Recombinational branch migration by the RadA/Sms paralog of RecA in Escherichia coli
Figures
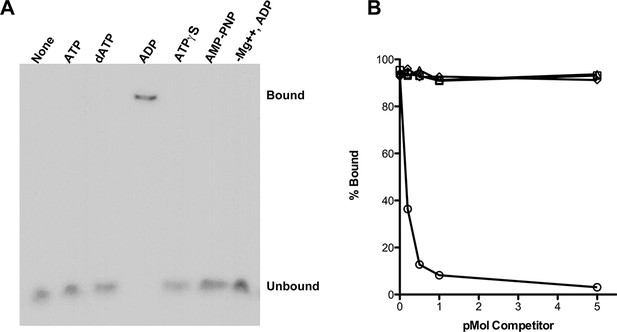
RadA Binding to DNA.
(A) Nucleotide dependence of RadA binding to poly d(T)30. Reactions (10 µl) contained 100 fmol (molecule) of radio-labeled poly d(T)30, 3.3 pmol RadA and 1 mM nucleotide. After incubation at 37 °C for 20 min, binding was assessed using EMSA. (B) DNA substrate specificity of RadA Binding. Reactions containing 1 mM ADP, 3.3 pmol RadA, 100 fmol (molecule) of radio-labeled poly d(T)30 and unlabeled competitor DNA (circles-poly d(T)30, triangles- poly d(C)30, diamonds-poly d(G)30, squares poly d(A)33 were incubated at 37° for 20 min. The extent of binding was determined using scanned autoradiographs of EMSA gels processed with Image J-64.
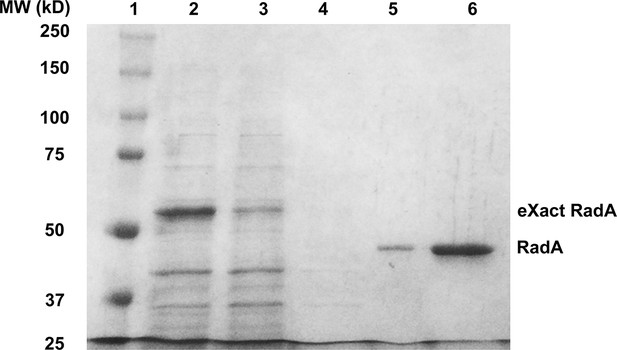
Purification of RadA.
1. SDS PAGE Gel Showing Purification of RadA. Lane 1) MW Markers; Lane 2) Crude Lysate; Lane 3) Flowthrough; Lane 4) Wash; Lane 5) eXact peak fraction; Lane 6) MonoQ HP peak fraction.
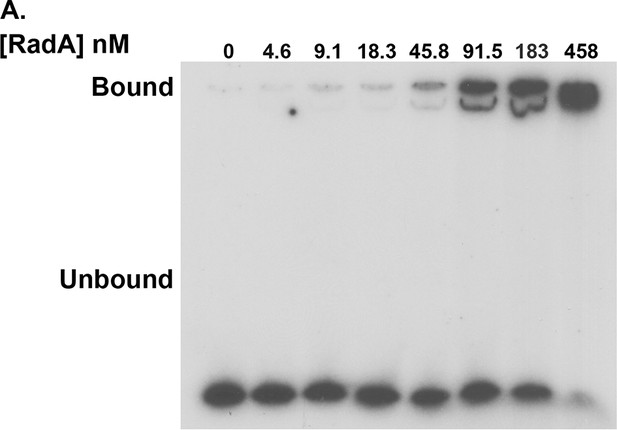
RadA binding to poly(dT)30.
Electrophoretic mobility shift assay for RadA binding to poly(dT)30, with RadA concentrations indicated.
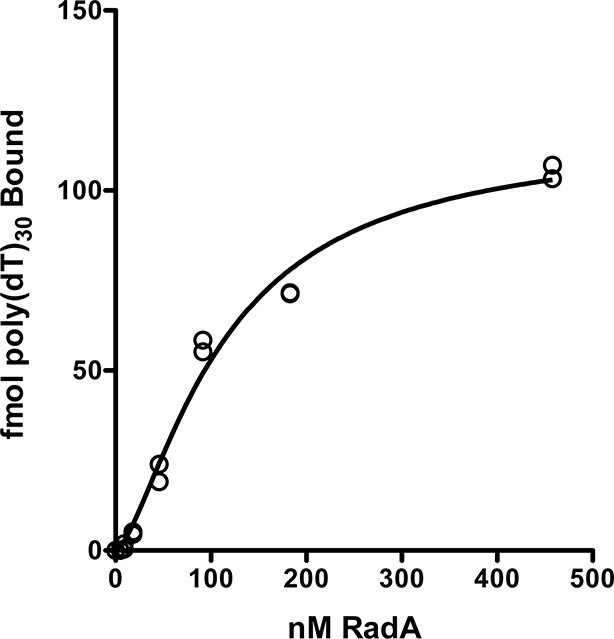
RadA binding curve to poly(dT)30.
The autoradiographs from Figure 1—figure supplement 2 and a second gel were scanned and binding was quantitated using Image J 64. GraphPad Prism software was used to fit the data to a binding curve. The data from two replicates are shown. The apparent Kd derived from this curve is 110 nM ± 14 nM.
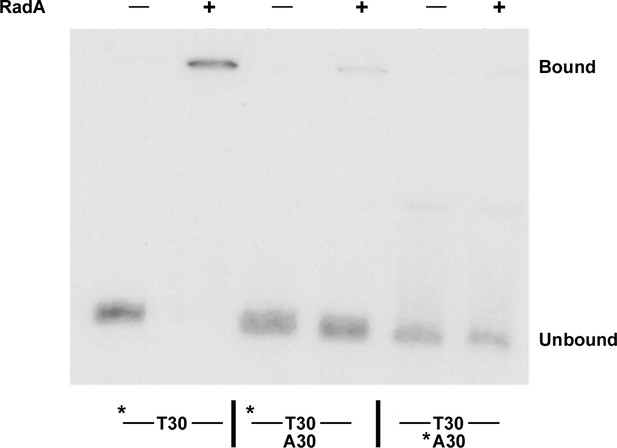
Binding of RadA to substrate E2.
Electrophoretic mobility shift assay for RadA binding. Substrate E2 has approximately 30 nucleotides on either side of a poly(dT) 30 core. Poly(dA) 30 was annealed to E2 before the binding reaction was initiated for the samples indicated. The * indicates the strand labeled with 32P.
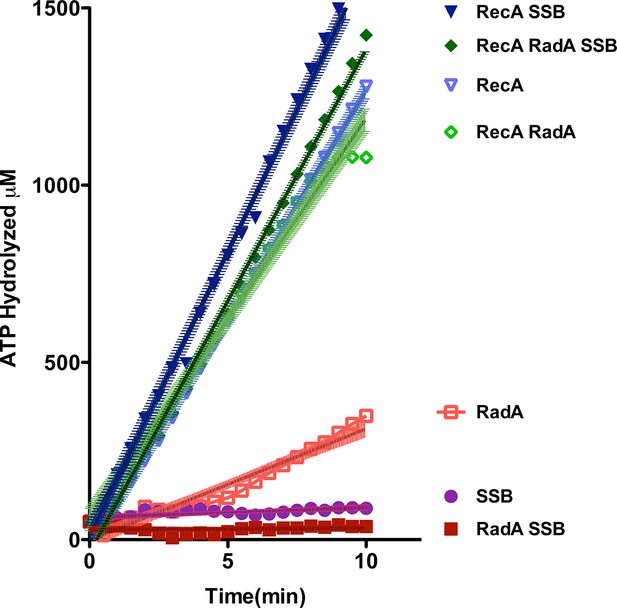
ATP hydrolysis in reactions including RecA, SSB and RadA.
ATP hydrolysis was measured in reactions containing DNA and protein concentrations similar to those in recombination reactions and included 21 µM (nucleotide) single-strand circular DNA and 6.7 µM RecA, 1.9 µM SSB, and 630 nM RadA. RecA and/or RadA were pre-incubated with the single-strand DNA for 8 min at 37 °C before the reactions were initiated with ATP +/- SSB. Rate measurement started 5 min after the addition of ATP. Reactions included: SSB (closed purple circles), RadA (open red squares), RadA +SSB (closed dark red squares), RecA alone (blue inverted triangles), RecA+SSB (closed dark blue inverted triangles), RadA + RecA (open green diamonds), RadA+RecA+SSB (closed dark green diamonds). Error bars represent the 95% confidence interval of the linear fit of the data calculated using Prism Graph Pad.
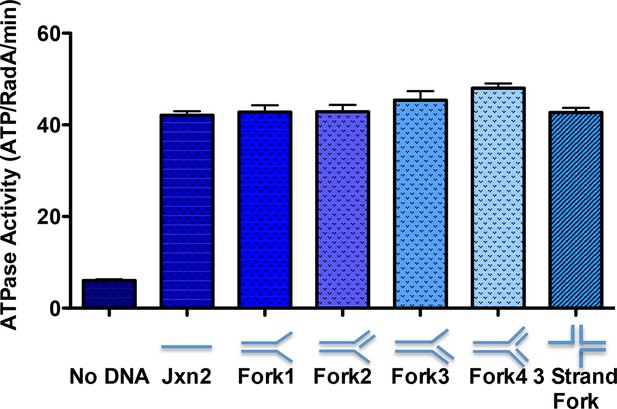
ATPase activity on model oligonucleotide substrates.
NADH coupled ATP hydrolysis assays were performed as described in the procedures. Reactions contained 0.3 μM (molecule) model forks and 0.63 μM RadA. Average rates from three experiments are shown. Error bars represent standard deviations. The sequences for the oligonucleotides used to make the forks are in Table 2.
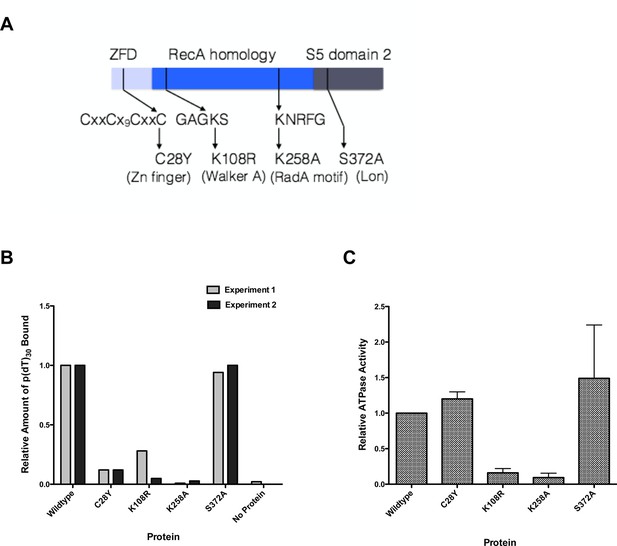
Properties of RadA Domain Mutants.
(A) Schematic of RadA Domains and Locations of Domain Mutants. (B) Binding of RadA Mutants to poly d(T)30. Reactions contained 100 fmol (molecule) of poly d(T)30, 3.3 pmol (mutant) RadA and 1 mM ADP. After incubation at 37 °C for 20 min, binding was assessed using EMSA. Binding relative to wild-type RadA is shown for two independent experiments. (C) ATP hydrolysis by RadA domain mutants. ATP hydrolysis was assessed using PEI TLC to visualize release of inorganic phosphate (Pi) as described in the procedures. Reactions contained either no or 10.5 µM (nucleotide) single-strand circular M13 DNA and 250 nM RadA or RadA mutant protein. Graph shows the mean ATP hydrolysis activity of RadA mutants from three independent experiments relative to the activity of wild-type RadA. Error bars represent the standard deviation of the mean.
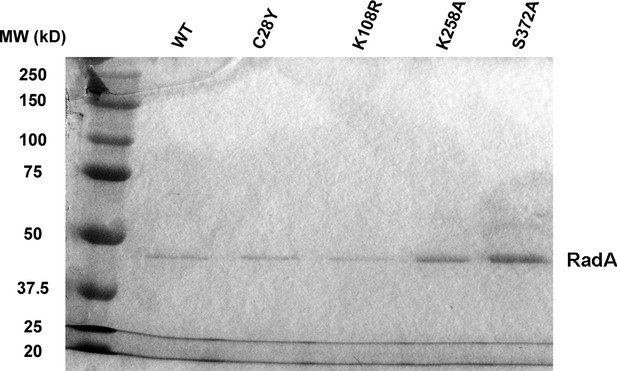
SDS-PAGE of RadA mutants.
SDS-PAGE gel showing purified RadA mutant proteins.
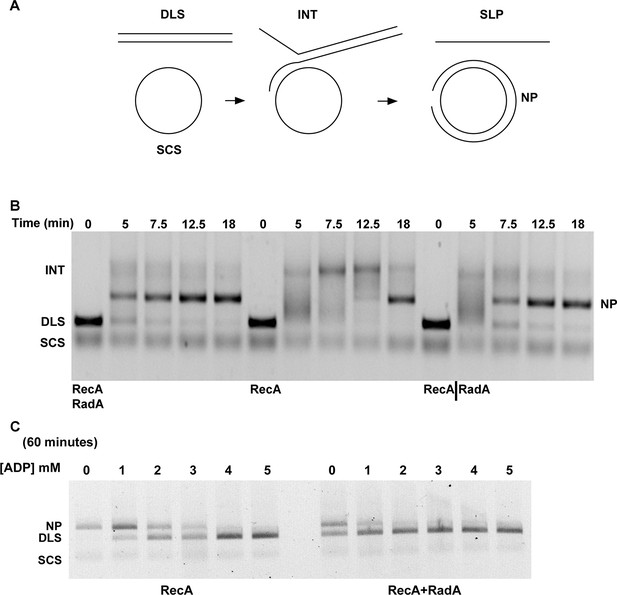
Three-strand Recombination Reactions in the Presence of RadA.
(A) Diagram of thethree-strand recombination reaction. Single-strand circular φX-174 DNA (SCS) was mixed with double-strand φX174 DNA linearized with PstI (DLS) in the presence of RecA, SSB, ATP and an ATP regenerating system. When RadA was included in the reactions, it was added to achieve a RadA:RecA ratio of 1:17. Initially, branched Intermediates (INT) form between the singe-strand circular DNA and its complementary sequence. After continued incubation, nicked circular product (NP) and single-strand linear product (SLP) are formed. Note: The SLP is not usually visible. The standard order of addition for this reaction is: 1) Incubation at 37 °C for 8 min with buffer, ATP regenerating system, φX174 single-strand DNA, and RecA (and RadA when included). 2) Addition of double-strand linear φX174 and continued incubation for 5 min at 37°. 3) Addition of pre-mixed ATP and SSB to initiate the reaction. Incubation then continued for the times indicated. 4) Deproteinization of the reaction and separation of the products from substrates on a 0.8% agarose gel run in TAE. (B) Effect of RadA on Three-strand Recombination Reactions. Three-strand recombination reactions were performed as described above with either RecA and RadA or RecA alone in the first incubation step. In the third set of reactions, RadA was added to reactions containing RecA (split from the RecA alone reaction) five minutes after addition of SSB and ATP. (C) Effect of excess ADP on Three-strand Recombination Reactions. Recombination reactions were performed as described except for the following modifications. No regenerating system was included, but the ATP concentration was increased to 5 mM. ADP was added at the concentrations indicated. Finally, incubation at 37 °C was extended to 60 min.
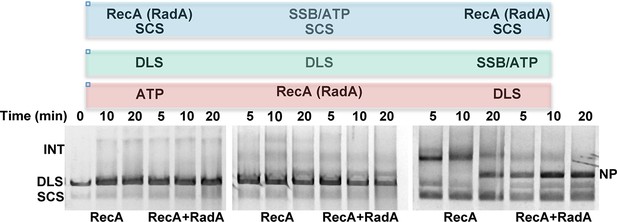
Effect of order of addition of reaction components on 3-strand recombination reactions in the presence or absence of RadA.
Components in the blue box were pre-incubated with buffer and the regenerating system for 8 min at 37 °C. Then, reaction components in the green box were added and pre-incubation was continued for 5 min. Recombination was initiated by the addition of components in the red box. The reactions were then incubated for the times indicated above the gel.
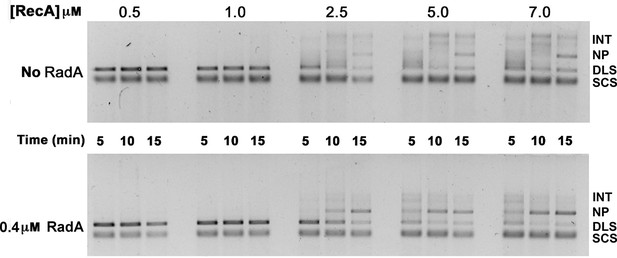
Titration of RecA in 3-strand recombination reactions in the presence or absence of RadA.
Intermediates (INT); Nicked Product (NP); Duplex Linear Substrate (DLS); and Single-strand Circular Substrate (SCS).

3-strand recombination reactions with saturating RecA concentrations and RadA concentrations as indicated.
Intermediates (INT); Nicked Product (NP); Duplex Linear Substrate (DLS); and Single-strand Circular Substrate (SCS).
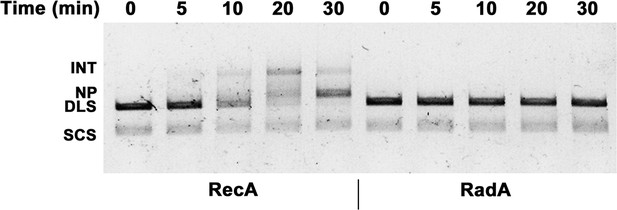
Effect of RadA alone on 3-strand recombination reactions.
Reactions were performed as described in the procedures except 4 μM RecA or Rad A was used.
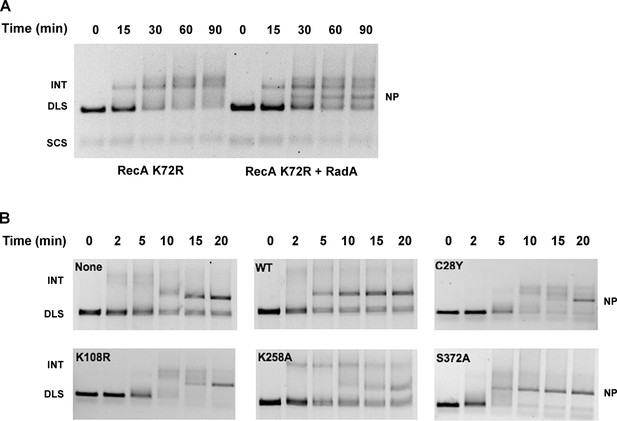
Mutational Analysis of 3-strand Recombination Reactions.
(A) Three-strand recombination reactions with RecA K72R and RadA. Recombination reactions with the mutant RecA K72R were performed as described except dATP replaced ATP and the Mg(OAc)2 concentration was decreased to 3 mM. (B) Three-strand recombination reactions with RadA domain mutants. The reactions were performed as described except with the indicated RadA mutant protein replacing wild-type RadA.
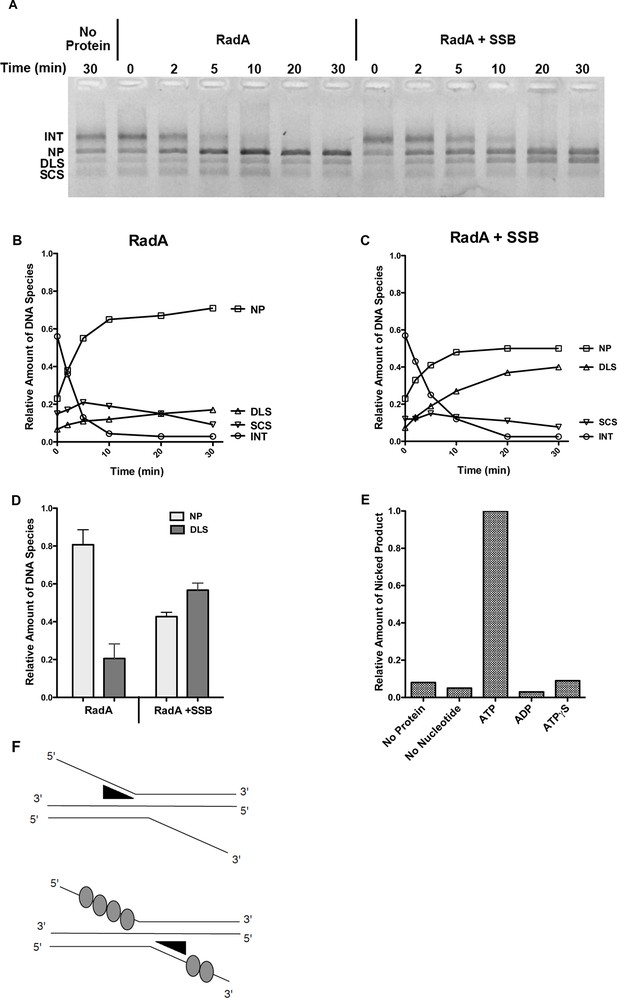
Recombination Intermediate Branch Migration.
(A) Branch Migration of intermediates Mediated by RadA. Three-strand recombination reactions were stopped after 12 min and deproteinized and purified as described in the procedures. Branch migration assays contained DNA intermediates (100 ng), 1 µM RadA, 3 mM ATP, and 2.4 µM SSB when indicated. After incubation for the times incubated, reactions were stopped and products were resolved on an 0.8% TAE agarose gel. The No protein sample includes DNA intermediate fractions and ATP and was incubated for 30 min at 37 °C without RadA or SSB. (B) Quantification of DNA Species in the Branch Migration Assay Formed by RadA. Amounts of each DNA species was determined from scanned digital photographs using ImageJ64 (Nicked Product (NP)-squares, Duplex Linear Substrate (DLS)-triangles, Single-strand Circular Substrates (SCS)-inverted triangles, and Intermediate Substrates (INT)-circles). (C) Quantification of DNA Species in the Branch Migration Assay Formed by RadA and SSB.* Amounts of each DNA species was determined from scanned digital photographs using ImageJ64. (Nicked Product (NP)-squares, Duplex Linear Substrate (DLS)-triangles, Single-strand Circular Substrates (SCS)-inverted triangles, and Intermediate Substrates (INT)-circles). (D) Quantification of the Nicked Product (NP) and Duplex Linear Substrate (DLS) Formed in Branch Migration Assays. Graph shows the mean and standard deviation of the relative amounts of NP and DLS formed in three independent branch migration experiments. Two different RadA preparations and three different DNA Intermediate preparations were used in these experiments. (E) Nucleotide Dependence of the Branch Migration Assay. Reactions were performed as above except 1mM of the nucleotide indicated replaced 3mM ATP. Incubation was for 30 min at 37 °C. (F) Model Depicting RadA Directionality. In the absence of SSB, RadA (illustrated by wedge shape) preferentially migrates DNA, displacing a 5’ ssDNA flap. In the presence of SSB, the directional bias of RadA branch migration is largely eliminated. * No correction for the difference in binding affinity of ethidium bromide for single-strand and double-strand DNA was made. Thus, the absolute amount of the DNA species containing single-strand DNA may be underestimated.
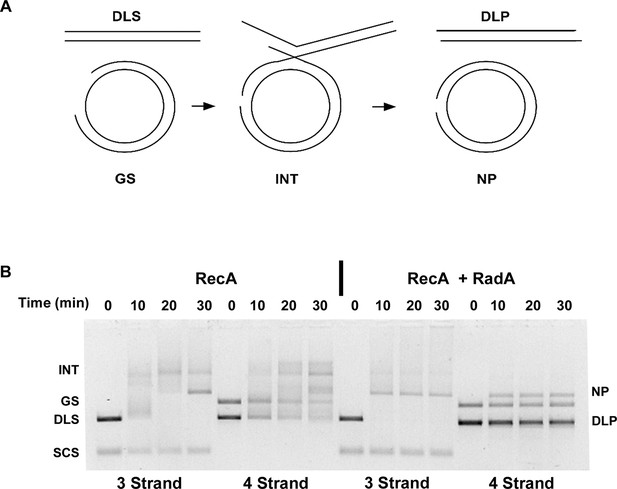
Four-strand Recombination Reactions in the Presence of RadA.
(A) Diagram of the Four-strand Recombination Reaction. Gapped circular substrate (GS) prepared as described in the procedures was mixed with double-strand φX174 DNA linearized with PstI (DLS) in the presence of RecA, SSB, RadA, ATP and an ATP regenerating system. Complex, largely duplex DNA intermediates are formed first. The final products are nicked circular double-DNA (NP) and Duplex Linear DNA with Single-strand Tails (DLP). Note: The tailed linear product species is not well-resolved from the duplex linear substrate (DLS). (B) Comparison of 3-strand and 4-strand Recombination Mediated by RecA in the Presence and Absence of RadA. Recombination reactions between either single-strand circular φX174 DNA (SCS) and double-strand φX174 DNA linearized with PstI (DLS)-3-strand reactions or double-strand circular φX174 with a 1.3 kB single-strand gap (GS) and double-strand φX174 DNA linearized with PstI (DLS)-4-strand reactions were performed as described. At the times indicated, reactions were stopped and de-proteinated. Products were resolved using an 1.0% agarose gel in TAE buffer.
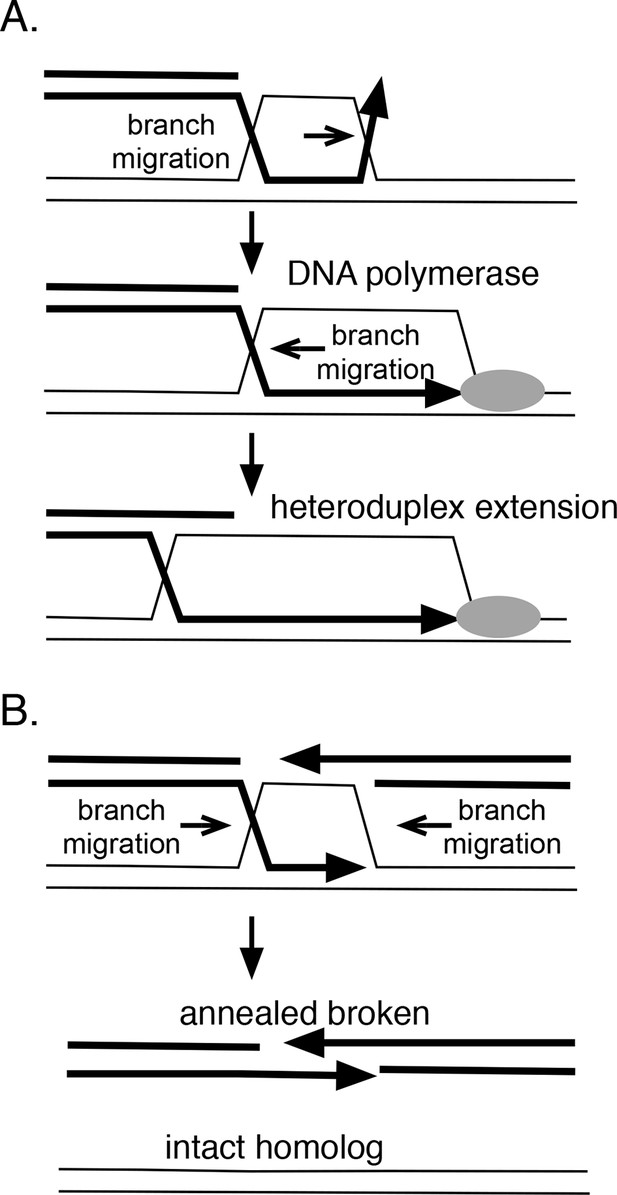
How branch migration assists homologous recombination.
(A) Heteroduplex extension. In reactions between linear resected DNA and an intact chromosome, initial strand pairing and invasion may occur at a distance from the 3’ end. Branch migration of the D-loop (in direction of the arrow) allows the heteroduplex region to extend fully to the 3’ end, allowing it to be engaged by DNA polymerases. Branch migration also allows the D-loop to be extended, lengthening and stabilizing the region of heteroduplex and forming a 4-strand Holliday juntion. (B) Synthesis-dependent strand annealing (SDSA). After resection of a broken chromosome and strand invasion into a sister molecule, branch migration is required to dissolve the intermediate, allowing broken strands to anneal to one another and the break to be healed. Reactions contained 1 mM ATP and 20.3 µM (nucleotide) φX174 DNA. The values shown are the average of two experiments, except for the circular single-strand value. It is the average of five experiments. Standard deviations are reported.
Tables
DNA dependence of RadA ATP hydrolysis.
DNA substrate | Apparent kcat (ATP/RadA/min) |
---|---|
None | 2.9 +/- 0.2 |
Circular single-strand | 29.4 +/- 0.4 |
Supercoiled double-strand | 9.1 +/- 0.4 |
Linear double-strand | 4.2 +/- 0.2 |
Nicked double-strand | 15.2 +/- 0.5 |
-
Reactions contained 1 mM ATP and 20.3 µM (nucleotide) φX174 DNA. The values shown are the average of two experiments, except for the circular single-strand value. It is the average of five experiments. Standard deviations are reported.
Oligonucleotides used in this study.
Oligonucleotide name | Sequence |
---|---|
radAeXactF | GGAAGCTTTGACTTCTGTGGCAAAAGCTCCAAAACG |
radAeXactR | TTTGCGGCCGCTTATAAGTCGTCGAACACGC |
polyd(N)33 | TTAGCGGCCGCATAGTCAAGATGACAATGTTCT |
Substrate E2 | CGGTCAACGTGGGCATACAACGTGGCACTG(T)30ATGTCCTAGCAAAGCGTATGTGATCACTGG |
Jxn1 | CCGCTACCAGTGATCACCAATGGATTGCTAGGACATCTTTGCCCACCTGCAGGTTCACCC |
Jxn2 | TGGGTGAACCTGCAGGTGGGCAAAGATGTCCTAGCAATCCATTGTCTATGACGTCAAGCT |
Jxn3 | AGCTTGACGTCATA |
Jxn4 | GATCACTGGTAGCGG |
Jxn5 | TGCCGATATTGACAAGACGGCAAAGATGTCCTAGCAATCCATTGGTGATCACTGGTAGCGG |