Evolutionary diversification of the trypanosome haptoglobin-haemoglobin receptor from an ancestral haemoglobin receptor
Figures
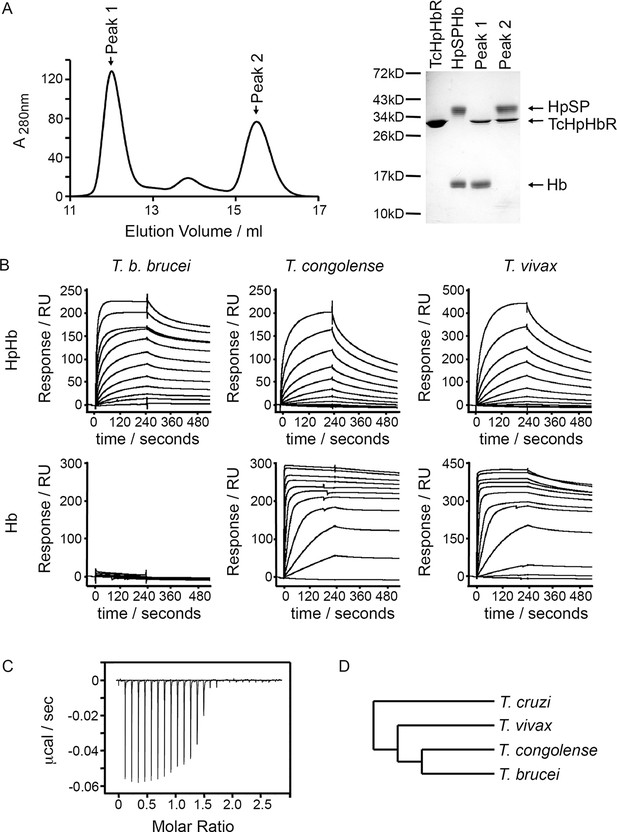
T. congolense and T. vivax HpHbRs are haemoglobin receptors.
(A) Analytical gel filtration chromatography analysis showing the consequence of mixing HpSPHb and TcHpHbR. A mixture of TcHpHbR and HpSPHb was loaded onto the column, resulting in two peaks. The gel shows that peak 1 contains a complex of TcHpHbR bound to Hb, while peak 2 contains free TcHpHbR and free HpSP. (B) Surface plasmon resonance analysis of the binding of HpHbRs from T. b. brucei, T. congolense and T. vivax to either Hb or HpHb. (C) Isothermal titration calorimetry shows the binding of two TcHpHbR to one Hb tetramer. (D) A phylogenetic tree indicating the evolutionary history of the trypanosome strains under study (Stevens et al., 2001).
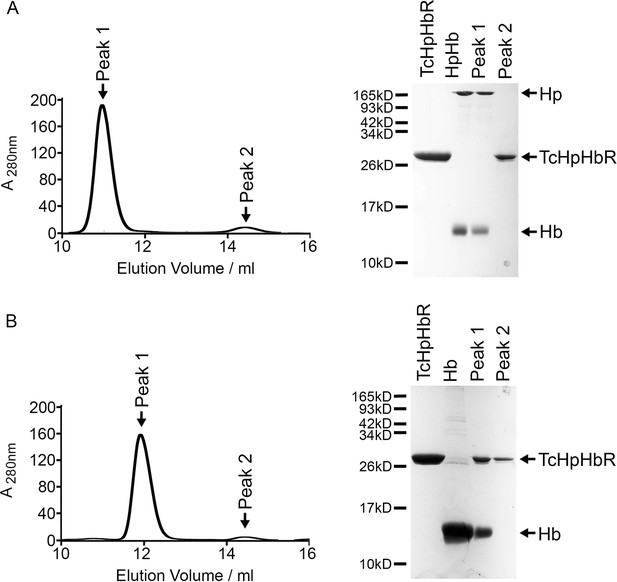
TcHpHbR does not disassemble HpHb.
(A) Analytical gel filtration chromatography analysis showing the consequence of mixing HpHb and TcHpHbR. A mixture of TcHpHbR and HpHb was loaded onto the column, resulting in two peaks. The gel shows that peak 1 contains HpHb while peak 2 contains free TcHpHbR. This shows that TcHpHbR did not disassemble HpHb and that the TcHpHbR:HpHb affinity is not sufficient for the complex to remain stable during the course of a gel filtration experiment. (B) Analytical gel filtration chromatography analysis showing the consequence of mixing Hb and TcHpHbR. A mixture of TcHpHbR and Hb was loaded onto the column, resulting in two peaks. The gel shows that peak 1 contains the TcHpHbR:Hb complex while peak 2 contains free TcHpHbR, showing the formation of a stable complex.
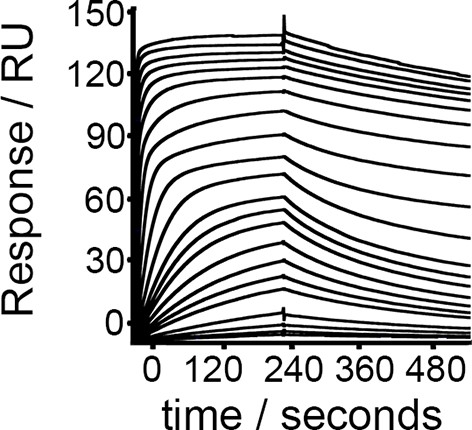
TcHpHbR binding to bovine Hb.
Surface plasmon resonance analysis of the binding of T. congolense HpHbR to bovine Hb.
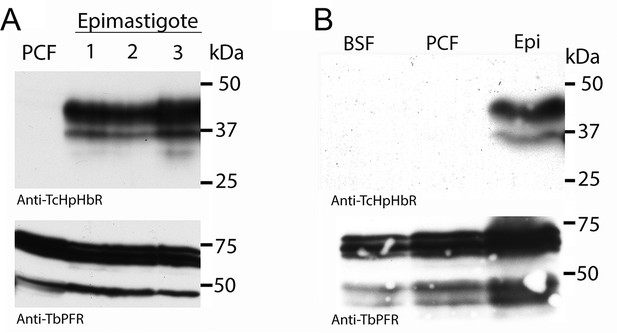
Western analysis reveals high levels of HpHbR expression in epimastigote-enriched cultures.
(A) Three populations of T. congolense epimastigotes were generated in vitro by maintaining procyclic form cultures of T. congolense IL3000 cells at stationary phase (Coustou et al., 2010). The majority of epimastigote forms are adherent and stick to the culture flask while the culture supernatant is predominantly trypomastigotes with a low percentage of detached epimastigote forms. Cell lysates where generated from culture supernatants and subject to western blot analysis. No TcHpHbR protein expression was detected in procyclic form cultures (PCF) whereas TcHpHbR expression was observed in all epimastigote-containing cultures. The protein is observed above the expected 32 kDa, probably due to the GPI-anchor and N-glycosylation affecting mobility as has been observed for the TbHpHbR (Vanhollebeke et al., 2008). Loading control is anti-TbPRF2. (B) No TcHpHbR protein expression was detected in T. congolense bloodstream forms (BSF) or procyclic form cultures (PCF) by western blot, whereas expression was detected in epimastigote-containing cultures (Epi).
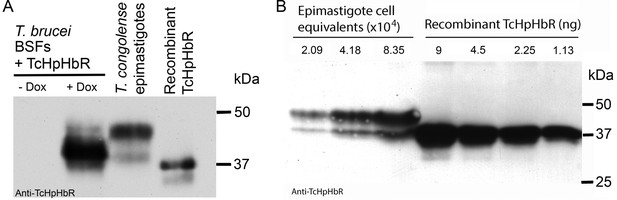
Validation of TcHpHbR antisera and quantification of TcHpHbR protein expression in T. congolense epimastigotes.
(A) Antisera was raised and purified against recombinant TcHpHbR. The antisera recognized two bands in T. congolense epimastigotes that run at a higher molecular weight on an acrylamide gel, as expected of a GPI-anchored and N-glycosylated protein and as observed for the TbHpHbR in T. brucei bloodstream forms, BSFs (Vanhollebeke et al., 2008), with the largest band representing the fully processed form of the protein. To confirm this was indeed the TcHpHbR, T. brucei TbHpHbR KO cells were transfected with an inducible copy of the TcHpHbR in an over-expression vector. Upon induction with doxycycline (Dox), the antisera detected two bands that again ran at a higher molecular weight than the recombinant TcHpHbR. While the upper band represents the minority of protein in this case, this is probably due to differential modification of the GPI-anchor in bloodstream and insect cell forms (Ferguson et al., 1993). (B) Known quantities of recombinant TcHpHbR protein were loaded next to known numbers of TcHpHbR expressing cell equivalents (calculated by IFA analysis on mixed epimastigote and procyclic populations). By two independent western blots 8.35 x 104 cell equivalents was estimated to be equivalent to between 2.25 and 4.5 ng of recombinant protein (an average of between 5 and 9 x 105 molcules per cell).
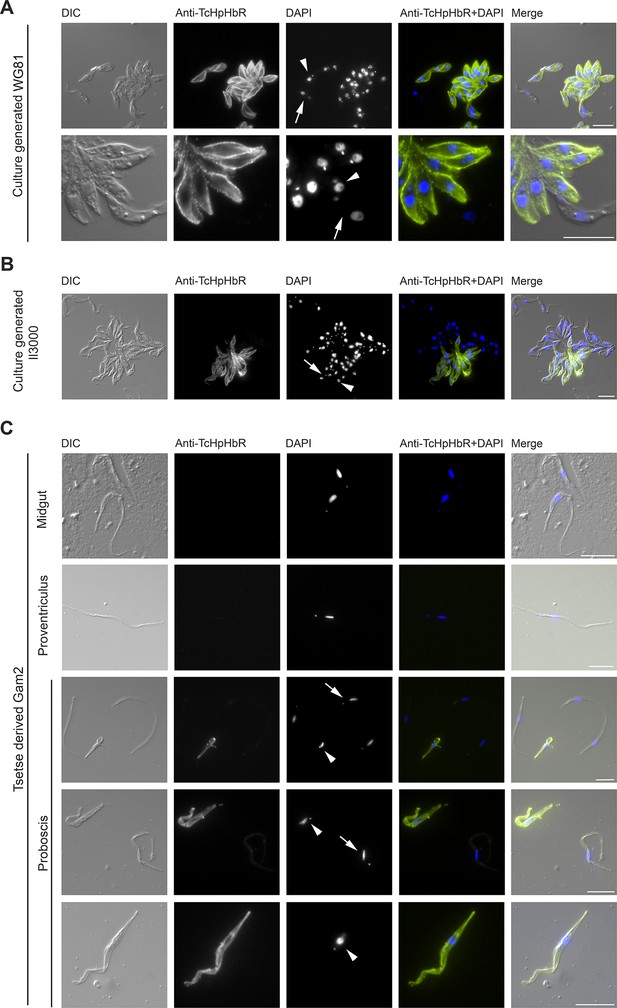
Immunofluorescent analysis of T. congolense epimastigotes reveals TcHpHbR protein is expressed at high levels across the entire cell surface.
(A) Immunofluorescence analysis of paraformaldehyde-fixed (non-permeabilised) in vitro generated T.congolense WG81 epimastigotes with rabbit anti-TcHpHbR antisera and an Alexa488 conjugated anti-rabbit secondary antibody. TcHpHbR was readily detected on the surfaces of all cells where kinetoplast repositioning had occurred. (Arrow highlights a trypomastigote, arrowhead highlights an epimastigote) (B) This was also observed with immunofluorescence analysis of methanol-fixed (permeabilised) in vitro generated T. congolense Il3000 epimastigotes. Occasionally TcHpHbR expression was detected in cells that did not display kinetoplast repositioning. (Arrow highlights a TcHpHbR positive cell without associated kinetoplast repositioning.) (C) T.congolense Gam2 cells were harvested from the midgut (top panel), proventriculus (second panel) and proboscis (lower three panels) of tsetse flies. Cells were fixed with methanol and immunofluorescence analysis was carried out as above. Trypanosomes harvested from the midgut (top panel) were always negative for TcHpHbR and those harvested from the proventiculus (second panel) were mostly negative for TcHpHbR, although occasional cells were identified with a faint positive signal. Epimastigotes harvested from the proboscis (lower three panels, arrowheads) were always strongly positive for TcHpHbR. Trypomastigotes from the proboscis (lower three panels, arrows) showed a faint or negative signal for TcHpHbR (arrows). All scale bars represents 10 µm.
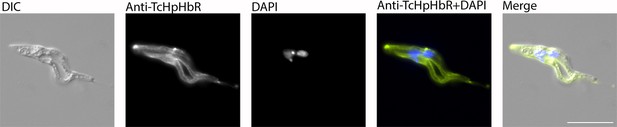
TcHpHbR is expressed on a cell undergoing asymmetric division.
A TcHpHbR-expressing cell was identified that was undergoing asymmetric division, where one daughter cell will be an epimastigote and the other will be a trypomastigote, as occurs during metacyclogenesis in T. brucei. Scale bar represents 10 µm.
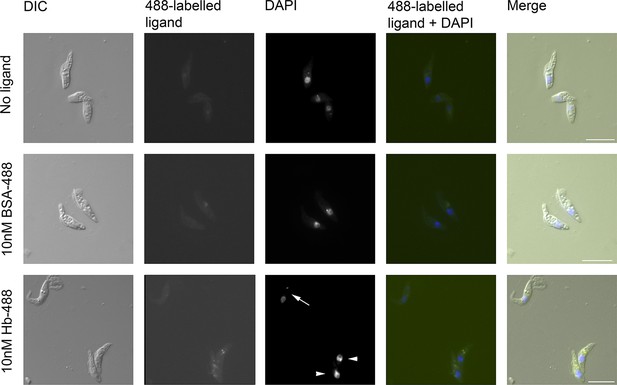
T. congolense epimastigotes internalise 488-labelled Hb.
Assay for uptake of Alexa488-labelled Hb (Hb-488) and Alexa488-labelled BSA (BSA-488) into T. congolense WG81 epimastigotes was monitored by microscopy. Uptake of Hb-488 was detected at 10 nM in epimastigotes (lower panel, arrowheads) but not in trypomastigotes (lower panel, arrow). No fluid phase uptake of BSA-488 at 10 nM in any cells (centre panel).
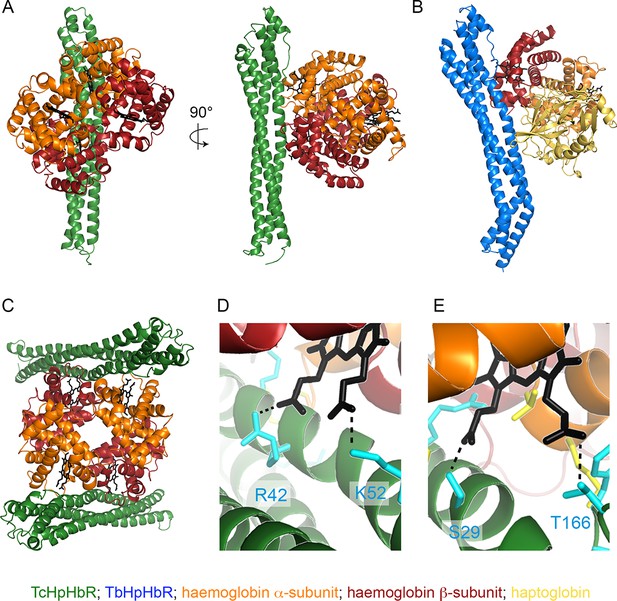
The structural basis of haemoglobin binding by TcHpHbR.
(A) The structure of a complex of TcHpHbR bound to Hb. (B) The structure of the TbHpHbR:HpSPHb complex (Lane-Serff et al., 2014). (C) The contents of the asymmetric unit of the TcHpHbR:Hb crystals, showing two receptors binding to a single haemoglobin tetramer. (D) A close up view of the interaction of TcHpHbR with the β-chain of haemoglobin showing the direct contacts made with the haem group. (E) A close up view of the interaction of TcHpHbR with the α-chain of haemoglobin showing the direct contacts made with the haem group.
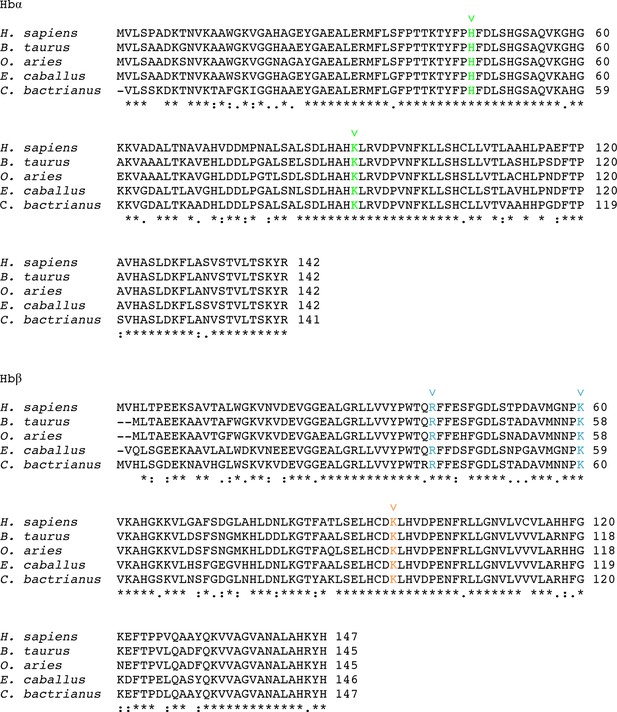
TcHpHbR-binding residues are conserved across mammalian haemoglobins.
Residues in green interact with TcHpHbR in the TcHpHbR:Hb structure. Residues in blue interact with TbHpHbR in the TbHpHbR:HpSPHb structure. Residues in orange interact with both TcHpHbR and TbHpHbR. These residues are conserved in human haemoglobin and also in haemoglobins from livestock species that can be infected by T. congolense.
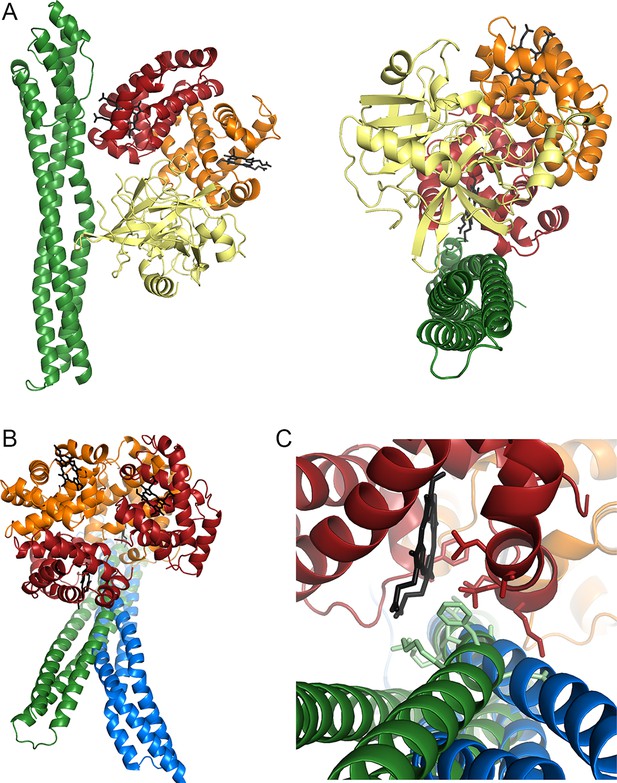
Understanding HpHbR ligand specificity.
(A) A model of TcHpHbR bound to HpSPHb, based on the TcHpHbR:Hb structure. (B, C) The TcHpHbR:Hb and TbHpHbR:HpSPHb complexes have been aligned, with the haemoglobin subunit that interacts with the membrane distal binding site used for the alignment. This shows that a change in the path of the helical bundle of TbHpHbR (blue) prevents the interaction that occurs between TcHpHbR (green) and the membrane proximal haemoglobin subunit. This disruption of the membrane proximal binding site has caused TbHpHbR to lose affinity for Hb
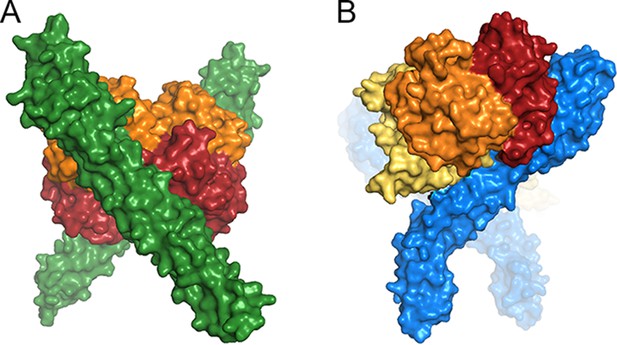
A comparison of ligand binding by HpHbRs from different species.
Space filling models of (A) Two TcHpHbR molecules binding to a single haemoglobin tetramer. (B) Two TbHpHbRs bound to a single haptoglobin-haemoglobin dimer.
Tables
Crystallographic statistics.
Beamline | Diamond I03 |
Space Group | P22121 |
Cell parameters (Å) | a=72.75, b=127.3, c=172.42 |
Resolution (Å) | 101.89-3.2 |
Wavelength (Å) | 0.976 |
RPIM (%) | 7.3 (41.0) |
I/ σ(I) | 7.3 (2.4) |
Completeness (%) | 99.0 (99.1) |
Multiplicity | 2.7 (3.0) |
Resolution (Å) | 3.2 |
No. reflections | 25191 |
Rwork / Rfree (%) | 20.44 / 23.51 |
No. of protein residues in model | 1063 |
rmsd bond lengths (Å) | 0.010 |
rmsd bond angles (°) | 1.21 |
Ramachandran plot | |
Preferred region | 93.1% |
Allowed region | 6.9% |
Outliers | 0% |
Description of interactions between TcHpHbR and Hb.
TcHpHbR | Hb | ||||
---|---|---|---|---|---|
Residue | Group | Chain | Residue | Group | Interaction |
Hbα | |||||
S29 | backbone CO | C/E | H46 | side chain | Hydrogen bond |
S29 | side chain | C/E | Haem | O1 | Hydrogen bond |
I30 | side chain | C/E | Patch | Hydrophobic | |
R37 | side chain NH1/NH2 | C/E | L92 | backbone CO | Hydrogen bond |
K127 | side chain | C/E | P45 | backbone CO | Hydrogen bond |
K130 | side chain | C/E | Patch | Hydrophobic | |
T166 | side chain | C/E | Haem | O3 | Hydrogen bond |
Y168 | side chain | C/E | Patch | Hydrophobic | |
Y168 | backbone CO | C/E | K91 | side chain | Hydrogen bond |
D169 | side chain | C/E | K91 | side chain | Salt bridge |
Hbβ | |||||
I41 | side chain | D/F | Patch | Hydrophobic | |
R42 | side chain NH2 | D/F | Haem | O1 | Hydrogen bond |
A44 | side chain | D/F | Patch | Hydrophobic | |
T45 | side chain | D/F | Patch | Hydrophobic | |
E47 | side chain OE2 | D/F | K96 | side chain | Salt bridge |
F48 | side chain | D/F | Patch | Hydrophobic | |
K52 | side chain | D/F | Haem | O3 | Hydrogen bond |