Daple is a novel non-receptor GEF required for trimeric G protein activation in Wnt signaling
Figures
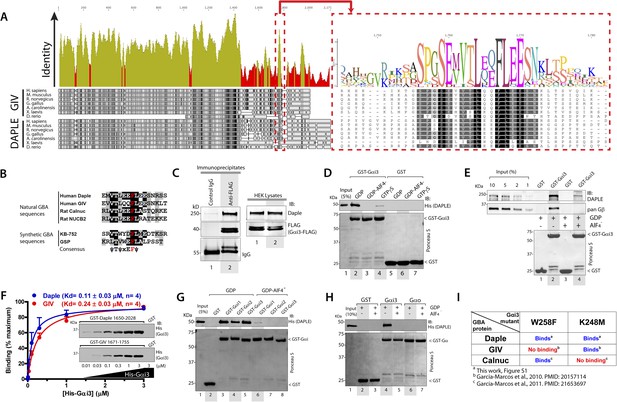
Daple contains a GBA motif.
(A) Phylogenetic sequence analysis reveals a conserved motif in Daple similar to GIV's Gα-binding and activating (GBA) motif within an otherwise highly divergent C-terminal domain. Sequences of GIV and Daple from different species were aligned and the degree of identity at each position plotted. A high degree of identity is observed in the N-terminal region (<∼aa 1400), whereas the C-terminal domain (>aa 1400) is highly divergent. The peak of highest identity (red box) within the C-terminal domain corresponds to the GBA motif (enlarged on the right). (B) Daple's putative GBA motif is similar to known GBA sequences. Alignment of the putative GBA motif of Daple with the natural GBA sequences of GIV, Calnuc and NUCB2, and the synthetic GBA sequences of KB-752 and GSP peptides. Consensus is shown below (ψ = hydrophobic, x = any). (C) Full-length Daple binds to Gαi3 in cells. Equal aliquots of lysates of HEK293 cells expressing Gαi3-FLAG were incubated with anti-FLAG mAb or control IgG and protein G beads. Immune complexes were analyzed for Daple and Gαi3 (FLAG) by immunoblotting (IB). Gβ was monitored as positive Gαi3-binding control. (D) Purified Daple binds directly to inactive but not active Gαi3. Purified, recombinant GST-Gαi3 preloaded with GDP (inactive), GDP + AlF4− (active), or GTPγS (active) and immobilized on glutathione-agarose beads was incubated with purified His-Daple-CT (aa 1650–2028, containing the putative GBA motif) as indicated. Resin-bound proteins were eluted, separated by SDS-PAGE and analyzed by Ponceau S-staining and IB with the indicated antibodies. No binding to GST alone was detected. (E) Full-length Daple expressed in cells binds preferentially to inactive vs active Gαi3. Purified, recombinant GST-Gαi3 preloaded with GDP (inactive) or GDP + AlF4− (active) and immobilized on glutathione-agarose beads was incubated with cell lysates of Cos7 cells expressing full-length myc-Daple as indicated. Bound proteins were analyzed for Daple (myc) and Gβ by IB as in D. Binding of Gβ to inactive but not active Gαi3 was used as positive control. No binding of myc-Daple or Gβ to GST alone was detected. (F) Daple and GIV bind to Gαi3 with comparable submicromolar affinities. Inset, Purified GST-Daple-CT and GST-GIV-CT (aa 1671–1755, containing the GBA motif) immobilized on glutathione-agarose beads were incubated with increasing amounts (0.01–3 µM) of purified His-Gαi3 (GDP-loaded) and binding analyzed by IB as described in (D). No binding to GST alone was detected at the highest His-Gαi3 concentration tested. Graph, Gαi3 binding was quantified by measuring band intensities and data fitted to a single-site binding hyperbola (Daple = BLUE, GIV = RED) to determine the equilibrium dissociation constants (Kd). Mean ± S.E.M of four independent experiments. (G) Daple binds to all three Gαi subunits. Binding of His-Daple-CT to GST-fused Gαi1, Gαi2, or Gαi3 in the inactive or active conformations was analyzed exactly as described in (D). (H) Daple selectively binds to Gαi, but not Gαo. Binding of His-Daple-CT to GST-fused Gαi3 or Gαo in the inactive or active conformations was analyzed exactly as described in (D). (I) Daple binds to Gαi3 mutants that do not bind to other GBA proteins. Table summarizing the binding properties of Gαi3 K248M and W258F mutants to Daple (from Figure 1—figure supplement 1) and GIV or Calnuc (Garcia-Marcos et al., 2010, 2011b).
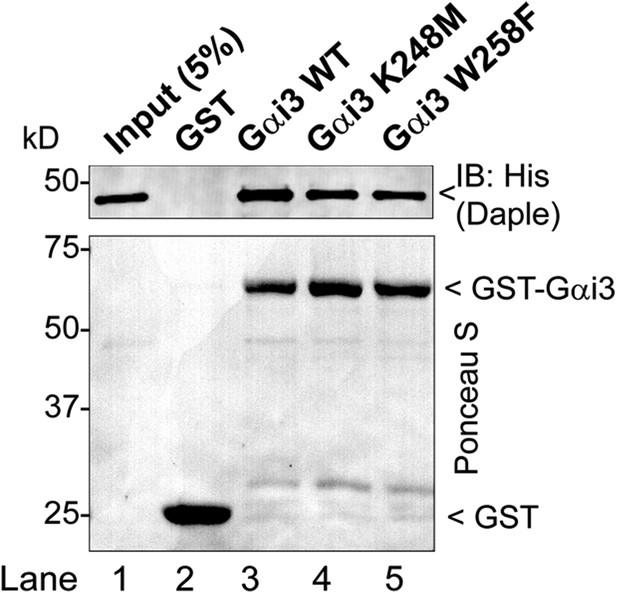
Daple binds mutants of Gαi3 that do not bind GIV (W258F) or Calnuc (K248M).
Purified, recombinant GST-Gαi3 (WT and mutants) preloaded with GDP and immobilized on glutathione-agarose beads was incubated with purified His-Daple-CT (aa 1650–2028) as indicated. Resin-bound proteins were eluted, separated by SDS-PAGE, and analyzed by Ponceau S-staining and IB with anti-His antibodies. No binding to GST alone was detected.
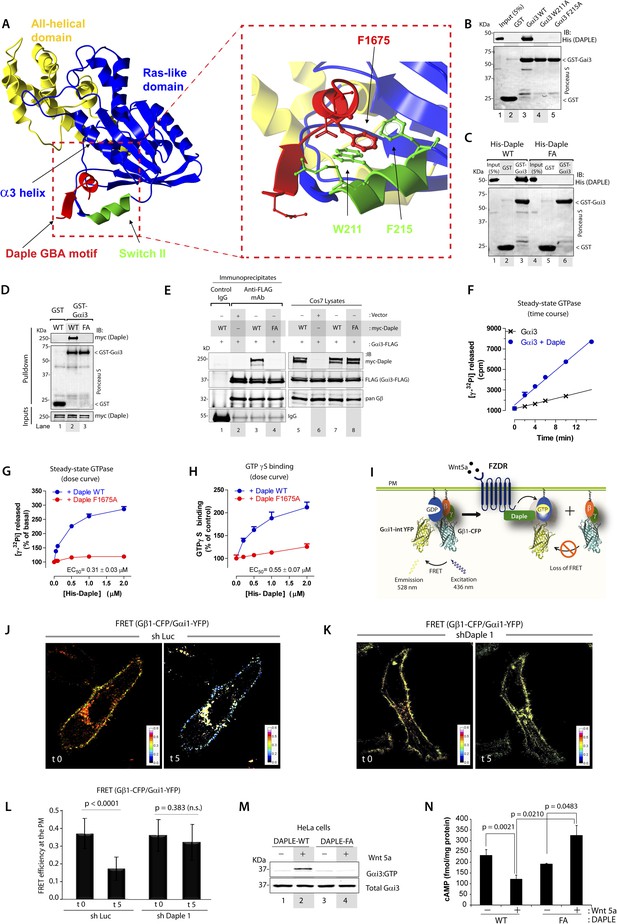
Daple binds and activates Gαi3 in vitro and in vivo via its GBA motif.
(A) Prediction of molecular contacts critical for the Daple-Gαi interaction. Homology-based model of Daple's GBA motif (Red) bound to Gαi3 (green = Switch II, blue = ras-like domain, yellow, all-helical domain) with an enlarged section depicting a putative hydrophobic contact between Daple's F1675 and Gαi3's W211/F215. (B) Mutation of residues in the SWII region of Gαi3 disrupts Daple binding. Binding of His-Daple-CT to GST-Gαi3 WT, W211A, or F215A was analyzed exactly as described in Figure 1D. (C) Mutation of Daple F1675 to A abrogates Gαi3 binding. Binding of His-Daple-CT WT or F1675A (FA) to GST-Gαi3 was analyzed exactly as described in Figure 1D. (D) F1675A mutation disrupts binding of full-length Daple expressed in cells to Gαi3. Myc-Daple WT or F1675A (FA) was expressed in Cos7 cells and binding to GST-Gαi3 analyzed exactly as described in Figure 1E. (E) Binding of full-length Daple to Gαi3 in cells is abolished upon F1675A mutation. Lysates of Cos7 cells expressing Gαi3-FLAG and myc-Daple-WT or F1675A (FA) were incubated with anti-FLAG mAb and subsequently with protein G beads. Immune complexes were analyzed for Daple (myc) and Gαi3 (FLAG) by IB. Gβ was monitored as positive Gαi3-binding control. (F) Daple accelerates the rate of Gαi3 steady-state GTPase activity. The steady-state GTPase activity of His-Gαi3 alone (black) or in the presence of 2 µM His-Daple-CT (blue) was determined by measuring the production of [32P]Pi at different time points as described in ‘Materials and methods’. One experiment representative of 3 is shown. (G) Daple WT but not F1675A (FA) accelerates the rate of Gαi3 steady-state GTPase activity in a dose-dependent manner. The steady-state GTPase activity of His-Gαi3 was determined in the presence of increasing concentrations (0–2 µM) of His-Daple-CT WT (blue) or His-Daple-CT FA (red) by measuring the production of [32P]Pi at 15 min. Mean ± S.E.M of five independent experiments. (H) Daple WT but not F1675A dose-dependently accelerates the rate of GTPγS binding to Gαi3. GTPγ35S binding to His-Gαi3 at 15 min was determined in the presence of increasing concentrations (0–2 µM) of His-Daple-CT WT (blue) or His-Daple-CT FA (red). Mean ± S.E.M of four independent experiments. (I) Schematic for the Gαi1-intYFP and Gβ1-CFP constructs used as paired Fӧrster resonance energy transfer (FRET) probes in J, K, and L. (J–L) Heterotrimers of Gi1 (Gαi1 and Gβ1γ2) are dissociated at the plasma membrane (PM) in control (J, sh Luc), but not Daple-depleted (K, sh Daple 1) HeLa cells after Wnt5a stimulation. Control (Left) or Daple-depleted (Right) HeLa cells (sh Daple 1 described in Figure 2—figure supplement 1A,B) cotransfected with Gαi1-intYFP, Gβ1-CFP, and Gγ2 were maintained overnight in 0.2% FBS and subsequently stimulated with 0.1 mg/ml Wnt5a and analyzed for FRET by confocal microscopy. Representative freeze-frame images from live-cell movies are shown, which display intensities of acceptor emission due to FRET in each pixel. Activation of Gi, as determined by the loss of interaction (i.e., FRET) between Gαi1 and Gβ1γ2 was observed exclusively after ligand stimulation (compare t0 and t5) in control (J), but not in Daple-depleted HeLa cells (K). (L) Bar graphs display differences between FRET intensities observed in control vs Daple-depleted cells in (J, K). Error bars representing mean ± S.D. of 5 randomly chosen regions of interest (ROIs) at the PM per cell, from 4 to 5 cells per experiment, from three independent experiments. (M) HeLa cells expressing Daple-WT, but not Daple-F1675A activate Gαi3 in response to Wnt5a stimulation, as determined by immunoprecipitation (IP) with conformationally-sensitive anti-Gαi:GTP antibodies. Daple-depleted HeLa cells transiently transfected with myc-Daple WT or F1675A (FA) were serum-starved and treated (+) or not (−) with 0.1 mg/ml Wnt5a for 20 min were subjected to immunoprecipitation with antibodies that selectively recognize active Gαi subunits in their GTP-bound state. Immune complexes (top) and lysates (bottom) were analyzed for active Gαi3:GTP and total Gαi3 by immunoblotting (IB) with anti-Gαi3 antibody. (N) HeLa cells expressing Daple-WT, but not Daple-F1675A inhibit cAMP in response to Wnt5a stimulation, as determined by radioimmunoassay. HeLa cells transiently transfected with myc-Daple WT or F1675A (FA) incubated with forskolin and PDE inhibitors for 10 min, treated (+) or not (−) with 0.1 mg/ml Wnt5a for 20 min and cAMP levels quantified as detailed in ‘Materials and methods’. Mean ± S.D. of three independent experiments.
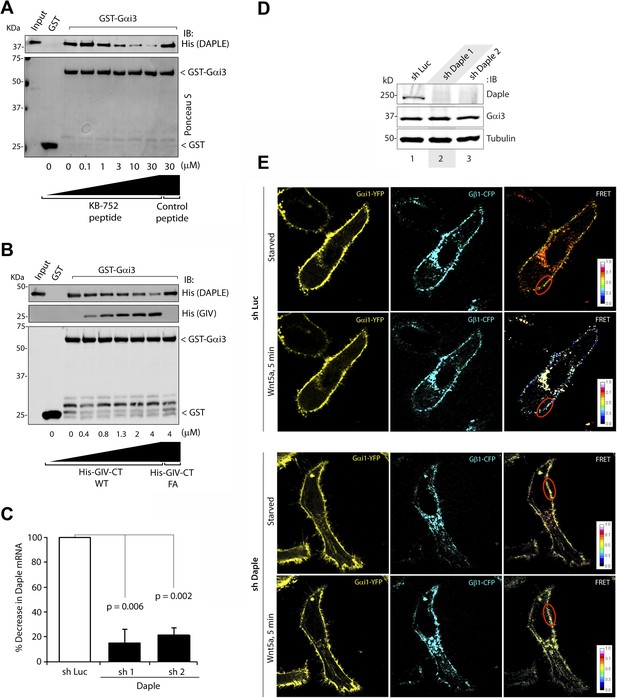
Binding of Daple to Gαi triggers activation of Gi at the PM after Wnt5a stimulation.
(A, B) Daple competes for binding to Gαi3 with peptides/proteins that dock onto the switchII/α3 cleft of the G protein. (A) Purified, recombinant GST-Gαi3 preloaded with GDP and immobilized on glutathione-agarose beads (∼0.3 mM) was incubated with a fixed concentration (∼0.2 mM) of purified His-Daple-CT (aa 1650–2028) in the presence of the indicated concentrations of KB-752 or a control peptide. Resin-bound proteins were eluted, separated by SDS-PAGE, and analyzed by Ponceau S-staining and IB with anti-His antibodies. No binding to GST alone was detected. One experiment representative of 3 is shown. (B) Analogous experiments to those described in A were carried out using His-GIV-CT WT and His-GIV-CT F1685A (as negative control) instead of peptides. (C–E) Daple is essential for activation of trimeric Gi at the PM after Wnt5a stimulation. (C, D) Two independent shRNA sequences targeting the 3′ UTR of the gene efficiently (<90%) deplete Daple mRNA (A) and protein (B) from HeLa cells. The knock-down efficiency was assessed by comparing Daple mRNA by quantitative PCR (qPCR) (C) or protein by IB (D) on HeLa cells stably expressing two Daple-targeting (shDaple1 and shDaple2) or control (shRNA targeting luciferase [shLuc]) shRNA sequences. (E) Control (Luc shRNA) or Daple-depleted (Daple shRNA1) HeLa cells co-transfected with Gαi1-YFP, Gβ1-CFP, and Gγ2 were starved overnight in media containing 0.2% FBS prior to stimulation with Wnt5a and analyzed for FRET using confocal microscope. Representative freeze-frame images from live-cell movies are shown, which display acceptor (Gαi1-YFP), donor (Gβ1-CFP) and intensities of acceptor emission due to FRET in each pixel (from left to right). Activation of Gi, as determined by the loss of interaction (i.e., FRET efficiency) between Gαi1-YFP and Gβ1-CFP is observed at the PM exclusively after ligand stimulation (compare t0 and t5) in Luc shRNA treated control cells, but not in Daple-depleted cells. Red circle = ROI at the PM.
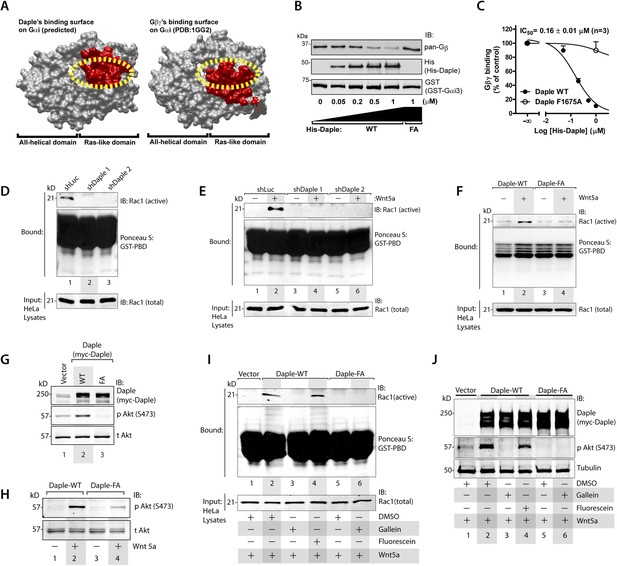
Daple's GBA motif triggers the release of ‘free’ Gβγ subunits, which in turn enhance Rac1 and PI3K-Akt signaling.
(A) Daple's GBA motif and Gβγ subunits are predicted to dock onto an overlapping binding site on Gαi. Binding areas (in red) for Daple (left) or Gβγ (right) on Gαi (solid gray) were extracted from a homology-based model of Daple-Gαi3 and the crystal structure of the Gαi1·Gβγ complex (Protein Data Bank [PDB]: 1GG2), respectively. (B, C) Daple displaces Gβγ subunits from Gαi3 via its GBA motif. GST-Gαi3·Gβγ preformed complexes immobilized on glutathione beads were incubated with increasing concentrations of His-Daple-CT WT or F1675A (FA). Bound proteins were analyzed by IB (B) and Gβγ binding data fitted to a single-site competition curve (C). Mean ± S.E.M. of three independent experiments. (D, E) Activation of Rac1 is impaired in Daple-depleted HeLa cells. Control (shLuc) or two clones of Daple-depleted HeLa cell lines (sh Daple 1 and 2) (described in Figure 2—figure supplement 1A,B) were incubated in 2% serum media (D) or starved and treated (+) or not (−) with Wnt5a (0.1 mg/ml) for 5 min (E) and analyzed for Rac1 activation by pulldown assays using GST-PBD. (F) Activation of Rac1 is impaired in cells expressing Daple-F1675A (FA) mutant compared to those expressing Daple-WT. Daple-depleted (sh Daple 1) HeLa cells transiently transfected with myc-Daple-WT or FA were starved and stimulated with Wnt5a and analyzed for Rac1 activation as in E. (G, H) Daple's GBA motif is required for activation of PI3K-Akt signaling in HeLa cells, as determined by phosphorylation of Akt at S473. Daple-depleted (sh Daple 1) HeLa cells transiently transfected with myc-Daple WT or F1675A (FA) were incubated in a 2% serum media (G) or in a 0.2% serum media overnight and treated (+) or not (−) with 0.1 mg/ml Wnt5a for 5 min (H) prior to lysis. Equal aliquots of whole-cell lysates were analyzed for Akt phosphorylation (pAkt S473) by IB. (I, J) Inhibition of Gβγ signaling impairs Daple-dependent activation of Rac1 and Akt. Daple-depleted (sh Daple 1) HeLa cells transiently transfected with myc-Daple WT were treated with DMSO, 10 µM of the Gβγ inhibitor gallein or its inactive analog fluorescein for 6 hr, as indicated, and analyzed for Rac1 (I) or Akt (J) activation by IB or pulldown assays, respectively.
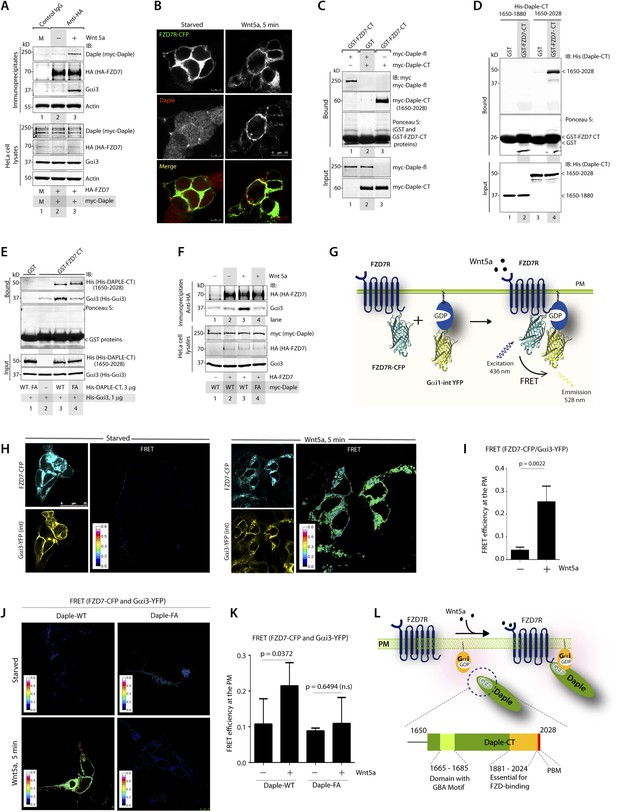
The C-terminus of Daple directly binds ligand-activated FZDRs and triggers the assembly of FZDR-Gαi complexes at the PM.
(A) Daple and Gαi3 co-immunoprecipitate with FZD7R after Wnt5a stimulation. HeLa cells cotransfected with myc-Daple WT and HA-FZD7 were starved and stimulated with Wnt5a as in 3G. Equal aliquots of lysates (bottom) were then incubated with anti-HA mAb and subsequently with protein G beads. Immune complexes (top) were analyzed for myc (myc-Daple) and endogenous Gαi3 by IB. (B) Daple is recruited to the PM after Wnt5a stimulation, where it colocalizes with FZD7R. HEK293 cells expressing FZD7-CFP (pseudocolored green) were grown on cover slips coated with Poly-D-Lysine, starved for 24 hr (0% FBS) and treated with 0.1 mg/ml Wnt5a as in 4A. Cells were fixed and stained for Daple (red) and analyzed by confocal microscopy. (C) The C-terminal region (1650–2028 aa) is sufficient for Daple to bind FZD7R. Lysates of Cos7 cells expressing full-lenght myc-Daple-WT or myc-Daple-CT (1650–2028 aa) were incubated with recombinant GST-FZD7-CT immobilized on glutathione-agarose beads in pulldown assays. Bound Daple (myc) was analyzed by IB. (D) Daple directly binds FZD7R and the extreme C-terminus (1881–2028) is essential for the interaction. His-Daple-CT (1650–2028 aa) or a shorter fragment of Daple-CT (1650–1880 aa) was incubated in pulldown assays with immobilized GST-FZD7-CT exactly as above. Bound Daple-CT (His) was analyzed by IB. (E) Daple's GBA motif is required for enhanced binding of Gαi3 to cytoplasmic tails of FZD7R in vitro. His-Gαi3 preloaded with GDP was incubated with immobilized GST-FZD7-CT, either alone (lane 2) or in the presence of His-Daple-CT (1650–2028 aa) WT (lane 3) or FA (lane 4) in pulldown assays as described in D. Bound Gαi3 and Daple-CT were detected by IB. (F) Daple's GBA motif is essential for the co-IP of Gαi3 with ligand-activated FZD7Rs. HeLa cells cotransfected with HA-FZD7 and myc-Daple-WT or FA were starved and subsequently stimulated with Wnt5a prior to lysis as in A. Equal aliquots of lysates (bottom) were incubated with anti-HA antibodies and subsequently with protein G beads. Immune complexes were analyzed for the presence of Gαi3 by IB. (G–I) Wnt5a stimulates formation of FZD7R-Gαi3 complexes at the PM in HEK293T cells. (G) Schematic of the FRET probes used in H. (H) HEK293 cells were cotransfected with FZD7-CFP and Gαi3-YFP, starved, and subsequently stimulated with Wnt5a and analyzed for FRET using confocal microscopy. Image panels display CFP, YFP, and intensities of acceptor emission due to FRET in each pixel. FRET was observed after Wnt5a stimulation (right). (I) Bar graphs display FRET efficiency observed at the PM in starved vs Wnt5a stimulated cells in H. Error bars represent mean ± S.D. The analysis represents 5 randomly chosen ROIs at the PM per cell, from 4 to 5 cells per experiment, from three independent experiments. (J, K) Daple's GBA motif is essential for the assembly of FZD7R-Gαi3 complexes at the PM. HEK293T cells were cotransfected with FZD7-CFP, Gαi3-YFP and myc-Daple (WT or FA), starved, and subsequently stimulated with Wnt5a prior to fixation. Fixed cells were stained for Daple (632 nm, far red; see Figure 4—figure supplement 2) and analyzed for FRET using confocal microscope. Image panels display the intensities of acceptor emission due to FRET in each pixel. FRET was observed in cells expressing Daple-WT, but not in cells expressing Daple-FA. (K) Bar graphs display the FRET efficacy observed in Daple WT vs Daple FA cells before (−) and after (+) Wnt5a stimulation. Error bars representing mean ± S.D. The analysis was done exactly as in H, I. (L) Schematic summary. Upon stimulation with Wnt5a, Daple's C-terminus enables the formation of FZD7R-Daple-Gαi3 complexes at the PM. Two distinct interaction modules present in-tandem within the C-terminus of Daple, the GBA motif, and the FZD-binding domain are essential for the formation of such complexes.
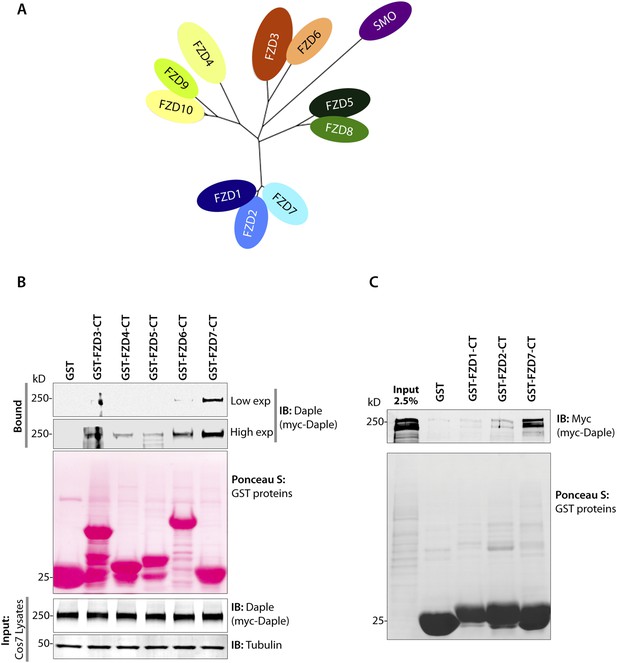
Daple preferentially binds the cytoplasmic tail of the FZD7R.
(A) A sequence homology-based cluster tree of vertebrate Frizzled receptors (FZDRs) is shown. The FZD (IUPHAR nomenclature) family roughly clusters into four distinct families based on sequence identity (modified from Verkaar and Zaman, 2010): (I) FZD 1, 2, and 7; (II) Frizzled-5 and Frizzled-8; (III) Frizzled-3 and Frizzled-6; (IV) Frizzled-4, Frizzled-9, and Frizzled-10. The Smoothened (SMO) receptor is a distant relative of FZD receptors that functions in Hedgehog signal transduction. (B, C) Lysates of cells expressing myc-Daple full length was used as source of Daple in pulldown assays with immobilized recombinant GST-tagged C-termini of various FZDRs. Bound proteins were analyzed for Daple by IB. Full-length Daple binds preferentially to the cytoplasmic tail of FZD7R, to an intermediate extent to the cytoplasmic tail FZD6R and only weakly the cytoplasmic tails of other FZDRs.
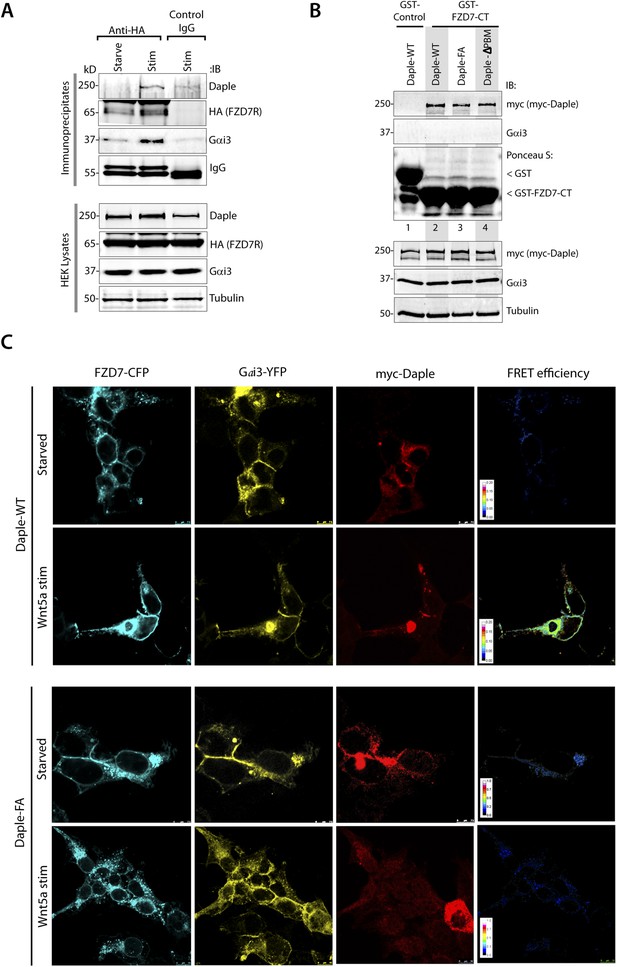
Daple binds to the C-terminus of FZD7R and links Gαi to ligand-activated receptors.
(A) HEK cells expressing HA-tagged FZD7R were starved for 24 hr (0% FBS) and stimulated with Wnt5a for 5 min as indicated prior to lysis. IP was carried out on lysates with anti-HA or control mouse IgGs and protein G beads. Equal aliquots of lysates (bottom) and immune complexes (top) were analyzed for Daple, Gαi3, FZD7R (HA), and tubulin by IB. Endogenous Daple and Gαi3 are recruited to FZD7R exclusively after Wnt5a stimulation. (B) Lysates of Cos7 cells expressing myc-tagged Daple-WT or GBA-deficient (FA) and PBM-deficient (ΔPBM) mutants were used as source of Daple in pulldown assays with GST-FZD7-CT immobilized on glutathione beads. Bound proteins were analyzed for Daple by IB. Mutant Daple proteins bound FZD7 as efficiently as Daple-WT. (C) HEK cells co-transfected with FZD7-CFP, Gαi3-YFP, and Daple-WT or FA were starved and subsequently stimulated with Wnt5a and analyzed for FRET using confocal microscope. Representative freeze-frame images from live-cell movies are shown, which display (from left to right) donor (FZD7-CFP), acceptor (Gαi3-YFP), Daple (far-red; 632 nm) and intensities of acceptor emission due to FRET in each pixel. Interaction (i.e., FRET) is observed exclusively after Wnt5a stimulation in cells expressing Daple-WT, but not Daple-FA.
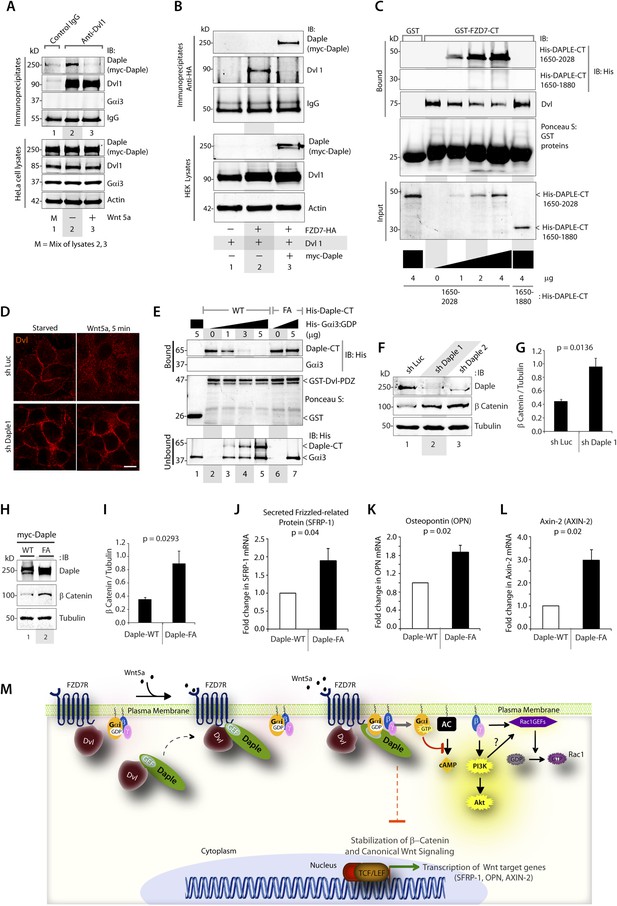
Daple competes with Dvl for binding to FZD7R and inhibits the canonical β-catenin/TCF/LEF signaling pathway via the GBA motif.
(A) Dishevelled (Dvl)–Daple complexes are disrupted upon Wnt5a stimulation. HeLa cells cotransfected with myc-Daple-WT and Dvl were incubated in a 0.2% serum media overnight, and treated (+) or not (−) with 0.1 mg/ml Wnt5a for 5 min prior to lysis. Equal aliquots of lysates (bottom) were incubated in the presence of anti-Dvl mAb and subsequently with protein G beads. Immune complexes (top) were analyzed for Daple (myc), Dvl, and Gαi3 by IB. (B) Dvl and Daple compete for recruitment to FZD7 receptor in cells. Equal aliquots of lysates of HEK293 cells cotransfected with FZD7-HA with Dvl and/or myc-Daple-WT were incubated with anti-HA mAb and subsequently with protein G beads. Immune complexes were analyzed for Daple and Dvl by IB. (C) Daple can displace Dvl bound to the cytoplasmic tail of FZD7R in vitro. Dvl expressed in HEK cells was pre-bound to GST or GST-FZD7CT and subsequently incubated with increasing amounts of recombinant His-Daple-CT proteins as indicated. Bound proteins were analyzed for Daple (His) and Dvl by IB. (D) Daple is required for the ligand-stimulated dissociation of Dvl from the PM. Control (sh Luc) and Daple-depleted (sh Daple 1) Hela cells coexpressing Dvl and FZD7R were starved and stimulated with Wnt5a prior to fixation as in 4B. Fixed cells were stained for Dvl (red) and analyzed by confocal microsocpy. Bar = 10 µM. (E) Gαi competes with Dvl for binding to Daple in vitro. Equal aliquots of GST or GST-Dvl-PDZ (immobilized on glutathione beads) and Daple-CT (WT or FA) recombinant proteins were incubated with increasing amounts of purified His-Gαi3 as indicated. Bound (top) and unbound (supernatant; lower) proteins were analyzed for Daple-CT and Gαi3 (His) by IB. GST and GST-Dvl-PDZ were visualized by ponceau staining. (F) Depletion of Daple increases the levels of β-catenin. Whole-cell lysates of control (shLuc) and Daple-depleted (shDaple1 and 2) HeLa cells were analyzed for β-catenin by IB. (G) Bar graphs display quantification of β-catenin in F. Error bars represent mean ± S.D of three independent experiments. (H) Daple's GBA motif is required for suppression of β-catenin expression/stability. Whole-cell lysates from HeLa cells transfected with myc-Daple-WT or FA were analyzed for β-catenin expression by IB. Two biological replicates are shown. (I) Bar graphs display quantification of β-catenin in H. Error bars represent mean ± S.D of three independent experiments. (J, K, L) Daple's GBA motif is required for suppression of Wnt target genes. HeLa cells transfected with myc-Daple-WT or FA were analyzed for SFRP-1, OPN, AXIN-2 mRNA by qPCR. Results were normalized internally to mRNA levels of the housekeeping gene, GAPDH. Bar graphs display the fold change in each RNA (y axis) in cells expressing Daple-FA normalized to the expression in cells expressing Daple-WT. Error bars represent mean ± S.D of three independent experiments. (M) Schematic of working model. (From left to right) In the absence of Wnt5a ligand, Dvl remains at the PM complexed to inactive FZD7Rs, whereas Daple remains in the cytosol in complex with cytosolic Dvl, and Gαi/βγ trimers at the PM are largely inactive. Upon ligand stimulation, Dvl-Daple complexes dissociate and Daple is recruited to the cytoplasmic tails of activated receptors, Dvl is displaced from the receptor tail by Daple, Daple favors the assembly of receptor-Gαi complexes and triggers the activation of Gαi within these complexes. Activated Gαi and Gβγ subunits trigger signaling via their respective downstream intermediates (Rac1, PI3K, and cAMP). Another major consequence of these signaling events is suppression of the canonical β-catenin/TCF/LEF signaling pathway, which regulates the transcription of Wnt target genes.
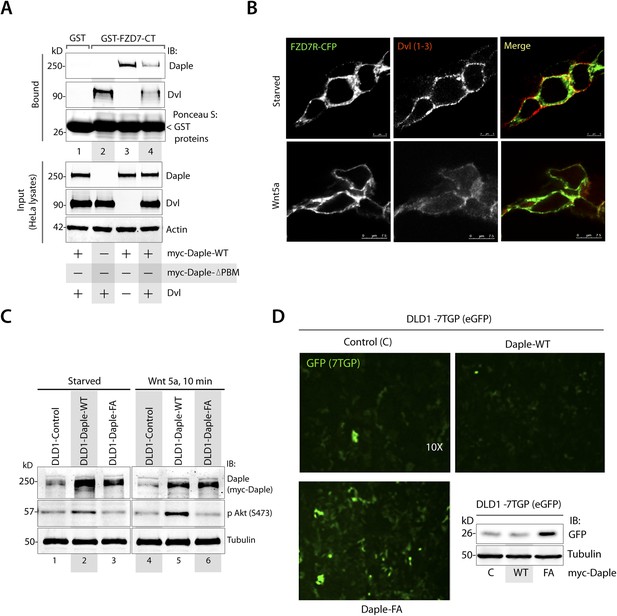
Daple competes with Dvl for binding to FZD7R and inhibits the canonical β-caenin/TCF/LEF signaling pathway.
(A) Equal aliquots of lysates from Cos7 cells expressing Dvl1 alone (lane 2), myc-Daple alone (lane 3), or coexpressing both (lanes 1, 4) were used as source of Daple and Dvl in GST pulldown assays with recombinant, immobilized GST or GST-FZD7-CT. Bound proteins were analyzed for Dvl1 and Daple by immunoblotting (IB). Binding of each protein was higher when expressed alone (lanes 2, 3) than when co-expressed (lane 4). (B) Dvl loses colocalization with FZD7R at the PM after Wnt5a stimulation. HEK293 cells expressing FZD7-CFP were grown on cover slips coated with Poly-D-Lysine, starved overnight, and treated with 0.1 mg/ml Wnt5a as in 4B. Cells were fixed and stained for endogenous Dvl (red) and analyzed by confocal microscopy. (C, D) Generation and characterization of DLD1 7TGP cell lines stably expressing Daple. (C) DLD1 7TGP cell lines stably expressing Daple-WT or FA were starved and stimulated analyzed for Daple expression and phosphorylation of Akt by immunoblotting (IB). (D) Images display representative fields from monolayers of DLD1 cells grown in 0.2% FBS by fluorescence microscopy. The intensity of eGFP signals denotes Wnt transcriptional activity. Inset shows immunoblots (IB) of equal aliquots of whole-cell lysates of DLD1-7TGP cells expressing control vector, Daple-WT, or Daple-FA. Compared to DLD1 cells expressing Daple-WT, those expressing Daple-FA also express higher levels of GFP protein, indicative of higher Wnt transcriptional activity.
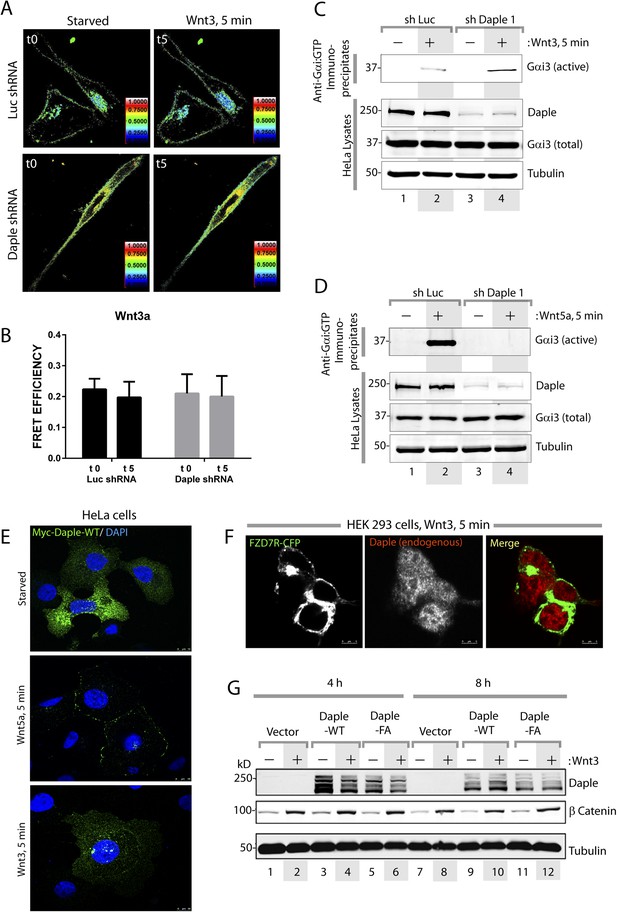
Daple and its GBA motif do not affect canonical Wnt signaling.
(A–D) Daple does not activate Gi after Wnt3 stimulation. (A, B) Control (Luc shRNA) or Daple-depleted (Daple shRNA) HeLa cells were cotransfected with Gαi1-YFP, Gβ-CFP, and untagged Gγ, serum starved overnight (0.2% FBS) and subsequently stimulated with either Wnt3 and analyzed for FRET by confocal microscopy. Representative freeze-frame images from live-cell movies are shown (A), which display intensities of acceptor emission due to FRET in each pixel. Activation of Gi was insignificant, as determined by continued interaction (i.e., continued FRET) between Gαi1 and Gβ1γ2 both before and after Wnt3 stimulation (compare t0 and t5) both in control (Luc shRNA) and in Daple-depleted HeLa cells. Bar graphs (B) display FRET intensities observed in control (Luc shRNA) vs Daple-depleted HeLa cells. Error bars representing mean ± S.D. of 5 randomly chosen ROIs at the PM per cell, from 2 to 3 cells per experiment, from three independent experiments. These results are in striking contrast to the findings after Wnt5a stimulation (see Figure 2I–L in manuscript). (C, D) Control (Luc shRNA) or Daple-depleted (Daple shRNA) HeLa cells were serum-starved (0.2% FBS) and treated (+) or not (−) with Wnt3 (C) or Wnt5a (D) for 15 min prior to lysis. Equal aliquots of lists were subjected to IP with antibodies that selectively recognize active Gαi subunits in their GTP-bound state. Immune complexes (top) and lysates (bottom) were analyzed for active Gαi3:GTP and total Gαi3 by IB. Wnt5a robustly activates Gαi3, and this activation is abolished upon Daple depletion, whereas Wnt3 marginally activates Gαi3 and this activation is not diminished upon Daple depletion. (E) Myc-Daple is translocated to the PM after Wnt5a, but not after Wnt3 stimulation. HeLa cells were transfected with myc-tagged Daple-WT, serum starved overnight (0.2% FBS) and subsequently stimulated with either Wnt5a or Wnt3 as indicated. Cells were fixed at 5 min after ligand stimulation and analyzed for localization of myc-Daple (Green) by immunofluorescence. Myc-Daple was found in cytosolic distribution prior to ligand stimulation in starved cells. Upon stimulation with Wnt5a Daple was found to localize sharply at the PM. Upon stimulation with Wnt3 myc-Daple remained in cytosolic location. (F) Endogenous Daple is translocated to the PM after Wnt5a, but not after Wnt3 stimulation. HEK cells transfected with CFP-tagged FZD7R were serum starved for 24 hr (0% FBS), and subsequently stimulated with either Wnt5a or Wnt3 as indicated. Cells were fixed at 5 min after ligand stimulation and analyzed for localization of endogenous Daple by immunofluorescence. Daple was found in cytosolic distribution prior to ligand stimulation in starved cells (see Figure 4B). Upon stimulation with Wnt3, Daple remained in cytosolic location, however, upon stimulation with Wnt5a Daple was found to localize at the PM, where it colocalized with FZD7R (see Figure 4B). (G) Daple's GBA motif does not affect Wnt3-dependent stabilization of β Catenin. HEK293 cells were transfected with Daple-WT or FA mutant, serum starved (0% FBS) for 24 hr, and subsequently stimulated with Wnt3 for 4 hr (lanes 1–6), 8 hr (lanes 7–12), or 20 hr (lanes 13–18) prior to lysis. Equal aliquots of cytoplasmic extracts were analyzed for β Catenin, Daple, and tubulin by IB. β Catenin was stabilized (increased, compare even lanes with odd lanes) in each condition tested, without significant differences between Daple-WT vs Daple-FA at any time points observed. A representative experiment from a total of four independent experiments is shown.
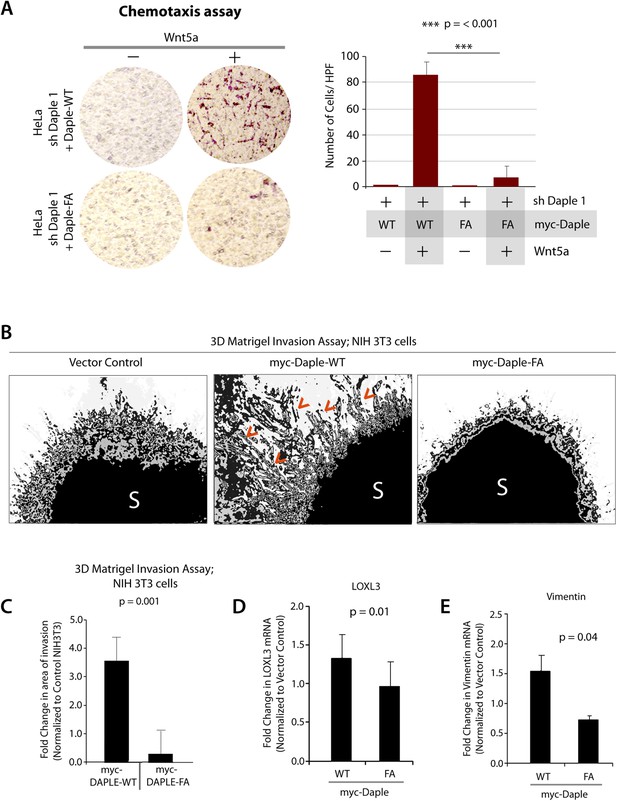
Daple enhances cell migration and invasion via its GBA motif.
(A) Daple WT, but not FA triggers chemotactic migration towards Wnt5a. Daple-depleted HeLa cells (sh Daple 1) stably expressing Daple-WT or Daple-FA were analyzed for their ability to migrate towards Wnt5a (+) or vehicle control (−) in transwell assays. Cells were allowed to migrate for 24 hr, fixed and stained with Giemsa. The number of migrating cells was averaged from 20 field-of view images per experiment. Data are presented as mean ± SEM; n = 3. HPF = high-power field. Lysates of cells used in this assay were analyzed for Daple expression by IB (see Figure 6—figure supplement 1C). (B, C) Daple WT, but not FA triggers cell invasion. Spheroids (S) of NIH3T3 cells expressing vector control, myc-Daple-WT, or FA were analyzed for their ability to invade matrigel in response to serum stimulation using a Cultrex-3D Spheroid Invasion Kit (Trevigen). An increase of invading cells (arrowheads; B) were noted only from the edge of tumor spheroids formed by cells expressing myc-Daple-WT, but not FA. Area of invasion was quantified using ImageJ (as shown with interrupted blue line in Figure 6—figure supplement 1D). (C) Bar graphs display area of invasion observed in Daple WT and Daple FA expressing cells. Error bars representing mean ± S.D of three independent experiments. (D, E) Daple-WT, but not Daple-FA enhances the expression of genes that trigger epithelial–mesenchymal transition (EMT). mRNA expression of the EMT markers, LOXL3, and Vimentin were analyzed by qPCR. Results were normalized internally to mRNA levels of the housekeeping gene, GAPDH. Bar graphs display the fold change in each RNA (y axis) normalized to the expression in cells expressing vector control. Error bars represent mean ± S.E.M of three independent experiments.
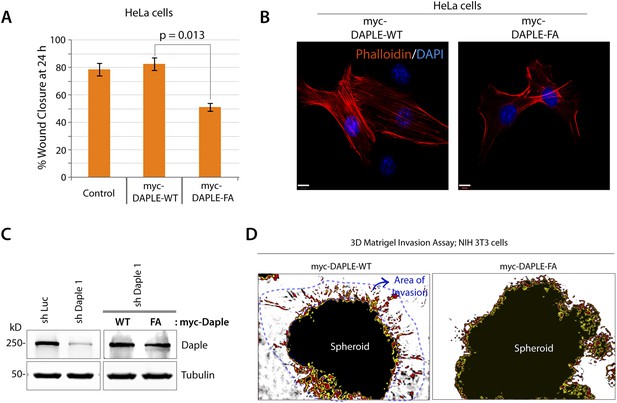
Daple enhances cell migration, promotes formation of actin stress-fibers, and triggers invasion, all via its GBA motif.
(A) Daple-FA, but not Daple-WT inhibits 2-D cell migration. Confluent monolayers of HeLa cells transiently transfected with myc-Daple WT or FA (∼90% efficacy of transfection confirmed by immunofluorescence) or control vector were scratch-wounded and incubated for 24 hr in a 0.2% serum media. Wound closure was monitored and quantified as detailed in ‘Materials and methods’. % wound closure (y axis) in various cell lines are displayed as bar graphs. For each cell line, ∼3–5 scratch-wounds were analyzed in each assay. Expression of Daple-FA significantly delays wound closure. Error bars represent mean ± S.E.M of three independent experiments. (B) Daple-WT, but not Daple-FA triggers formation of actin stress fibers. Daple-depleted HeLa cells transiently transfected with myc-Daple WT or FA were grown on cover slips in the presence of 0.2% FBS, fixed, and subsequently analyzed for actin cytoskeleton patterns by staining with Phalloidin (red). Abundance of stress fibers running across the cell bodies was seen in cells expressing Daple-WT. Blue = DAPI/nucleus. Bars = 10 µm. (C) Whole-cell lysates of HeLa cell lines used in transwell chemotaxis assays in Figure 6A were analyzed for Daple expression by IB. (D) Daple WT, but not FA triggers cell invasion. Spheroids of NIH3T3 cells expressing myc-Daple WT and FA were analyzed for their ability to invade matrigel in response to serum stimulation using a Cultrex-3D Spheroid Invasion Kit (Trevigen; see ‘Materials and methods’). Tracks created by invading cells were noted only in cells expressing myc-Daple WT. Area of invasion was quantified using ImageJ (as shown with interrupted blue line). Bar graphs showing the quantification of the area of invasion are shown in Figure 6C.
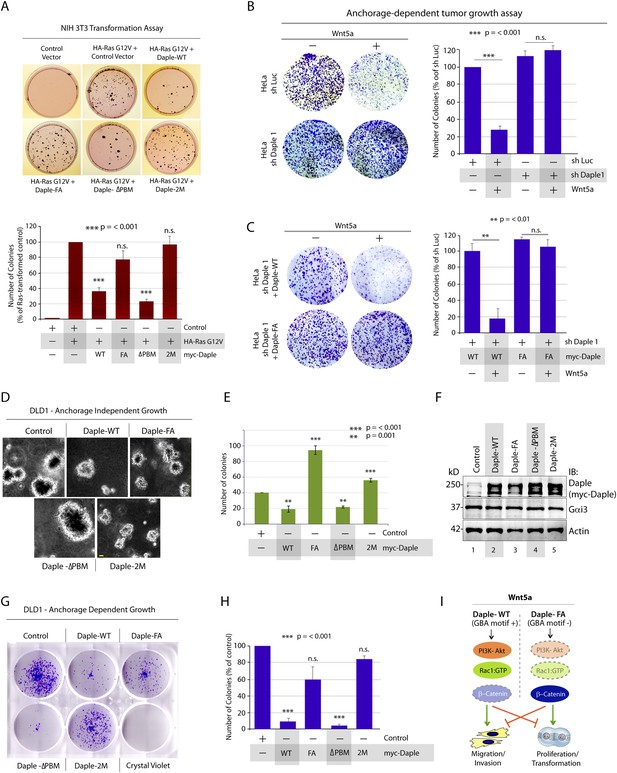
Daple suppresses proliferation and tumorigenesis via its GBA motif.
(A) Daple's GBA motif is required for inhibition of cell transformation induced by oncogenic KRas. NIH3T3 cells stably expressing HA-KRas G12V alone or coexpressing HA-KRas G12V with myc-Daple-WT or various mutants were analyzed for their ability to form colonies in soft agar prior to staining with MTT. The top panel displays representative images of colony-containing plates. Bar graphs in the lower panel shows % inhibition of colony formation (y axis) by each Daple construct compared to NIH3T3 cells transformed with KRas G12V alone. Lysates of NIH3T3 cells were analyzed for Daple and Ras constructs by IB (see Figure 7—figure supplement 1B). (B) Daple is required for inhibition of anchorage-dependent tumor growth by Wnt5a. Control (shLuc) and Daple-depleted (sh Daple 1) HeLa cells were analyzed for their ability to form colonies on plastic plates in the presence (+) or absence (−) of Wnt5a during a 2-week period prior to fixation and staining with crystal violet. Left panel shows the photograph of the crystal violet-stained wells of a 6-well plate. The number of colonies was counted by ImageJ (Colony counter). Right panel shows bar graphs that display the % inhibition of colony formation (y axis) seen in each condition normalized to control (shLuc) HeLa cells. (C) Daple's GBA motif is required for inhibition of anchorage-dependent tumor growth by Wnt5a. Daple-depleted (sh Daple 1) HeLa cells stably expressing either Daple WT or FA were analyzed for their ability to form colonies on plastic plates in the presence (+) or absence (−) of Wnt5a prior to fixation and staining with crystal violet, photographed and analyzed as in B. Left panel shows the photograph of the crystal violet-stained wells of a 6-well plate. Right panel shows bar graphs that display the % inhibition of colony formation (y axis) seen in each condition normalized to control (shLuc) HeLa cells. (D–F) Daple's GBA motif is required for inhibition of anchorage-independent tumor growth. DLD1 cells expressing either control vector or various myc-Daple constructs were analyzed for their ability to form colonies in soft agar for 2–3 weeks. In panel D, representative fields photographed at 20× magnification are shown. The number of colonies was counted by light microscopy throughout the depth of the matrix in 15 randomly chosen fields. In panel E, bar graphs display the number of colonies (y axis) seen in each cell line in D. In panel F, lysates of DLD1 cells used in D were analyzed for Daple constructs by IB. (G, H) Daple's GBA motif is required for inhibition of anchorage-dependent tumor growth. DLD1 cells used in D were analyzed for their ability to form adherent colonies on plastic plates during 2–3 weeks prior to fixation and staining with crystal violet. In panel G, photograph of the crystal violet-stained 6-well plate is displayed. The number of colonies was counted by ImageJ (Colony counter). In panel H, bar graphs display the % inhibition of colony formation (y axis) seen in each cell line in G normalized to control DLD1 cells. (I) Schematic summary. Modulation of G protein activity by Daple's GBA motif is a key determinant of cellular phenotype(s) triggered by Wnt5a. In cells expressing Daple-WT, a functionally intact GBA motif (+) can activate Gαi, enhance PM-based motogenic signals (PI3K-Akt and Rac1 activation), trigger EMT and cell migration/invasion. In cells expression Daple-FA, without the functional GBA motif (−) G protein remains inactive, non-canonical Wnt signaling is suppressed, which increases stability of β-catenin and upregulation of Wnt target genes, resulting in increased transformation, proliferation, and tumor cell growth.
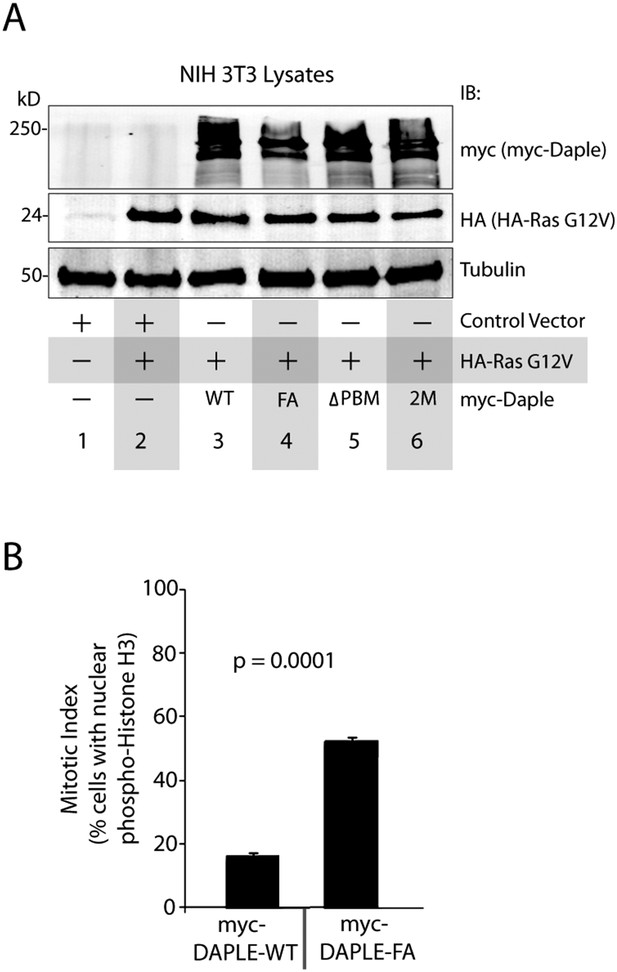
Daple suppresses cell proliferation via its GBA motif.
(A) Compared to cells expressing Daple-WT, those expressing Daple-FA have higher mitotic index, as determined by nuclear localization of phosphorylated histone H3. HeLa cells expressing myc-Daple WT or FA were grown on cover slips in the presence of 0.2% FBS, fixed, and stained for phospho-histone H3 and DAPI. Bar graphs display % cells with nuclear phospho-histone H3 (y axis). Error bars representing mean ± S.D. of three independent experiments. (B) Lysates of NIH3T3 cells NIH3T3 cells used in Ras-induced transformation assays (see Figure 7A) were analyzed for Daple and Ras constructs by IB.
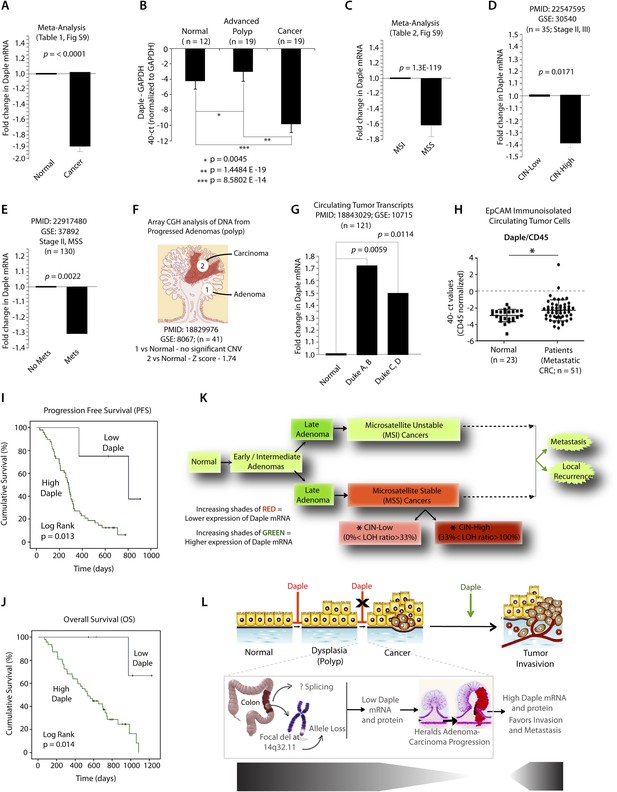
Expression of Daple mRNA is suppressed during oncogenesis by copy number loss, but expressed later during metastasis.
(A) Daple mRNA is downregulated in colorectal cancers. A meta-analysis was performed using all the available high-throughput microarray data from Genomic Spatial Event (GSE) database (see Figure 8—source data 1) to compare the levels of expression of Daple mRNA in colorectal cancer vs matched normal controls. Bar graphs display the results of such meta-analysis as fold change in Daple mRNA (y axis) in colorectal carcinomas normalized to matched normal controls. (B) Daple mRNA is downregulated during the adenoma-to-carcinoma step of oncogenesis in the colon. Daple mRNA was analyzed by qPCR in normal colon, advanced adenomas, and colorectal carcinomas. Bar graphs display the relative levels of Daple mRNA normalized to GAPDH, as determined by the calculation 2 − ΔCT with reference to an absolute baseline CT of 40 cycles. Error bars represent mean ± S.D. (C) Daple mRNA is downregulated in microsatellite stable (MSS), but not microsatellite unstable (MSI) colorectal cancers. A meta-analysis was performed using all the available high-throughput microarray data from GSE database (see Figure 8—source data 2) to compare the levels of expression of Daple mRNA in MSI vs MSS colorectal cancers vs their respective matched normal controls. Bar graphs display the results of such meta-analysis as fold change in Daple mRNA (y axis) in colorectal carcinomas normalized to normal controls. (D) Downregulation in Daple mRNA in MSS colorectal cancers directly correlates with the degree of chromosomal instability (CIN) in the tumor. High-throughput microarray data from GSE database (PMID: 22547595, GSE: 30,540) were analyzed for the levels of expression of Daple mRNA in MSS colorectal cancers (stages II and III) with varying degrees of CIN [CIN-low (LOH ratio <33%) and CIN-high (LOH ratio ≥33%)] and compared to MSI tumors. Bar graphs display the results of such analysis as fold change in Daple mRNA (y axis) in CIN-low or CIN-high colorectal carcinomas compared to MSI tumors. (E) Downregulation of Daple mRNA in the primary tumor early during cancer progression prognosticates tumor recurrence/metastasis. High-throughput microarray data from GSE database (PMID: 22917480, GSE: 37,892) were analyzed for the levels of expression of Daple mRNA in 130 stage II MSS tumors without (No Mets) or with (Mets) tumor recurrence/metastatic progression. (F) Loss of copy number for CCDC88C (DAPLE gene) occurs at the late stages of adenoma-to-carcinoma progression. Array comparative genomic hybridization data from GSE database were analyzed for ccdc88c copy number variations (CNVs) in 41 progressed adenomas (i.e., adenomas that present a focus of cancer). Progressed adenomas were analyzed for CNVs relative toploidy level in the DNA in laser-microdissected adenoma and carcinoma fractions and compared to adjacent normal epithelial fractions as matched controls. (G) Cell-free mRNA transcripts of Daple are detected in patients with colorectal cancer, but not in normal control subjects. Microarray data from GSE database (PMID: 18843029, GSE: 10,715) were analyzed for Daple mRNA expression in peripheral blood samples of healthy subjects (n = 11) and of 121 patients with early (Dukes A, B) or late (Duke's C, D) stages of colorectal cancer. (H) Levels of Daple mRNA are frequently elevated in EpCAM (epithelial cell adhesion molecule) immunoisolated circulating tumor cells (CTCs) from patients with metastatic colorectal cancer, compared to normal subjects. Immunoisolated CTC fractions from the peripheral blood of 51 patients with metastatic (stage IV) colorectal cancer or from healthy subjects were analyzed for Daple mRNA by Taqman qPCR and adjusted for leukocyte contaminants by normalizing to CD45. Scatter-plots display the level of Daple expression in each patient within each group. A normality test confirmed that data sets in both groups were distributed normally. No significant differences were observed in the CD45 levels between two groups (not shown). (I, J) High levels of Daple mRNA expression in CTCs are associated with poorer progression-free (PFS; I) and overall (OS; J) survival in patients with metastatic colorectal carcinoma. Optimal cut-off values for Daple mRNA expression were statistically derived (see detailed ‘Materials and methods’) to generate subgroups of patients with high- or low-expression levels. Time-dependent survival probabilities were estimated with the Kaplan–Meier method, and the log-rank test was used to compare the subgroups. (K) Schematic summarizing profile of Daple expression during oncogenic progression in the colon. Degree of upregulation (green) or downregulation (red) in Daple mRNA is indicated by increasing shades of each color during the normal-to-adenoma-to-carcinoma progression in the colon is shown. (L) Proposed model for how a bimodal dysregulation of tumor suppressor Daple, and resultant deregulation of non-canonical Wnt signaling may propel oncogenic progression in the colon. Daple's ability to modulate G proteins via its GBA motif exerts a potent tumor suppressive effect in the normal mucosa. Early during oncogenesis (top, from left to right), downregulation of Daple (marked by ‘X’) occurs at the step of adenoma to cancer conversion, in part by DNA copy loss (bottom) due to focal deletion affecting the long arm of Chr 14. Consequently, low expression of Daple mRNA and protein triggers transformation and tumor growth/progression. Later during cancer invasion, expression of Daple is triggered via unknown mechanisms, which favors (green arrow) tumor recurrence and prognosticates poor survival.
-
Figure 8—source data 1
Meta-analysis of Daple mRNA expression in colorectal cancer vs matched normal controls.
The publicly available GSE database, a system to store, retrieve, and analyze all types of high-throughput microarray data was used to compare the levels of expression of Daple mRNA in colorectal cancer vs matched normal controls. From left to right, the columns indicate the GSE series ID, the PMID number for the respective source manuscripts, total samples analyzed in each study, fold change in Daple mRNA observed, and the significance (p-value) of any changes observed. A meta-analysis combining the p-values from these studies was analyzed by Fisher's method and displayed as bar graphs in Figure 8A.
- https://doi.org/10.7554/eLife.07091.019
-
Figure 8—source data 2
Meta-analysis of Daple mRNA expression in microsatellite unstable (MSI) vs stable (MSS) colorectal cancers.
The publicly available GSE database was used to compare the levels of expression of Daple mRNA in MSI vs MSS colorectal cancers. From left to right, the columns indicate the GSE series ID, the PMID number for the respective source manuscripts, total samples analyzed in each study, fold change in Daple mRNA observed, and the significance (p-value) of any changes observed. A meta-analysis combining the p-values from these studies was analyzed by Fisher's method and displayed as bar graphs in Figure 8C.
- https://doi.org/10.7554/eLife.07091.020
-
Figure 8—source data 3
Daple expression in CTCs correlates with markers of EMT.
Expression of Daple, ZEB2, and LOXL3 mRNA were analyzed in CTCs immunoisolated from 50 patients with metastatic colorectal cancer. An analysis of the Pearson's correlation coefficient for each pair of genes shows that higher expression of Daple is significantly associated with higher expression of ZEB2 and LOXL3, two genes implicated in triggering EMT.
- https://doi.org/10.7554/eLife.07091.021
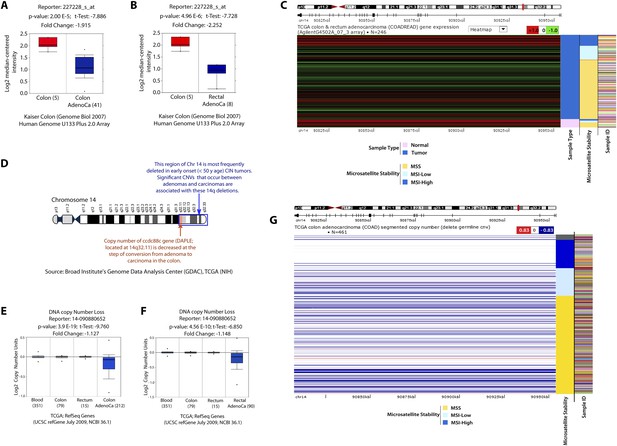
Expression of Daple mRNA is suppressed in colorectal cancers, in part by copy number loss.
(A, B) Publicly available Kaiser Colon database was analyzed for Daple mRNA expression in adenocarcinomas of the colon (A) and rectum (B) and their respective normal controls. Daple mRNA expression levels are displayed using log2 median-centered ratio boxplots for normal vs cancer that were generated using the UCSC Cancer Genome Browser. Numbers in parenthesis represent total number of samples analyzed. (C) The TCGA colon cancer database was analyzed for Daple mRNA expression in 246 colorectal adenocarcinomas. Daple mRNA expression levels are displayed as heat maps generated using the UCSC Cancer Genome Browser. Red = High Daple; green = Low Daple. Samples are arranged by sample type (normal vs cancer) and microsatellite status (MSI low or high vs MSS) as indicated on the right margin of the heat map. (D) Schematic of chromosome 14 is shown. Ccdc88c gene, which encodes Daple (red arow) is located within a frequently deleted region of Chr 14 (blue box). (E, F) Publicly available TCGA database was analyzed for number of copies of Daple gene in adenocarcinomas of the colon (E) and rectum (F) compared to matched normal mucosa and in blood cells. Copy number units of ccdc88c (Daple) in various matched samples are displayed using log2 median-centered ratio boxplots for that were generated using the UCSC Cancer Genome Browser. Numbers in parenthesis represent total number of samples analyzed. Compared to matched normal mucosa or peripheral blood, lower copy numbers of Daple gene were observed in adenocarcinomas of colon and rectum. (G) The TCGA colon cancer database was analyzed for the relationship between Daple copy number loss and microsatellite status in 461 tumor samples. Daple copy number in each tumor is displayed as heat map (blue = loss; red = gain) generated using the UCSC Cancer Genome Browser. Samples are arranged by microsatellite status (MSI low or high vs MSS) as indicated on the right margin of the heat map. A large majority of tumors had copy number loss (blue), but not gain (red). Tumors that had a loss of copy for the Daple gene (blue) are invariably MSS tumors, or MSI-low tumors. Copy number loss is virtually absent among MSI-high tumors.