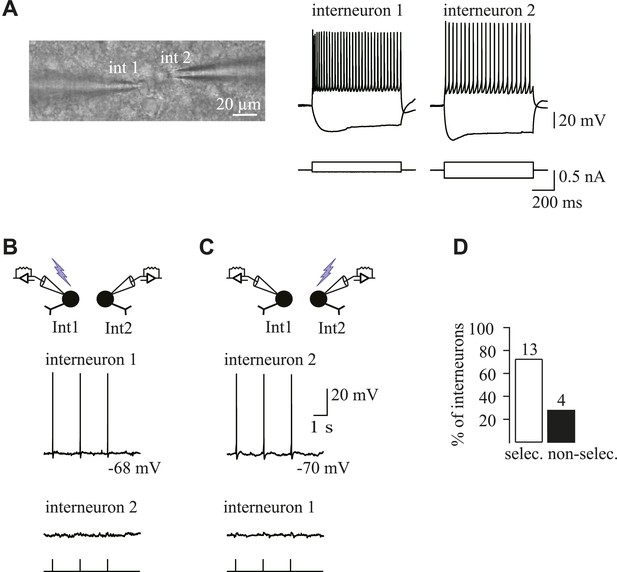
Spatial selectivity of holographic photostimulation of targeted Venus+ interneurons.
(A) DIC image of a simultaneous patch-clamp recording of two photostimulated Venus+ interneurons (left; fluorescence not shown). Note the close proximity of the two cell somata. Current-clamp recordings obtained for each recorded interneuron in response to current injections (right). (B, C) Simultaneous current-clamp recordings in response to single-cell photostimulation of either interneuron 1 (B) or interneuron 2 (C). Note that the laser pulse elicits a reproducible action potential in the target interneuron (top traces), but not in the nearby interneuron (bottom traces). (D) Histogram of the percentage of interneurons for which the photostimulation was selective when the illumination spot was moved onto the soma of the other interneuron. Note that the selectivity is attained in 76% of the cases. It is noteworthy that simultaneous recordings were performed between interneurons located at different planes and at very short intersomatic distances (mean intersomatic distance measured between the center of somata: 21.8 ± 2.0 µm, n = 17 simultaneous recordings). In addition, the time pulse used to test the spatial selectivity to generate action potentials in simultaneous recordings of two interneurons was always at least the minimal time needed to elicit an action potential in the nearby non-photostimulated interneuron.
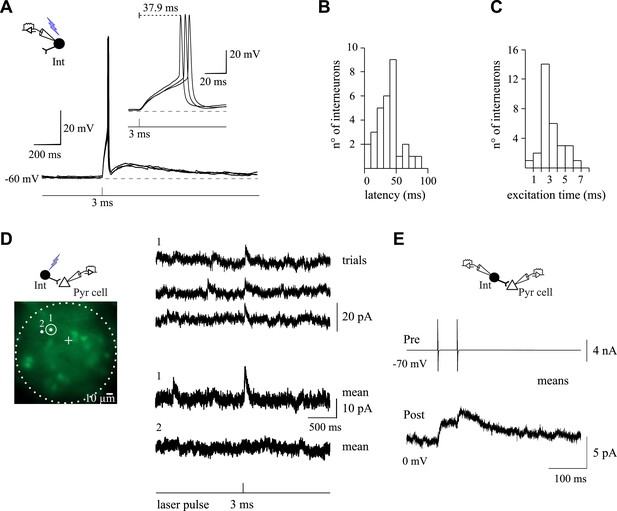
Monosynaptic connections with holographic photolysis.
(A) Current-clamp recordings of a Venus+ interneuron held at −60 mV in response to single-cell photostimulation. Note that a 3-ms laser pulse elicits an action potential in the target interneuron with a delay of several tens of ms and a jitter in the spike peak (inset). (B) Histogram of latencies between the beginning of the laser pulse and the peak of the photo-evoked action potential for recorded interneurons. Note that this latency can reach 100 ms. (C) Histogram of minimal excitation time needed to photo-evoke a single action potential in different Venus+ interneurons. Most interneurons are photo-activated by less than 3-ms pulses. (D) Excitation field (dashed circles) in epifluorescent images of Venus+ interneurons. A 3-ms photostimulation of an interneuron (spot 1) induces unitary synaptic currents in a recorded pyramidal neuron (+, non-visible) held at 0 mV and recorded with a CsMeS-based intracellular solution (top traces). The spatial selectivity of this connection is confirmed by displacing the illumination spot near the targeted soma (spot 2; bottom trace). (E) The connection in c was further confirmed to be monosynaptic by patching the interneuron with a second patch pipette. Two action currents evoked in the presynaptic interneurons (upper trace) elicits PSCs recorded in the postsynaptic neuron (bottom trace). This experiment was reproduced for 3 out of 3 postsynaptic cells.
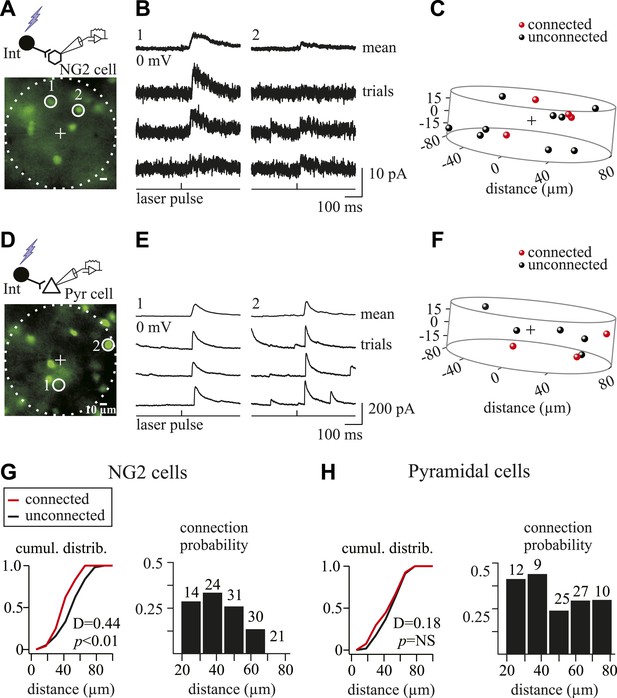
GABAergic connectivity maps of NG2 cells and pyramidal neurons.
(A, D) Excitation fields (dashed circles) in epifluorescent images of Venus+ interneurons. Recorded NG2 cell (A) and pyramidal neuron (D) are in the center (+, non-visible). (B, E) Photostimulation of interneurons (1 and 2) in A and D induces unitary PSCs in a recorded NG2 cell (B) and pyramidal neuron (E). (C, F) Connectivity maps within 1.05 × 106 µm3 volume of cells in A and D showing connected (red) and unconnected (black) interneurons. (G, H) Cumulative distribution of connected and unconnected interneurons (left) and distribution of connection probabilities in respect to intersomatic distances (right) between interneurons and either NG2 cells (n = 13) or pyramidal neurons (n = 11) displaying at least one connection.
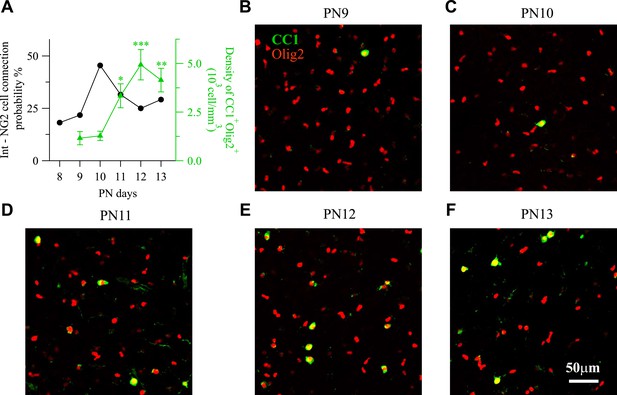
Time course of interneuron-NG2 cell connectivity and NG2 cell differentiation during the second PN week.
(A) Connection probability for interneuron-NG2 cell pairs and CC1+/Olig2+ cell density as a function of postnatal days (19–25 pairs tested per day). Note that the peak of NG2 cell connection probability at PN10 precedes a large increase in CC1+/Olig2+ differentiated oligodendrocytes at PN11. *p < 0.05; **p < 0.01, ***p < 0.001. (B–F) CC1+/Olig2+ and CC1−/Olig2+ cells in layer V at different PN days (stacks of 10 Z-sections; each 1 µm).
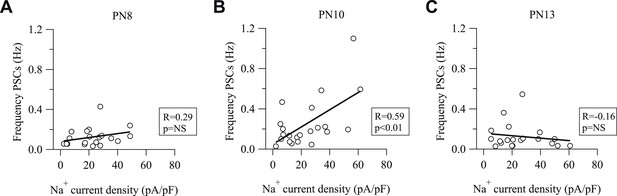
Na+ current density and frequency of spontaneous activity of NG2 cells correlates at PN10, but not at PN8 and PN13.
(A–C) Plot of synaptic current frequencies against Na+ current densities at PN8 (A), PN10 (B), and PN13 (C). There is a significant correlation only at PN10.
Tables
Electrophysiological properties of Venus+ FSI and NFSI
Parameter | FSI (n = 27) | NFSI (n = 105) | p | Comparison |
---|---|---|---|---|
Ftotal (Hz) | 28.32 ± 2.73 | 24.18 ± 0.93 | NS | – |
Finit (Hz) | 37.12 ± 3.89 | 45.29 ± 2.3 | NS | – |
F200 (Hz) | 29.55 ± 2.73 | 24.48 ± 0.89 | NS | – |
Ffinal (Hz) | 26.36 ± 2.72 | 21.11 ± 0.83 | NS | – |
Early accommodation | 14.14 ± 3.61 | 39.33 ± 1.85 | <0.0001 | FSI < NFSI |
Late accommodation | 11.43 ± 3.01 | 8.87 ± 0.68 | NS | – |
Threshold (mV) | −35.68 ± 0.64 | −36.31 ± 0.47 | NS | – |
First spike amplitude (mV) | 70.73 ± 2.33 | 70.61 ± 1.09 | NS | – |
Second spike amplitude (mV) | 70.55 ± 2.08 | 65.51 ± 1.3 | NS | – |
Spike amplitude reduction | −0.24 ± 0.94 | 7.59 ± 1.14 | <0.0001 | FSI < NFSI |
First spike duration (ms) | 1.37 ± 0.1 | 2.41 ± 0.1 | <0.0001 | FSI < NFSI |
Second spike duration (ms) | 1.46 ± 0.11 | 3.83 ± 0.22 | <0.0001 | FSI < NFSI |
Spike duration increase | 6.79 ± 0.75 | 55.06 ± 5.92 | <0.0001 | FSI < NFSI |
AHP (mV) | −15.89 ± 0.61 | −8.43 ± 0.4 | <0.0001 | FSI > NFSI |
AHP width (ms) | 25.57 ± 1.61 | 21.53 ± 1.05 | NS | – |
Peak to AHP trough (ms) | 7.18 ± 0.92 | 13.85 ± 0.88 | <0.0001 | FSI < NFSI |
AP-depolarizing slope (mV/ms) | 207.34 ± 12.86 | 140.45 ± 5.63 | <0.0001 | FSI > NFSI |
AP-hyperpolarizing slope (mV/ms) | −67.38 ± 4.35 | −32.81 ± 1.36 | <0.0001 | FSI > NFSI |
Rin (MΩ) | 208.22 ± 17.97 | 399.96 ± 19.75 | <0.0001 | FSI < NFSI |
-
For the identification of FSI and NFSI by firing properties, we first analyzed spike-frequencies in Venus+ interneurons during suprathreshold pulses in current clamp configuration from −70 mV (200 pA, 800–1000 ms). Firing frequency was calculated for the entire pulse as the number of spikes divided by the pulse duration (Ftotal). Three instantaneous discharge frequencies were also calculated: (1) between the first pair of spikes (Finitial); (2) at 200 ms from the beginning of the pulse (F200); and (3) at the end of the pulse (Ffinal). These values were used to quantify both early and late accommodations in accordance with the following formulas: (Finitial − F200/Finitial) and (F200 − Ffinal/Finitial), respectively. We also dissected the spike morphology from action potentials elicited by 80-ms suprathreshold pulses from −70 mV (150–200 pA). From these recordings, the spike threshold corresponded to the voltage at which the derivative of the AP (dV/dt) experienced a twofold increase. The amplitudes, the first and the second AP (A1 and A2), were calculated from the threshold to peak. Their duration (D1 and D2) corresponded to the full-width at half maximum (FWHM) from a Gaussian fit of the depolarized face of the AP immediately after the threshold. Both amplitude reduction and duration increase were calculated by the formulas A1 − A2/A1 and D2 − D1/D1, respectively. The amplitude of the after-hyperpolarization (AHP) was calculated as the difference between the threshold and the peak of the fast hyperpolarization. We also estimated the AHP width as the FWHM and the latency of AP peak to AHP trough. We extracted the positive and negative peaks from the derivative of the AP waveform to quantify the maximal speed excursion of the membrane voltage during both depolarizing and hyperpolarizing faces of the AP. Finally, the input resistance (Rin) was measured in current clamp by applying hyperpolarizing pulses from −60 mV (−200 pA). The three major parameters to differentiate FSI from NFSI appeared in bold. Note that seven other parameters also easily differentiate these interneurons. NS: no significant difference.
Additional files
-
Supplementary file 1
Synaptic coupling probabilities.
- https://doi.org/10.7554/eLife.06953.020