A new family of StART domain proteins at membrane contact sites has a role in ER-PM sterol transport
Figures
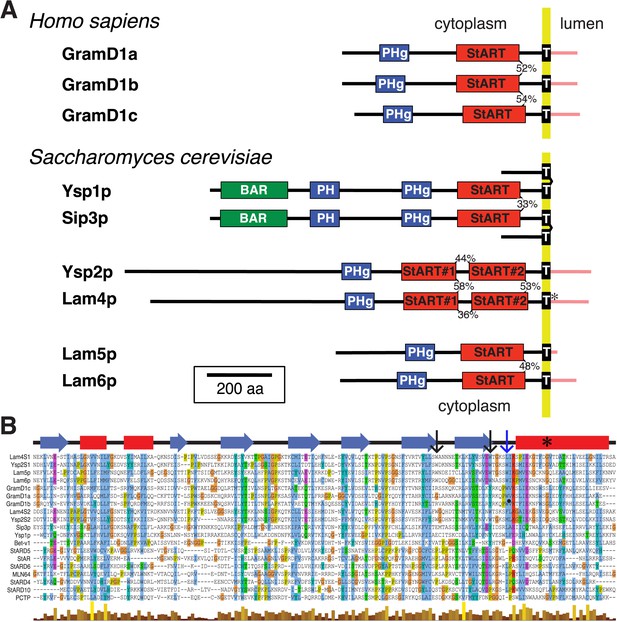
A new family of conserved lipid transfer proteins (LTPs) in the StART superfamily.
(A) StART-like domains are found in the predicted cytoplasmic domains of three human proteins and six yeast proteins, which are three pairs of paralogs. Proportions of identical residues in recently duplicated domains are indicated. Previous identifications were limited to GRAM domains in the pleckstrin homology superfamily (PHg) and transmembrane domains (T, in Lam4p the TMD is weakly predicted*). We identify Bin/amphiphysin/RVS (BAR), and other pleckstrin-homology (PH) superfamily domains. Predicted topology places most regions in the cytoplasm except short luminal regions (pink). Scale bar is 200 aa. (B) Alignment of yeast and human StART-like domains with Bet-v1 and seven human StARTs, with CLUSTALX coloring of conserved residues, together with secondary structure (above, sheets—blue arrows, helices—red) and quality of alignment (below). The C-terminal helix contains a glycine residue (*) predicted to interact with the omega-1 loop, hence affecting opening/closing of the lipid binding pocket. Arrows point to tryptophans present in Ysp2S1/2 and Lam4S1/2, black: in all 4 domains, blue: in Ysp2S1 only. A two residue insertion is omitted from the final loop of GramD1b.
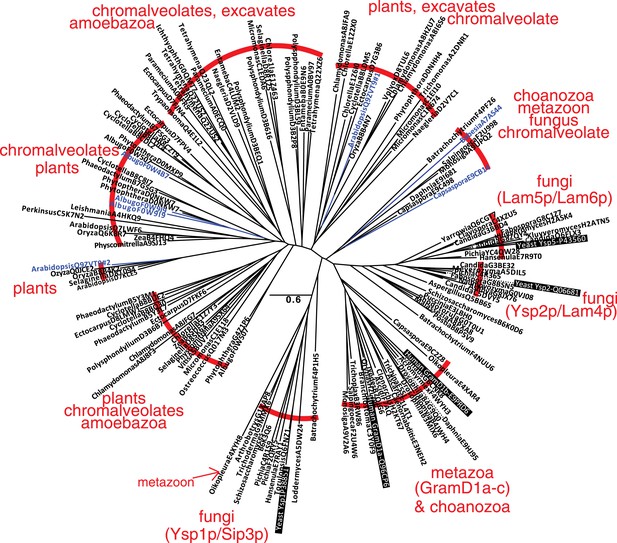
A family of StART-like domains in all eukaryotes.
An unrooted phylogenetic tree of 143 highly diverse of StART-like sequences was drawn by PHYML, showing species and Uniprot accession numbers. 10 sequence groups are bracketed to show the mixture of taxa in different parts of the tree. Four parts of the tree include yeast or human sequences (black background), showing that Ysp1p/Sip3p are more distant from Ysp2p/Lam4–6p than are human GramD1a-c. The 7 domains labelled in blue are shown in the context of their full-length proteins in Figure 1—figure supplement 2C.
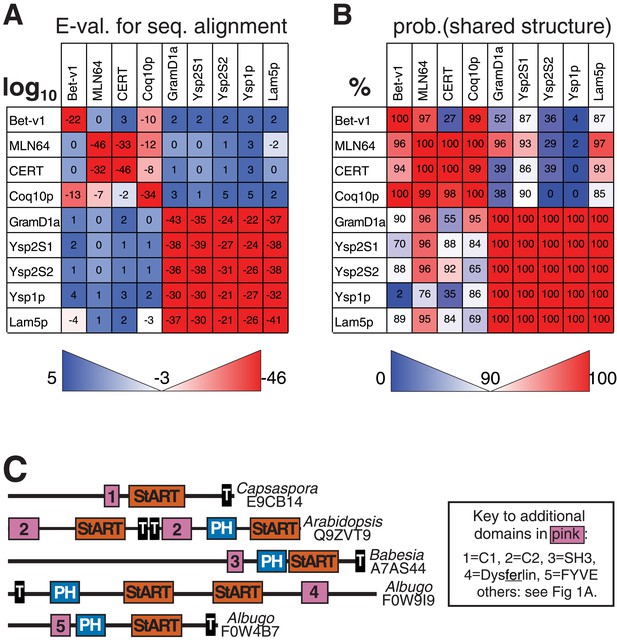
Relationships of StART-like domains.
(A) Each row shows E-values of sequence alignments obtained with the HMM alignment tool HHblits (Remmert et al., 2012). Each row was seeded with the sequence indicated on the left, and the strength of hit to each target is expressed as log10. (B) As A, but reporting HHpred results. Values indicate the probabilities of sharing the same structure (%). For both A and B squares are colored to indicate if the hit is above (red) or below (blue) the thresholds for significance: 90% in A, −3 (=log10, i.e., 0.001) in B. HHpred is more sensitive than HHblits, identifying many more links between known StART domains and new StART-like proteins. (C) Selected proteins with StART-like domains show a diverse range of accessory domains. Despite variation in the positioning of TMD(s), most of each protein is in the cytoplasm. Accessory domains (pink) are typically implicated in membrane targeting (see key).
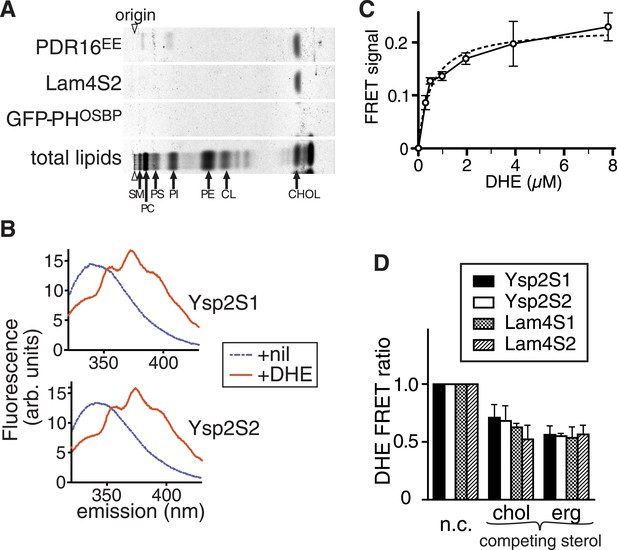
StART-like domains in Ysp2p and Lam4p specifically bind sterol.
(A) The second StART-like domain of Lam4p (Lam4S2) binds cholesterol. Human leukemic cells (HL60) in which all lipids were labelled with 14C-acetate were semi-permeabilized and incubated with bacterially expressed Lam4S2 and two control proteins: Pdr16(EE) which binds cholesterol (Holic et al., 2014) and GFP-PH-OSBP (negative control). Lipid extracts of re-isolated proteins were separated by TLC. Positions of major identifiable lipids were ascertained from total lipids (arrows, SM = sphingomyelin, PC = phosphatidylcholine, PI = phosphatidylinositol, PS = phosphatidylserine, PE = phosphatidylethanolamine, CL = cardiolipin). Arrowheads indicate origin. (B) FRET between Ysp2S1 or Ysp2S2 and dehydroergosterol (DHE). Tryptophan fluorescence (excitation at 295 nm) with purified protein either on its own or incubated with DHE. (C) Tryptophan-DHE FRET of Lam4S2 (1.05 µM) incubated with increasing concentrations of liposomes containing 30% DHE at the final concentrations indicated. The best fitting one saturable site binding curve (dashed line) indicates that Kd for binding = 0.5 µM DHE. (D) Effect on DHE FRET signal of adding non-fluorescent sterols (chol—cholesterol, erg—ergosterol) added at the same concentration as DHE and one of four StART-like domains from Ysp2p and Lam4p. ‘n.c.’ = no competitor, signal defined as 1; lipids added in methanol.
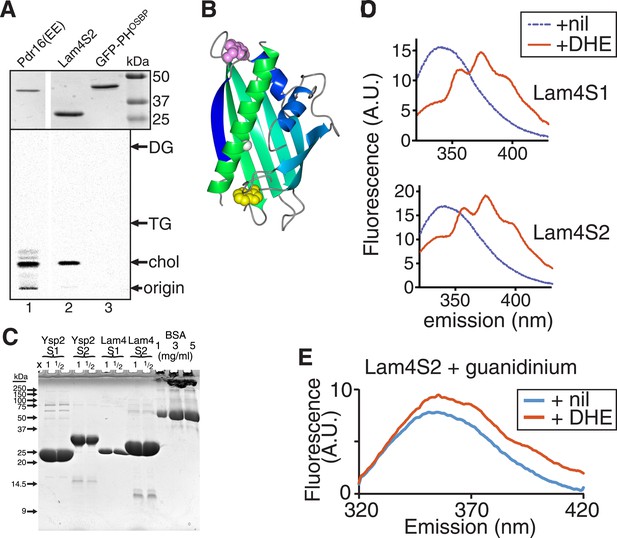
Lipid binding properties of StART-like domains.
(A) Lam4S2 binding to cellular lipids (see Figure 2A). TOP: His6-tagged proteins (1) Pdr16(EE) (Holic et al., 2014), (2) Lam4S2, (3) GFP-PHOSBP were re-isolated after incubation with radiolabelled cells, separated by SDS-PAGE and stained by Coomassie (MW markers indicated). BOTTOM: Bound lipids that ran near the solvent front in the first TLC (Figure 2A) were scraped and re-separated to distinguish between cholesterol and other neutral lipids triacyl- and diacyl-glycerol (TG and DG). (B) Lam4S2 (966–1136) was modelled using SAM-T08 (Karplus, 2009), colored in a spectrum from N-terminus (blue) to C-terminus (green). Also shown: two conserved tryptophans either inside (yellow) or outside (pink) the pocket, a conserved glycine in the C-terminal helix (white = G1205 in Ysp2p, G1119 in Lam4p). (C) Stock solution of BSA and aliquots of the indicated purified StART-like domains (x1 and x1/2 volume compared to BSA) were run on a 15% SDS-PAGE gel that was stained by Coomassie. (D) FRET between Lam4S1 or Lam4S2 and DHE. Tryptophan fluorescence (excitation at 295 nm) with purified protein either on its own or incubated with DHE. (E) Tryptophan emission spectrum of Lam4S2 with and without DHE (as in panel C) but in the presence of 7 M guanidinium to denature the protein. This shows that DHE is only slightly fluorescent when stimulated at 295 nm.
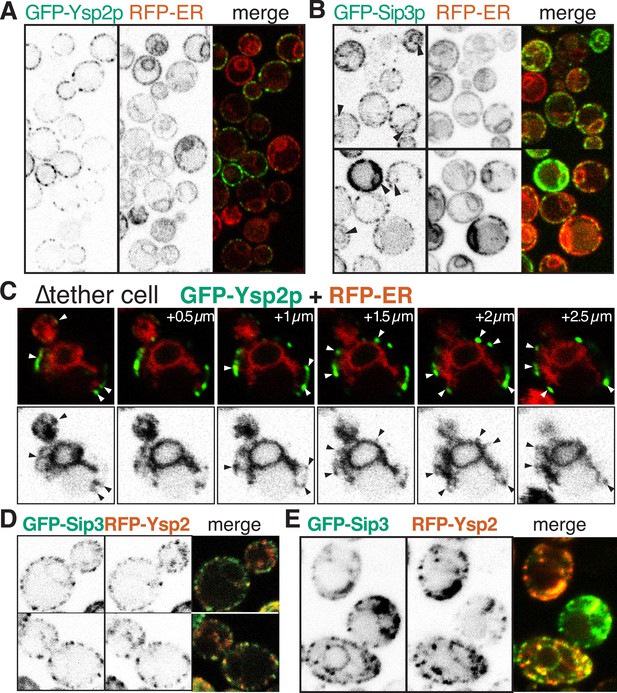
GFP-Ysp2p and Sip3 at ER-PM contacts in wildtype and ∆tether cells.
(A and B) GFP-Ysp2p and GFP-Sip3p (PHO5 promoter) in cells co-expressing RFP-ER, showing separate channels (inverted grey-scale) and merges. Arrowheads indicate nuclear envelopes containing GFP-Sip3p. (C) Confocal sections of a ∆tether cell expressing GFP-Ysp2p and RFP-ER (top), with high contrast inverted grey-scale images of RFP-ER (bottom) to visualize endoplasmic reticulum (ER) strands extending to Ysp2p-positive peripheral puncta (arrowheads). (D) Cells co-expressing GFP-Sip3p and RFP-Ysp2 at low levels, with separate channels as inverted grey-scale images and the merge, showing colocalization in most puncta. (E) As D, but showing cells with high levels of GFP-Sip3p, which accumulates internally with delocalized internal RFP-Ysp2p.
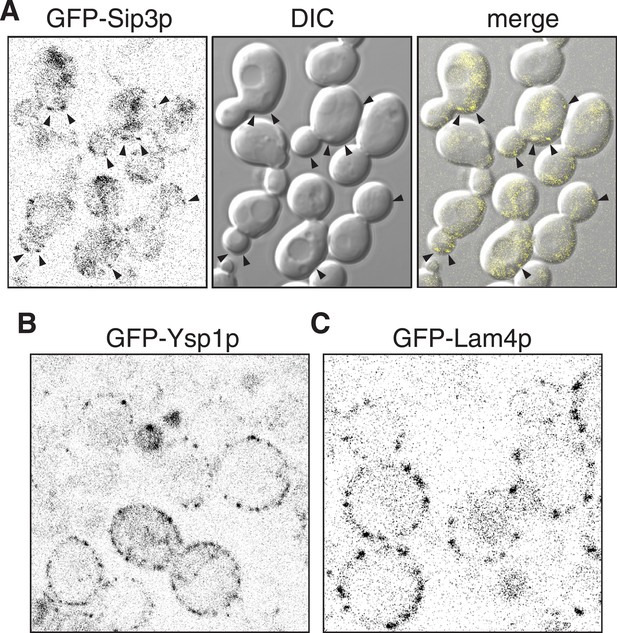
Sip3p Ysp1p and Lam4p expressed from their own promoters.
(A, B, C): Cells expressing plasmid borne N-terminally GFP-Sip3p, GFP-Ysp1p and GFP-Lam4p with their own promoters (inverted grey-scale). In A, Sip3p puncta are highlighted by arrowheads and also shown superimposed on DIC images of the same cells.
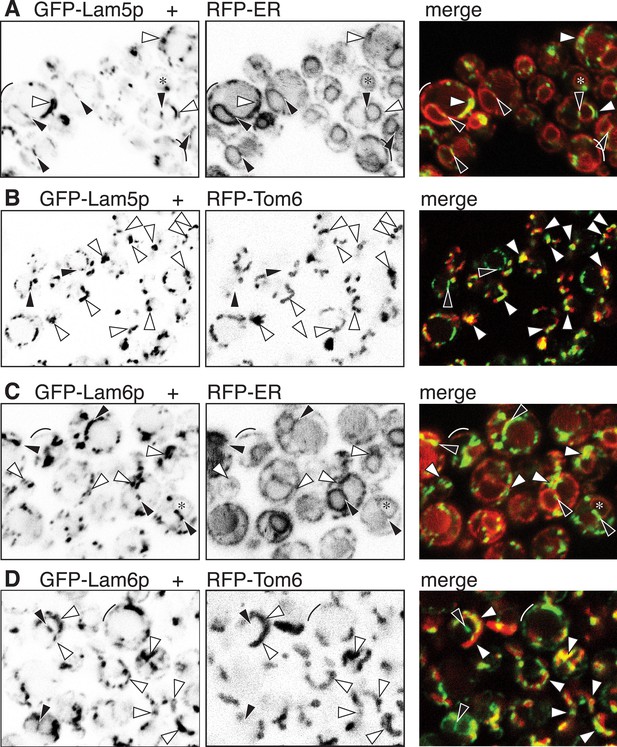
GFP-Lam5p and -Lam6p target the NVJ and ER-mitochondrial contact sites.
(A–D): LEFT panels: GFP-Lam5p (A and B) and GFP-Lam6p (C and D) in cells co-expressing MIDDLE panels: RFP-ER (A and C) or RFP-mitochondrial outer membrane (Tom6p) (B and D). RIGHT = merged images. Targeting by either GFP-tagged protein is indicated: NVJ (black filled arrowheads); mitochondrial outer membrane, sometimes seen as parallel lines (white filled arrowheads); generic ER (faint): nuclear envelope (asterisks), periphery (arcs).
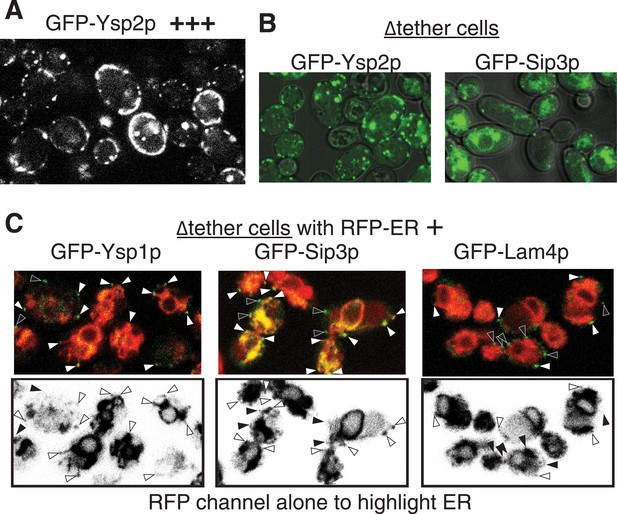
Ysp2p and its homologs target ER-PM contacts.
(A) GFP-Ysp2p expressed from the GAL1 promoter after induction in galactose for 8 hr. The distribution is largely unaffected by massive overexpression. (B) ∆tether cells expressing GFP-Ysp2p and GFP-Sip3p including the DIC channel to show where puncta are at cell peripheries. (C) ∆tether cells expressing RFP-ER and GFP-tagged Ysp1p/Sip3p/Lam4p. UPPER panels: colored merges; LOWER panels: inverted grey-scale images of RFP channel to indicate the extent of the ER. Arrows indicate all GFP +ve peripheral puncta, white if there is coincident RFP-ER, or black if not. ER was seen in ∼70% of GFP puncta, which underestimates the degree of colocalization as GFP puncta are seen in more sections than the faint ER strands (see Figure 3C).
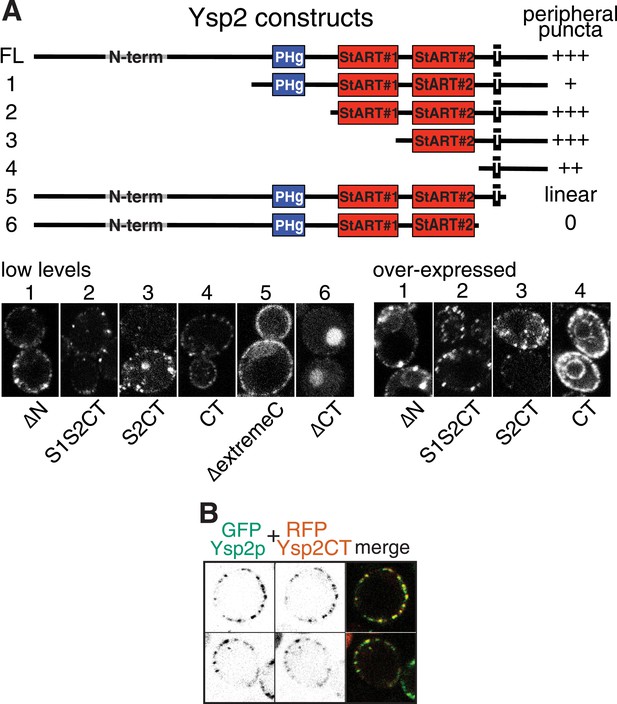
Analysis of punctate targeting by Ysp2p.
(A) Cells expressing the six indicated Ysp2 constructs, all with N-terminal GFP. TOP: diagram of constructs and degree of targeting to peripheral puncta. Domains are identified as in Figure 1A, along with the N-terminus (residues 1–608), which is predicted to be unstructured. BOTTOM: LEFT: low level expression. Construct 6 (∆CT) lacks the TMD and is diffuse in the cytoplasm and nucleus. RIGHT high level expression of constructs 1–4. Ysp2-carboxy terminus (CT = 194 aa, incl. TMD and luminal domain) is sufficient for targeting to peripheral puncta, but the construct is in general ER (incl. nuclear envelope) when highly expressed. (B) Co-expression of GFP-Ysp2p with RFP-Ysp2CT, with separate channels (grey scale) and merge showing almost 100% colocalization.
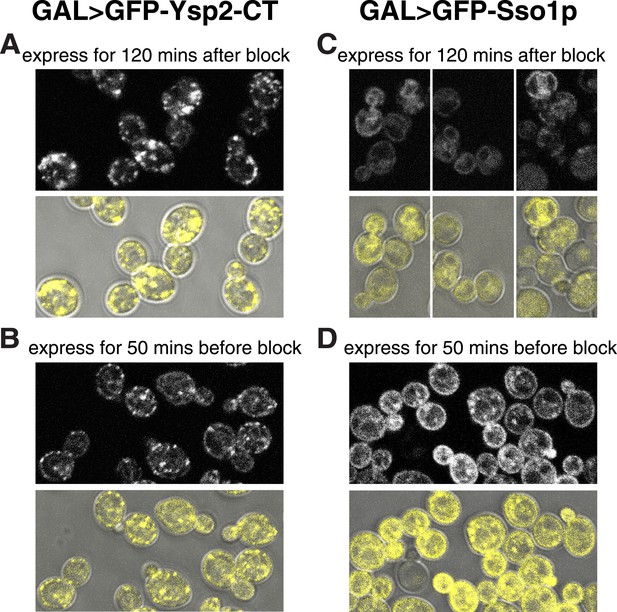
Ysp2CT targets peripheral puncta after imposition of sec18-1 blockade to SNARE-mediated transport.
(A and B) sec18-1 cells (RSY271) expressing GFP-Ysp2CT from the GAL1 promoter. (A): growth at 37°C for 10 min, then 120 min induction (addition 1% galactose); (B): induction for 50 min with 1% galactose at the permissive temperature (25°C), then cells were washed into dextrose containing growth medium (to stop transcription) and grown for 10 min further at 25°C before temperature shift to 37°C for 60 min. (C and D) As A and B respectively, except expressing GFP-Sso1p. In all panels, the lower images show GFP falsely coloured yellow superimposed on DIC images of cells to indicate the relationship between puncta and the cell periphery. GFP-Ysp2CT reached peripheral puncta when expressed after the temperature shift blocked secretion (A), and its expression levels were lower when the induction period was shorter (B). In contrast GFP-Sso1p was found in the ER (including nuclear envelope and cortical patches) when expressed after blocking Sec18-mediated secretion (C), but otherwise reached the plasma membrane (with some punctate internal staining) and was expressed at higher levels despite the shorter induction (D), indicating that Sso1p destabilized by the sec18-1 block.
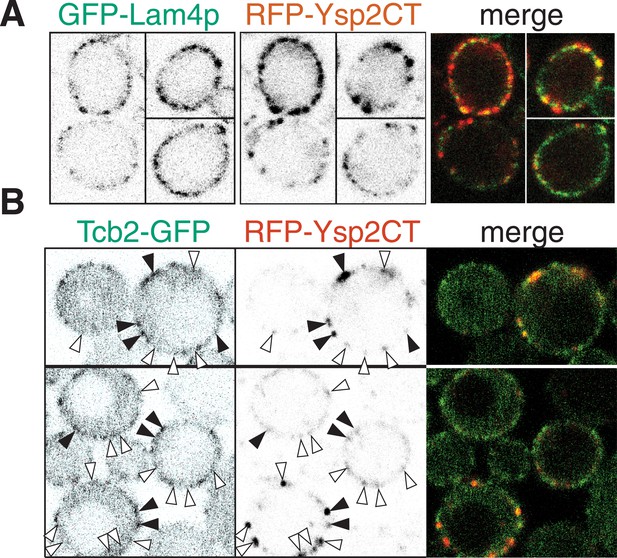
Ysp2 colocalizes partially with Lam4p but not significantly with Tcb2p.
(A) Co-expression of GFP-Lam4p with RFP-Ysp2CT, with separate channels as inverted grey-scale images, and the merge. ∼50% of puncta with Ysp2CT contained Lam4p and vice versa, indicating partial but significant overlap. (B) Wild-type cells co-expressing Tcb2-GFP and RFP-Lam2CT, shown as single channels (inverted grey-scale) and merge. Lam2CT + ve puncta (middle panels) are marked with arrowheads: filled if Tcb2-GFP is also enriched at that site, otherwise empty. ∼33% of Ysp2CT puncta also contained Tcb2-GFP. The proportion of the periphery overall that contained Tcb2-GFP was ≥25%, so the co-localization between the two proteins was not significant.
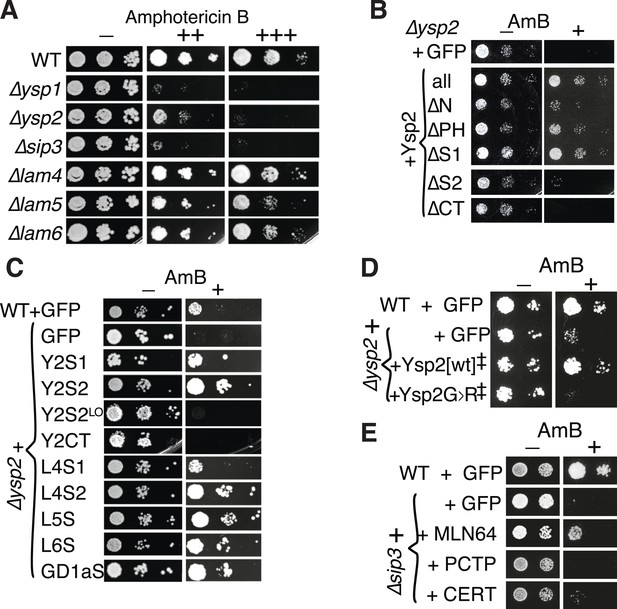
Amphotericin B (AmBS) phenotypes and rescue by StART-like domains that bind sterol.
(A) Dilutions of cells with single gene deletions were compared with the wild-type parental strain (WT) for ability to grow at two concentrations (moderate and high) of AmB, with AmB = 0 to control for cell number. (B) Effect of AmBS growth by ∆ysp2 cells from GFP-Ysp2p under its own promoter with individual domains deleted. (C) Rescue of ∆ysp2 by domains of Ysp2p (S1/S2/CT), Lam4S1/S2, and StART-like domains of Lam5p Lam6p and human GramD1a. All plasmids had the PHO5 promoter for moderately high expression, except Y2S2LO had the weakly expressing YSP2 promoter. (D) Growth by ∆ysp2 cells expressing Ysp2p or the G > R mutant under the YSP2 promoter ± AmB. ‡ indicates that Ysp2S2 in both plasmids was substituted by Lam4S2 (53% identical, 72% homologous). (E) Growth ± AmB by ∆sip3 cells overexpressing human LTP domains of MLN64, PCTP and ceramide transfer protein (CERT) (TPI1 promoter).
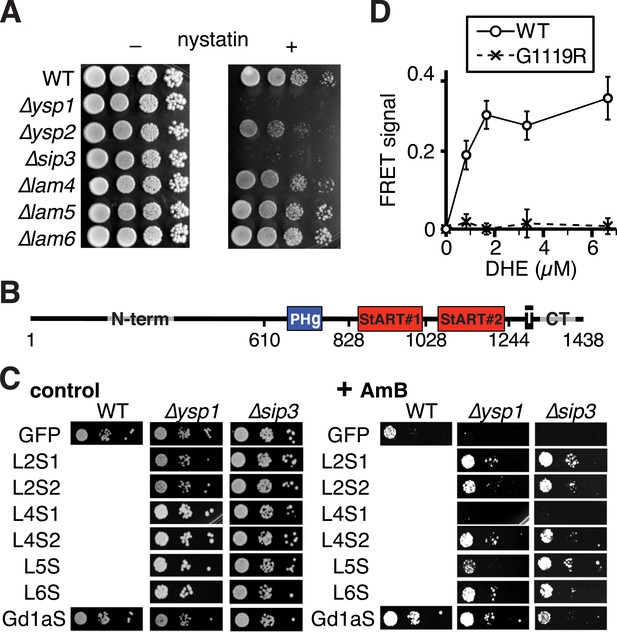
Activities of different StART-like domains.
(A) Nystatin sensitivities of WT, and single deletions in ∆ysp1/∆ysp2/∆sip3/∆lam4—6 grown with and without nystatin (30 µg/ml). (B) Diagram of domain boundaries in Ysp2 constructs used in Figure 4B,C. (C) AmB sensitivities of WT, ∆ysp1 and ∆sip3 cells with plasmids expressing GFP alone or GFP tagged to seven StART-like domains (Lam2S1, Lam2S2, Lam4S1, Lam4S2, domains from Lam5, Lam6, and human GramD1a). LEFT: control plates to show cell number; RIGHT: plates with AmB 125 ng/ml. All domains were active except Lam4S1, which was less active in ∆ysp2. (D) Tryptophan-DHE FRET with constant concentration of wild-type or mutant (G1119R) Lam4S2 (1.05 µM) incubated with increasing concentrations of liposomes containing 30% DHE.
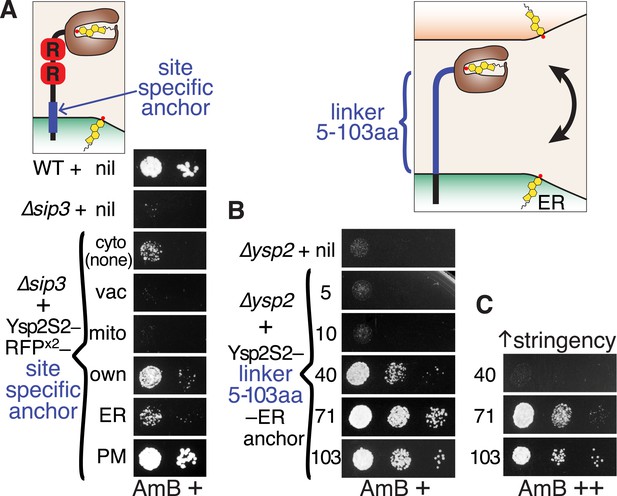
Ysp2S2 is activated by anchoring that allows crossing of ER-PM contacts.
(A) Anchoring at different locations. AmBs growth of ∆sip3 cells transformed with plasmids weakly expressing (YSP2 promoter) Ysp2S2-RFP(dimeric) followed either by nothing (cytoplasmic) or by anchors for specific sites: vacuole (Nyv1p, all), mitochondria (Tom6p, all), own anchor (Ysp2 1249–1438), generic ER (Scs2: 220–244), and plasma membrane (PM) (Sso1p, all). WT and ∆sip3 cells carrying empty plasmids were included as controls. (B and C) Varying the length of the linker. AmBs growth of ∆ysp2 cells carrying an empty plasmid or weakly expressing (YSP2 promoter) Ysp2S2 anchored to the ER by the TMD of Scs2 (residues 226–244) with intervening linkers of 5, 10, 40, 71 or 103 residues. Cells in B were grown on plates with moderate AmB (63 ng/ml AmB). In C, cells with 40, 71 and 103 aa linker constructs were grown at higher stringency (125 ng/ml AmB). Diagrams indicate the variable sites for each experiment in blue. Equal cells were plated, as shown on control plates (Figure 5—figure supplement 1).
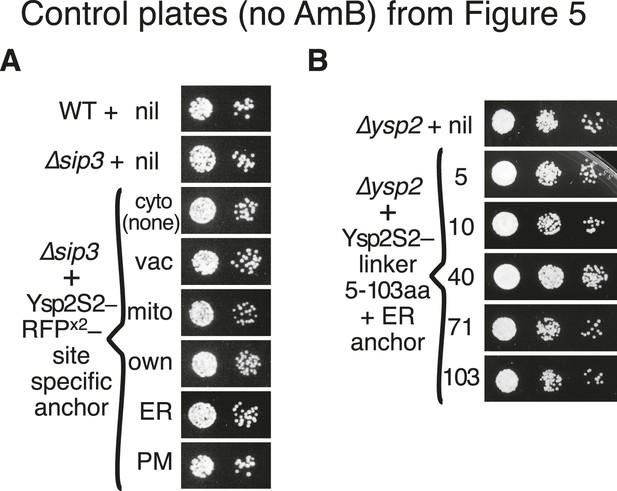
Controls for tests of LAM domain function at ER-PM contacts.
(A) and (B): Cells from parts A and B of Figure 5 were also plated on control plates (no AmB) to indicate whether the cell number was equal throughout.
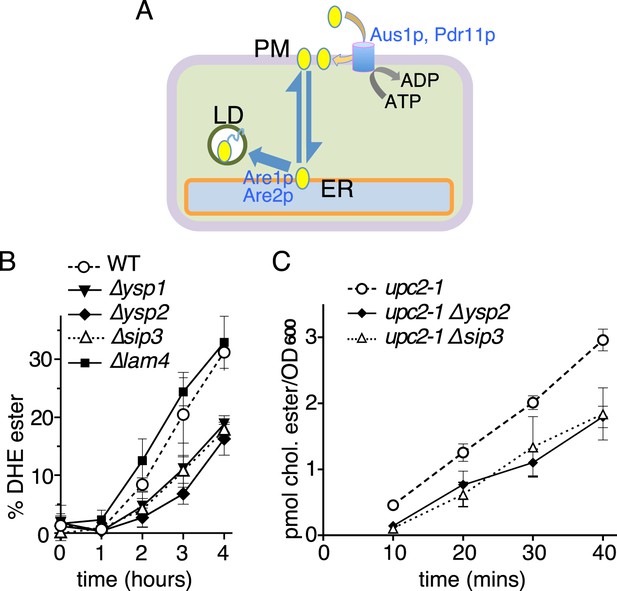
Retrograde sterol traffic is slower in strains lacking Ysp1p Ysp2p or Sip3p.
(A) Diagram of retrograde traffic pathway for exogenous sterols. Apart from the hypothesized sterol transfer protein (double headed arrow), other steps include insertion into the PM by ABC transporters Aus1p and Pdr11p, and esterification in the ER by ACAT enzymes Are1p and Are2p prior to storage in lipid droplets (LD). (B) Retrograde traffic of DHE in four single delete strains of the yeast StART-like family were compared to wildtype controls. DHE ester formation was followed by HPLC during redistribution of DHE away from the PM. (C) Retrograde traffic of cholesterol in wildtype and two delete strains (∆ysp2 and ∆sip3) as determined from esterification of cholesterol added exogenously. Strains were created in a WPY361 (upc2-1) background to allow cholesterol uptake.
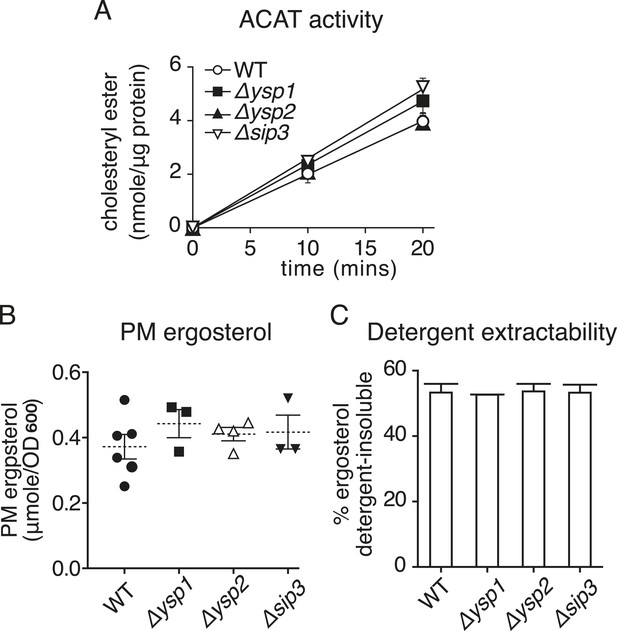
Strains lacking Ysp1p Ysp2p or Sip3p have no significant changes in sterol handling.
(A) ACAT activity producing sterol esters was measured in microsomes prepared from cell homogenates of the indicated strains over a 20 min period. Activity was marginally increased in ∆ysp1 and ∆sip3 cells. (B) Ergosterol was measured in PM fractions obtained by sucrose gradient centrifugation of lysates from the indicated strains. PM ergosterol was not significantly changed in ∆ysp1, ∆ysp2 and ∆sip3 strains compared to wild-type. (C) The proportion of total cellular sterol that partitions into detergent–insoluble complexes was measured. This was unaltered in ∆ysp1, ∆ysp2 and ∆sip3 strains compared with wild-type cells.
Tables
Plasmids used in this study
Bacterial expression | Constructs all start: MGGSHHHHHHGMASHHHHHARA |
His-Ysp2S1 | + PVMT + Ysp2 829-1027 + R |
His-Ysp2S2 | + PVMT + Ysp2 1027-1244 + R |
His-Lam4S1 | + M + Lam4 731-938 + DV |
His-Lam4S2 | + M + Lam4 946-1155 + DV |
His-Lam4S2(G > R) | as His-Lam4S2, G1119 > R |
Yeast expression | unless stated pRS series, 405 = integrating LEU2, 406 = integrating URA3, 316 and 416 = CEN URA3; unless stated PHO5 promoter {168} |
GFP-Ysp2p | 416: GFP + Ydr326c/Ysp2p ORF [1438], with 2 extra residues to facilitate cloning in five sections: K828 > KT and S1244 > SR |
GFP-Ysp1p/Sip3p/Lam4p | 416: GFP + whole ORFs: Yhr155w/Ysp1p [1228], Ynl257c/Sip3p [1229], Yhr080cp/Lam4p [1348] |
GFP-Lam5/6p* | 406: GFP + whole ORFs: Yfl042cp/Lam5p M1 > S [674], Ylr072wp/Lam6p M1 > S [693] |
GFP-Ysp1p/Ysp2p/Sip3p/Lam4p: own promoters | as above for GFP-ORF, except replace PHO5 promoter with: YSP1 {951}, YSP2 {459}, SIP3 {360}, LAM4 {701} |
RFP-ER | 405: dimeric dsRed tdimer2(12) [464] + RNSKP (linker) + ENESSS•MGIFILVALLILVLGWFY•R = Scs2p 220–244 = linker[6] + TMD[18] + lumen[1] |
RFP-Tom6p | as RFP-ER, but after RNSKP: G + Tom6p [61] |
RFP-Ysp2p | 406: dimeric dsRed tdimer2(12) [464] + Ysp2 K828 > KT and S1244 > SR |
GAL > GFP-Ysp2p | as for GFP-Ysp2p, except 406, and replace PHO5 promoter with GAL1 {807} |
GFP-Ysp2∆N | 416 YSP2 prom.: GFP + Ysp2 611-1438 |
GFP-Ysp2∆PH | 416 YSP2 prom.: GFP + Ysp2 1-610 + 829-1438 |
GFP-Ysp2∆S1 | 416 YSP2 prom.: GFP + Ysp2 1-828 + T + 1028-1438 |
GFP-Ysp2∆S2 | 416 YSP2 prom.: GFP + Ysp2 1-1027 + R + 1245-1438 |
GFP-Ysp2S1S2CT | 416 YSP2 prom.: GFP + Ysp2 829-1438 |
GFP-Ysp2S2CT | 416 YSP2 prom.: GFP + Ysp2 1028-1438 |
GFP-Ysp2CT | 416 YSP2 prom.: GFP + Ysp2 1245-1438 |
GFP-Ysp2∆extremeC | 416 YSP2 prom.: GFP + Ysp2 1-1319 |
GFP-Ysp2∆CT | 416 YSP2 prom.: GFP + Ysp2 1-1246 |
RFP-Ysp2CT | as RFP-ER, but after RNSK: LGSAPVMSR + Ysp2 1245-1438 |
GFP only | 416: GFP + GFP |
GFP-Ysp2S1 | 416: GFP + Ysp2 829-1028 |
GFP-Ysp2S2 | 416: GFP + Ysp2 1027-1244 + R |
GFP-Lam4S1 | 416: GFP + Ysp2 759-929 |
GFP-Lam4S2 | 416: GFP + Lam4 968-1140 |
GFP-Lam5S | 416: GFP + Lam5 381-586 + AS |
GFP-Lam6S | 416: GFP + myc tag + Lam6 374-582 + DV |
GFP-GramD1aS | 416: GFP + GramD1a 359-547 + DV |
GFP-Ysp2S2sw4 | 416 YSP2 prom.: GFP + Ysp2 1-1044 + Lam4 S953-V1161 + SR + Ysp2 1245-1438 |
GFP-Ysp2S2sw4G > R | as GFP-Ysp2S2sw4 G1119 > R |
GFP-StART-MLN64 | 406: TPI1 prom. {412}: GFP + MLN64 216-445 |
GFP-StART-PCTP | 406: TPI1 prom.: GFP + PCTP [214] |
GFP-StART-CERT | 406: TPI1 prom.: GFP + CERTl 397-624 |
Ysp2S2-RFP | 405 YSP2 prom.: M + Ysp2 1022-1244 + 40 aa linker + RFP + 27 aa linker + RFP + HLFLRNSK + final 8aa: LGSQSMFD |
Ysp2S2-RFP-Vacuole | as Ysp2S2-RFP; final 8 aa → PGASYQ + Nyv1p [253] |
Ysp2S2-RFP-Mito | as Ysp2S2-RFP; final 8 aa → PG + Tom6p [61] |
Ysp2S2-RFP-own CT | as Ysp2S2-RFP; final 8 aa → LGSAPVMSR + Ysp2 1245-1438 |
Ysp2S2-RFP-ER | as Ysp2S2-RFP; final 8 aa → P + Scs2p 220-244 (see RFP-ER, above) |
Ysp2S2-RFP-PM | as Ysp2S2-RFP; final 8 aa → PGAS + Sso1p [290] |
Ysp2S2-5aa-ER | 405 YSP2 prom.: M + Ysp2 1057-1224 + linker SRLE + Scs2 225-244 = linker [1] + TMD[18] + lumen [1] (S•MGIFILVALLILVLGWFY•R). Total linker = 5 aa |
Ysp2S2-10aa-ER | as Ysp2S2-5aa-ER; after SRLE insert extra 5 aa: Scs2 220-224 |
Ysp2S2-40aa-ER | as Ysp2S2-5aa-ER; after SRLE insert extra 35 aa: Scs2 190-224 |
Ysp2S2-71aa-ER | as Ysp2S2-5aa-ER; after SRLE insert extra 66 aa: Scs2 159-224 |
Ysp2S2-103aa-ER | as Ysp2S2-5aa-ER; after SRLE insert extra 98 aa: KL + Scs2 129-224 |
Pdr11p-GFP† | pWP1251. 316: PDR11 prom.: Pdr11p-GFP |
Aus1p-GFP† | pWP1220. 316: AUS1 prom.: Aus1p-GFP |
-
Cloned regions are from S. cerevisiae (S288c) or human I.M.A.G.E. clones; size of whole proteins/promoters are in brackets, for protein [aa] and DNA {bp}; promoters are regions of genome just prior to open reading frame starts; ranges within proteins are not in brackets; changes in natural residues are written as ‘X123 > Z’; specific amino acid sequences (e.g., linkers) are underlined.
-
Plasmids were received as kind gifts from
-
*
Sean Munro.
-
†
Will Prinz.
Strains used in this study
Strain | Genotype | Reference |
---|---|---|
BY4741 | (Euroscarf) MATa his3Δ1 leu2Δ0 met15Δ0 ura3Δ0 | Euroscarf |
∆ysp1 | BY4741 YHR155W::KANMX4 | Euroscarf |
∆ysp2 | BY4741 YDR326C::KANMX4 | Euroscarf |
∆sip3 | BY4741 YNL257C::KANMX4 | Euroscarf |
∆lam4 | BY4741 YHR080C::KANMX4 | Euroscarf |
∆lam5 | BY4741 YFL042C::KANMX4 | Euroscarf |
∆lam6 | BY4741 YLR072W::KANMX4 | Euroscarf |
WPY361 (upc2-1) | MATa upc2-1 ura3-1 his3-11,-15 leu2-3,-112 trp1-1 | (Li and Prinz, 2004) |
Upc2-1 ∆ysp2 | WPY361 (upc2-1) YDR326C::KanMX4 | This study |
Upc2-1 ∆sip3 | WPY361 (upc2-1) YNL257C::HYGROR | This study |
RS453C | MATα ade2-1 his3-11,15 ura3-52 leu2-3112 trp1-1 | (Levine and Munro, 2001) |
∆ysp1 | RS453C YHR155W::HIS5 S.p. | This study |
∆ysp2 | RS453C YDR326C::HIS5 S.p. | This study |
∆sip3 | RS453C YNL257C::HIS5 S.p. | This study |
∆lam4 | RS453C YHR080C::HIS5 S.p. | This study |
∆lam5 | RS453C YFL042C::HIS5 S.p. | This study |
∆lam6 | RS453C YLR072W::HIS5 S.p. | This study |
Tcb2-GFP | RS453C TCB2-EGFP::HIS5 S.p. | This study |
SEY6210 | MATa leu2-3,-112 ura3-52 his3Δ200 trp1Δ901 lys2-801 suc2-Δ9 GAL | (Manford et al., 2012) |
ANDY198 (Δtether) * | SEY6210 ist2Δ::HISMX6 scs2Δ::TRP1 scs22Δ::HISMX6 tcb1Δ::KANMX4 tcb2Δ::KANMX46 tcb3Δ::HISMX6 | |
RSY271 sec18-1† | MATa sec18-1 ura3-52 his4-619 | (Novick et al., 1980) |
Lnp1-GFP‡ | SFNY 2092, MAT a, ura3-52, leu2-3,112, his3Δ200, LNP1-3xGFP::URA3 | (Chen et al., 2012) |
-
Kind gifts of strains:
-
*
Chris Stefan.
-
†
Mike Lewis.
-
‡
Susan Ferro-Novick.