Endocannabinoid dynamics gate spike-timing dependent depression and potentiation
Figures
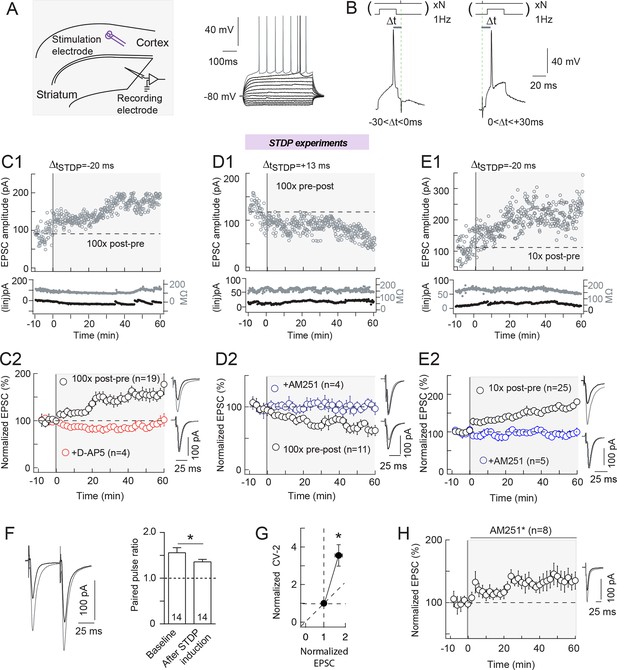
Bidirectional endocannabinoid-mediated STDP depends on the number of pairings.
(A) Whole-cell recording from the dorsal striatum with the stimulation electrode placed in layer 5 of the somatosensory cortex in horizontal rat brain slice. (B) Experimental design. Extracellular stimulation evoked EPSCs monitored at RMP. During pairings, recordings were switched to current-clamp to allow postsynaptic MSN to fire single action potentials paired with single cortical extracellular stimulations. MSN and cortical stimulation were repeated N times (10 or 100) at 1 Hz. ΔtSTDP indicates the time delay between pre- and post-synaptic stimulations. -30<ΔtSTDP<0 ms and 0<ΔtSTDP<+30 ms refers to post-pre and pre-post pairings, respectively. (C) 100 post-pre pairings induced NMDAR-mediated tLTP. (C1) Example of tLTP induced by 100 post-pre pairings. Top, EPSC strength before and after pairings (before pairings: 91±3 pA; 45–55 min after pairings: 169±2 pA; increase of 87%). Bottom, time courses of Ri (before, 132±1 MΩ; after, 134±2 MΩ; change of 2%) and injected current (Iinj) (before, -2±1 pA; after, -12±2 pA; change of 6% of baseline EPSC amplitude) for this cell. (C2) Summary of tLTP induced by 100 post-pre pairings. 15/19 cells showed significant tLTP. Inhibition of NMDAR with D-AP5 (50 μM, n=4) prevented the induction of tLTP; 4/4 cells showed no significant plasticity. The normality of D-AP5 data was assumed (test not passed). (D) 100 pre-post pairings induced CB1R-mediated tLTD. (D1) Example of tLTD induced by 100 pre-post pairings. Top, EPSC strength before and after pairings (before pairings: 134±2 pA; 45–55 min after pairings: 82±2 pA; decrease of 39%). Bottom, time courses of Ri (before, 156±2 MΩ; after, 157±1 MΩ; change of 1%) and injected current (Iinj) (before, 14±1 pA; after, 20±1 pA; change of 5%) for this cell. (D2) Summary of tLTD induced by 100 post-pre pairings. 7/11 cells showed significant tLTD. Inhibition of CB1R with AM251 (3 μM, n=4) prevented the induction of tLTD; 4/4 cells showed no significant plasticity. The normality of AM251data was assumed (test not passed). (E) 10 post-pre pairings induced CB1R-mediated tLTP. (E1) Example of tLTP induced by 10 post-pre pairings. Top, EPSC strength before and after pairings (before pairings: 112±4 pA; 45–55 min after pairings: 213±4 pA; increase of 90%). Bottom, time courses of Ri (before, 171±2 MΩ; after, 167±1 MΩ; change of 2%) and injected current (Iinj) (before, 10±1 pA; after, 12±1 pA; change of 2%) for this cell. (E2) Summary of tLTP induced by 10 post-pre pairings. 21/25 cells showed significant tLTP. Inhibition of CB1R with AM251 (3 μM, n=5) prevented the induction of tLTP; 5/5 cells showed no significant plasticity. Normality was assumed for the ctrl 10x post-pre data (test not passed). (F-H) eCB-LTP is maintained by a mechanism located downstream of CB1R activation in the presynaptic terminals. (F) Representative EPSCs and summary bar graphs (n=14) of paired-pulse cortical stimulations (50 ms interstimulus interval) illustrate a decrease of facilitation after 10 post-pre pairings. This indicates a presynaptic locus of the eCB-tLTP. (G) Mean variance analysis (CV-2, n=17) indicates a presynaptic locus of the eCB-tLTP maintenance. (H) Summary of tLTP induced by 10 post-pre pairings with application of CB1R inhibitor just after the pairings (AM251*) (7/8 cells showed significant tLTP). This treatment did not prevent tLTP, indicating that the maintenance of eCB-tLTP does rely on the signaling downstream of CB1R. Normality was assumed for the data og CV-2 after STDP protocol (test not passed). Representative traces are the average of 15 EPSCs during baseline (black traces) and 50 min after STDP protocol (grey traces). Error bars represent s.d. *p<0.05. ns: not significant.
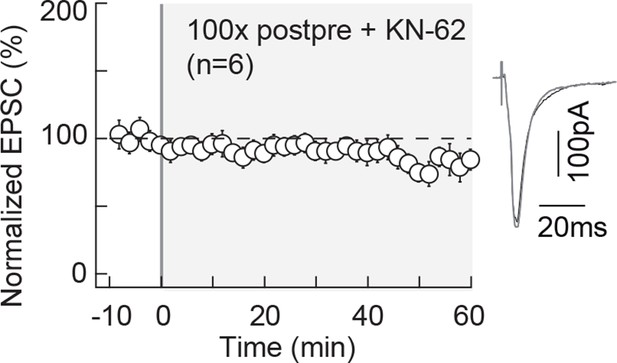
NMDAR-tLTP relies on CaMKII activity.
NMDAR-mediated tLTP induced by 100 post-pre pairings is CaMKII-activation dependent. Summary of tLTP induced by 100 post-pre pairings in control conditions (n=19) and the absence of plasticity observed with KN62 (3 μM, n=6); 15/19 and 1/6 cells showed significant tLTP, respectively. Representative traces are the average of 15 EPSCs during baseline (black traces) and 50 min after STDP protocol (grey traces). Error bars represent s.d. *p<0.05. ns: not significant.
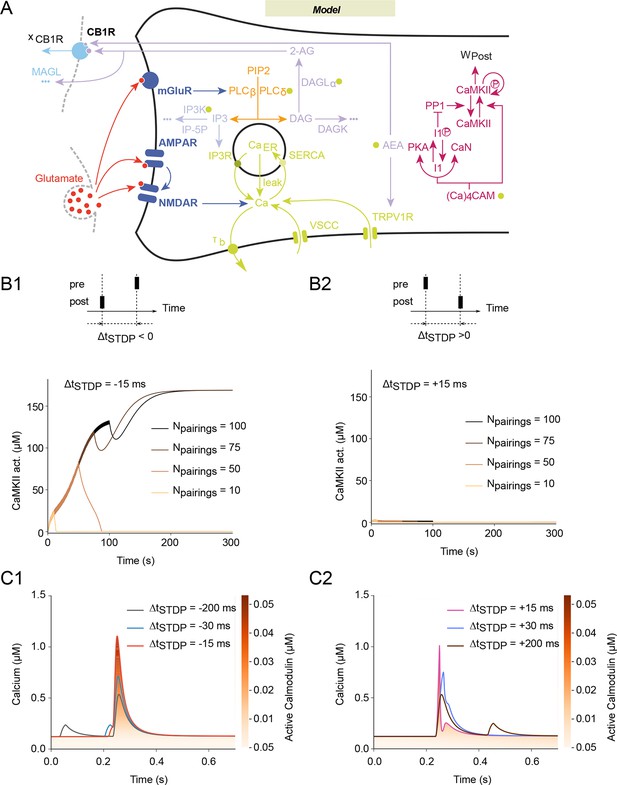
Mathematical model predicts NMDAR-tLTP with large numbers of post-pre pairings.
(A) Scheme of the modeled signaling network. The synaptic weight Wtotal is the product of pre- and postsynaptic weights Wpre and Wpost. The NMDAR-based pathway sets Wpost as the phosphorylation state of postsynaptic CaMKIIα. In the second pathway, coincident activation of phospholipase Cβ by postsynaptic mGluR and calcium entry via VSCC and TRPV1 induces the production of 2-AG and AEA. 2-AG, and to a lower extent AEA, activates CB1R (xCB1R is the fraction of non-desensitized CB1R), which then modulates the presynaptic weight, Wpre. Color code: glutamate receptors: dark blue; PLC pathway: yellow; IP3 pathway: powderblue; calcium pathways: green (green disks indicate calcium-dependent steps); DAGLα pathway: lavander; AEA pathway: light blue; CB1R pathway: blue. Abbreviations: PIP2: phospatidylinositol 4,5-biphosphate; DAG: diacylglycerol; IP3: inositol-1,4,5-triphosphate; PLCβ/δ: phospholipase C β/δ; DAGK: diacylglycerol kinase; IP-5P: inositol polyphosphate 5-phosphatase; IP3K: IP3-kinase; DAGLα: diacylglycerol lipase α;B/BCa: free/bound endogeneous calcium buffer; IP3R: IP3-receptor channel; SERCA: sarcoplasmic/endoplasmic reticulum calcium ATPase; CaER: calcium in the endoplasmic reticulum; (Ca)4CaM: fully bound calmodulin; CaN: calcineurin aka PP2B; PKA: protein kinase A; I1p/I1: phosphorylated/unphosphorylated protein phosphatase 1 inhibitor 1 (DARPP-32 in striatal output neurons); PP1: protein phosphatase 1; pCaMKII/CaMKII: phosphorylated/unphosphorylated CaMKII; DAGK: diacylglycerol kinase; MAGL: monoacylglycerol lipase; the '…' sign indicates transformation into products that are considered not to interfere with the other interactions of the model. (B) Corresponding changes in the levels of active CaMKII starting from the down (non-activated) state. The number of pairings, Npairings, is indicated for 1 Hz pairings at spike-timing ΔtSTDP=-15 (B1) or +15 (B2) ms. (C) Intracellular calcium changes for the first pairing in post-pre (C1) or pre-post (C2) pairing protocols. The colorcode shows the corresponding amount of calmodulin activation according to the colorbar.
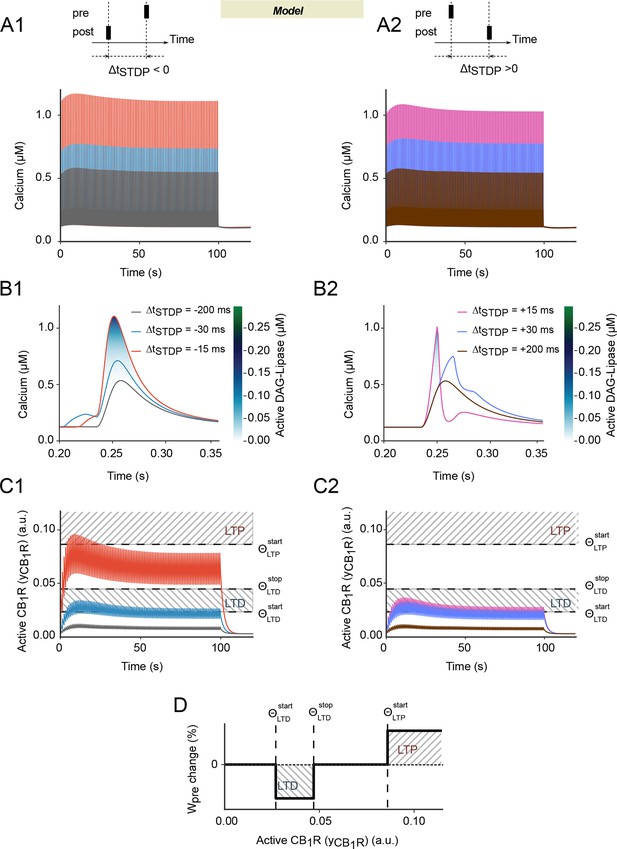
Spike-timing dependence in the endocannabinoid-signaling part of the model.
(A-C) Predicted dynamics of cytoplasmic calcium and CB1R activation for post-pre (first column) or pre-post pairings (second column): in our model, the postsynaptic calcium peaks (A, B) are slightly more width at large calcium values with post-pre (A1-B1) than pre-post pairings (A2-B2). As a consequence, DAGLα activation (color-coded in B) and the resulting CB1R activation, yCB1R (C) is larger too. The biphasic envelope of the calcium peak amplitude with the number of pairings (A) is amplified as a marked biphasic envelope for the amplitude of yCB1R. (C) As a result, whatever the stimulation, the amplitude of the yCB1R peaks first increases for the first 10–20 pairings, then decreases to converge to roughly constant amplitude. But yCB1R reaches large values only for short post-pre pairings (C1). This particular dynamics of yCB1R during the stimulations suggests a possible explanation to the bidirectional characteristics of eCB-dependent plasticity, where the presynaptic contribution to the synaptic weight Wpre depends on the magnitude of yCB1R. (D) Wpre decreases (LTD) when yCB1R reaches an intermediate range whereas it increases (LTP) if yCB1R overcomes a LTP threshold. The corresponding thresholds and ranges are reported in C1-2 as dashed lines and hashed boxes, respectively.
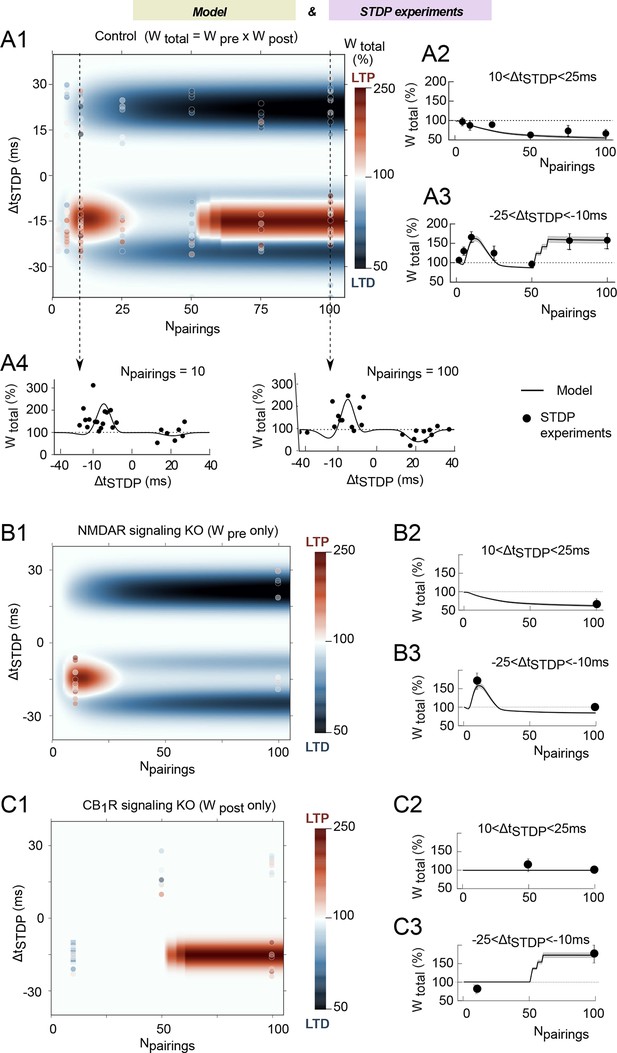
The mathematical model matches the experimental data.
(A) Changes of the total synaptic weight Wtotal (LTP and LTD) when Npairings and ΔtSTDP vary. (A1) Color-coded changes of Wtotal in the (Npairings, ΔtSTDP) space. The color bar indicates the color code. The background map shows the simulation results whereas the color-coded points (same color-code as the simulations) are experimental results. The average changes with Npairings of Wtotal integrated over short positive or short negative ΔtSTDP are shown in (A2) and (A3), respectively. Cross-sections of the two-dimensional map (A1) along the N-axis are shown as changes of Wtotal with ΔtSTDP, for Npairings=10 (A4) or 100 (A5) pairings at 1 Hz. In (A2-A5), full black lines represent the simulation results whereas the full black circles show experimental results. (B,C) Corresponding results obtained with variants of the mathematical model where NMDAR-signaling (B) or eCB-signaling (C) were knocked-out in silico. The 2D maps (B1, C1) use the same color code and symbols as (A1). The average changes of Wtotal over short positive or short negative spike timings ΔtSTDP (B2,C2) and (B3,C3), respectively, use the same symbols as (A1-2).
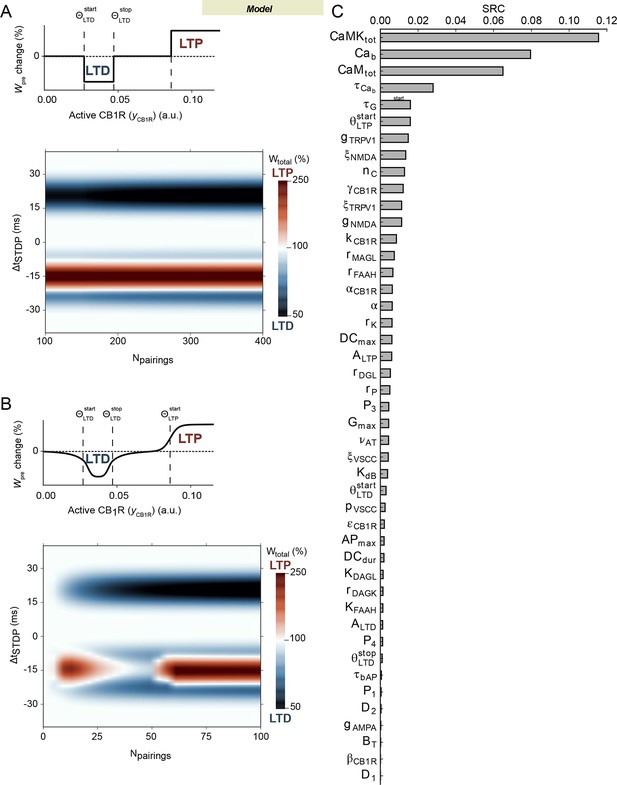
Robustness of the model.
(A) Output of the model for more than 100 pairings and (upper panel) the sharp threshold mechanism for eCB-plasticity given by Equation 1 The behavior observed with 100 pairings is conserved. (B) Output of the model when the sharp eCB plasticity (Equation 1) is replaced by a smooth function (upper panel, given by eq.S1-S2 of Supplementary file 2, here used with kS = 2). Even without changing any of the model parameters outside the threshold function, the model output with such smooth thresholds is very similar to the one obtained with the sharp threshold of Equation 1 (compare with Figure 4A1). Other values of kS essentially lead to the same conclusion. (C) Sensitivity analysis of the model parameters. On the y-axis, the parameters are ranked according to their standardized linear-regression coefficient (SRC, see Materilas and methods) that measures the sensitivity of the model output to variations of the parameter.
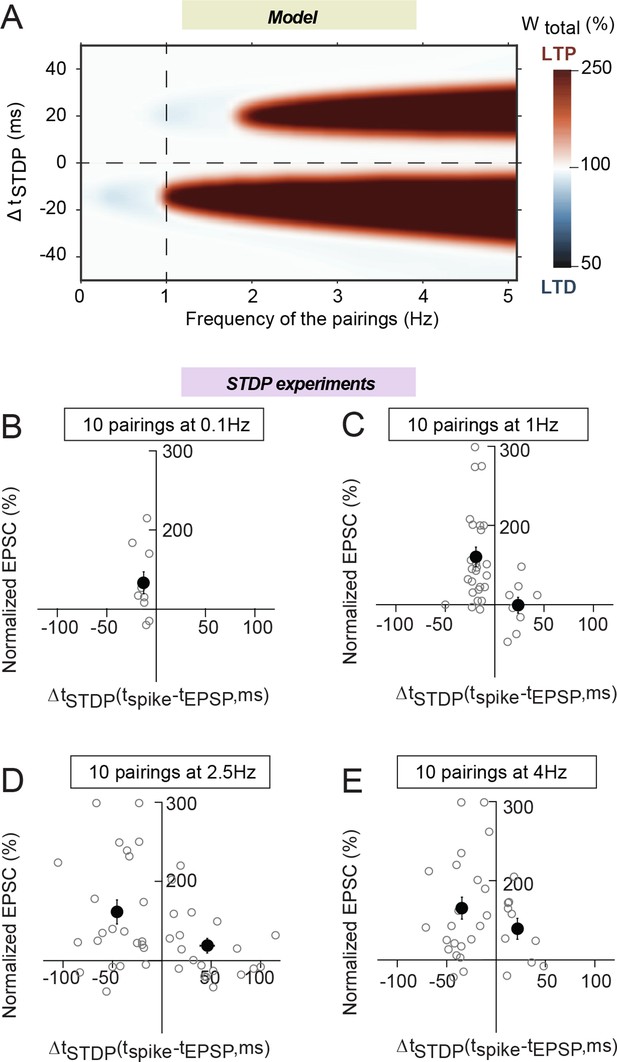
Frequency dependence of eCB-tLTP.
(A) Color-coded changes of Wtotal in the (ΔtSTDP, frequency) parameter space for 10 pairings. Except the pairing frequency, all parameters are the same as in Figure 4 (values given in Supplementary file 1A-C). For 10 post-pre pairings (ΔtSTDP<0), tLTP disappears quickly below 1 Hz but is maintained above 1 Hz, within an even enlarged ΔtSTDP range. For 10 pre-post pairings (ΔtSTDP>0), a new tLTP emerges for frequencies larger than 2 Hz. (B-D) Summary graphs of STDP occurrence for 10 pairings at 0.1 Hz (B), 1 Hz (C), 2.5 Hz (D) and 4 Hz (E); each grey empty circle represent the synaptic efficacy changes 45–50 min after pairings protocols for a single neuron; the black circles represent the averages of plasticity. tLTP was induced with 10 post-pre pairings at 0.1 Hz (7/10 cells showed significant tLTP) and 1 Hz (21/25 cells showed significant tLTP); no significant plasticity was observed for pre-post pairings. For 10 pairings at 2.5 and 4 Hz, symmetric Hebbian plasticity (tLTP for post-pre and pre-post pairings) was observed in an enlarged ΔtSTDP; at 2.5 Hz for post-pre and pre-post pairings, 18/23 and 10/20 cells displayed significant tLTP; at 4 Hz for post-pre and pre-post pairings, 18/22 and 7/10 cells showed significant tLTP. Normality was assumed for the post-pre pairings data (test not passed).
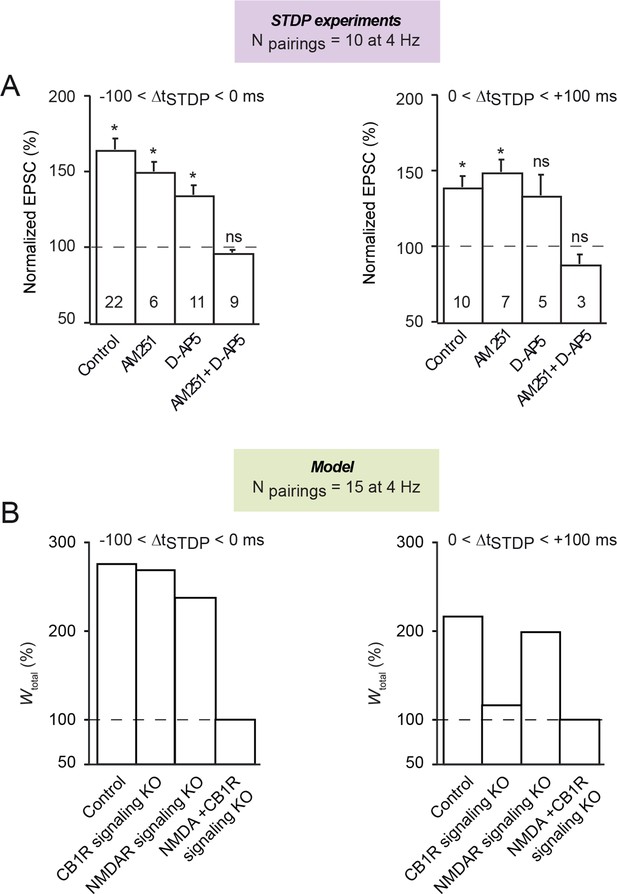
Both CB1R and NMDAR are involved in symmetric hebbian plasticity induced with 10 pairings at 4 Hz.
(A) Summary bar graphs illustrate that symmetric Hebbian plasticity (tLTP) induced with post-pre and pre-post were not prevented by AM251 (3 μM) or D-AP5 (50 μM) (except for pre-post pairings) but were precluded by the application of both antagonists AM251+D-AP5; with AM251, 6/6 and 6/7 cells showed significant tLTP with 10 post-pre and pre-post pairings, respectively; with D-AP5, 8/11 and 3/5 cells showed significant tLTP with 10 post-pre and pre-post pairings, respectively; with AM251+D-AP5, 8/9 and 3/3 cells showed an absence of significant plasticity with 10 post-pre and pre-post pairings, respectively. Error bars represent SEM. *p<0.05. ns: not significant. (B) The mathematical model predicts similar behavior for Npairings = 15 (at 4 Hz). Except the pairing frequency, all parameters are the same as in Figure 4 (values given in Supplementary file 1A-C). With post-pre pairings (ΔtSTDP<0), removing CB1R- or NDMAR-signaling (see Figure 4) separately only partially impairs tLTP, while no plasticity can be obtained when both are removed. Comparable results are obtained with pre-post pairings (ΔtSTDP>0), with the exception that tLTP is much more dependent on CB1R- signaling. Note that the additional NMDAR component of the post-pre tLTP emerges in the model at 4 Hz for Npairings>12.
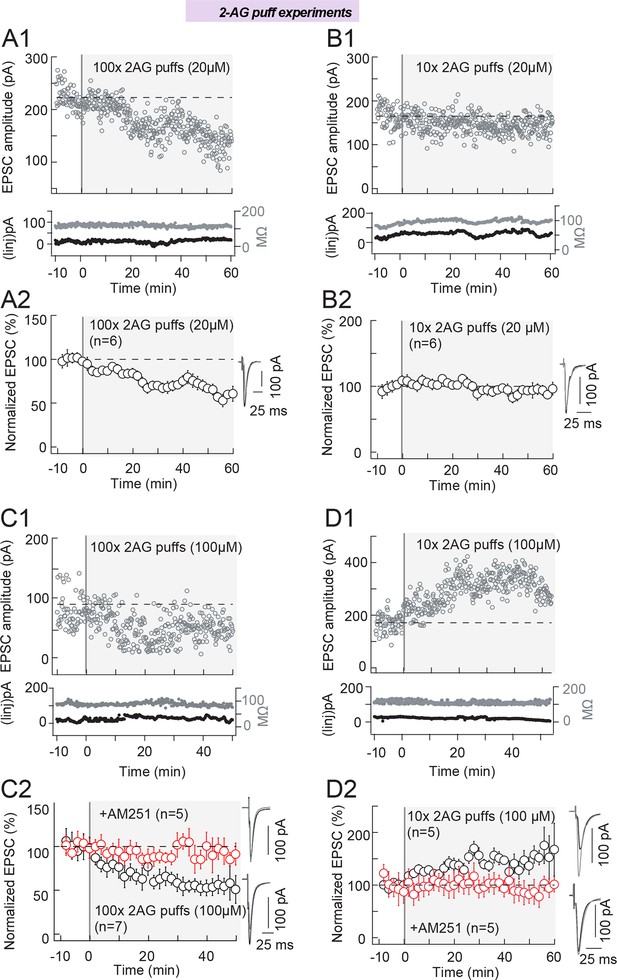
2-AG level and duration control the eCB-plasticity polarity.
(A) Repeated (100 times) brief application of 2-AG (at a low concentration, 20 μM) induces LTD. A series of 100 2-AG puffs (20 μM, 300 ms duration each) delivered at 1 Hz at the vicinity (50–100 μm) of the recorded striatal neuron, induced LTD in the absence of any STDP protocol (n=6). (A1) Example of LTD induced with 100 puffs of 20 μM 2-AG. Top, EPSC strength before and after 2-AG puffs (before 2-AG puffs: 223±3 pA; 45–55 min after 2-AG puffs: 144±3 pA; decrease of 35%). Bottom, time courses of Ri (before, 118±1 MΩ; after, 111±1 MΩ; change of 6.2%) and injected current (Iinj) for this cell. (A2) Summary of LTD induced with 100 puffs of 20 μM 2-AG; 6/6 cells showed significant LTD. (B) Repeated (10 times) brief application of 2-AG (at a low concentration, 20 μM) failed to induce plasticity. (B1) Example of absence of plasticity observed with 10 puffs of 20 μM 2-AG. Top, EPSC strength before and after 2-AG puffs (before 2-AG puffs: 165±3 pA; 45–55 min after 2-AG puffs: 143±2pA; change of 14%). Bottom, time courses of Ri (before, 82±1MΩ; after, 93±1MΩ; change of 13%) and injected current (Iinj) for this cell. (B2) Summary of absence of plasticity observed with 10 puffs of 20 μM 2-AG; 2/6 cells showed no significant plasticity. (C) Repeated (100 times) brief application of 2-AG (at a high concentration, 100 μM) induces LTD. (C1) Example of LTD induced with 100 puffs of 100 μM 2-AG. Top, EPSC strength before and after 2-AG puffs (before 2-AG puffs: 95±3 pA; 45–55 min after 2-AG puffs: 77±1 pA; decrease of 19%). Bottom, time courses of Ri (before, 92±1 MΩ; after, 78±1 MΩ; change of 15%) and injected current (Iinj; before, 16±1 pA; after, 26±1 pA; change of 12.8%) for this cell. (C2) Summary of LTD induced with 100 puffs of 100 μM 2-AG; 7/7 cells showed significant LTD. This 2-AG-mediated LTD was prevented by AM251 (3 μM, n=5); 5/5 cells showed no significant LTD. (D) Repeated (10 times) brief application of 2-AG (at a high concentration, 100 μM) induces LTP. (D1) Example of LTP induced with 10 puffs of 100 μM 2-AG. Top, EPSC strength before and after 2-AG puffs (before 2-AG puffs: 171±4 pA; 45–55 min after 2-AG puffs: 331±6 pA; increase of 94%). Bottom, time courses of Ri (before, 119±1 MΩ; after, 109±1 MΩ; change of 8.4%) and injected current (Iinj) (before, 26±1 pA; after, 18±1 pA; change of 4.7%) for this cell. (D2) Summary of LTP induced with 10 puffs of 100 μM 2-AG; 4/5 cells showed significant LTP. This 2-AG-mediated LTP was prevented by inhibition of CB1R with AM251 (3 μM, n=5); 5/5 cells showed no significant plasticity. Example recording monitoring EPSCs (at 0.1 Hz) (A1, B1, C1 and D1) before and after 2-AG puffs, together with the time course of Ri and of the injected current (Iinj). Summary (A2, B2, C2 and D2) show global average of experiments with error bars representing s.d. Representative traces are the average of 15 EPSCs during baseline (black traces) and 50 min after STDP protocol (grey traces). *p<0.05. ns: non-significant.
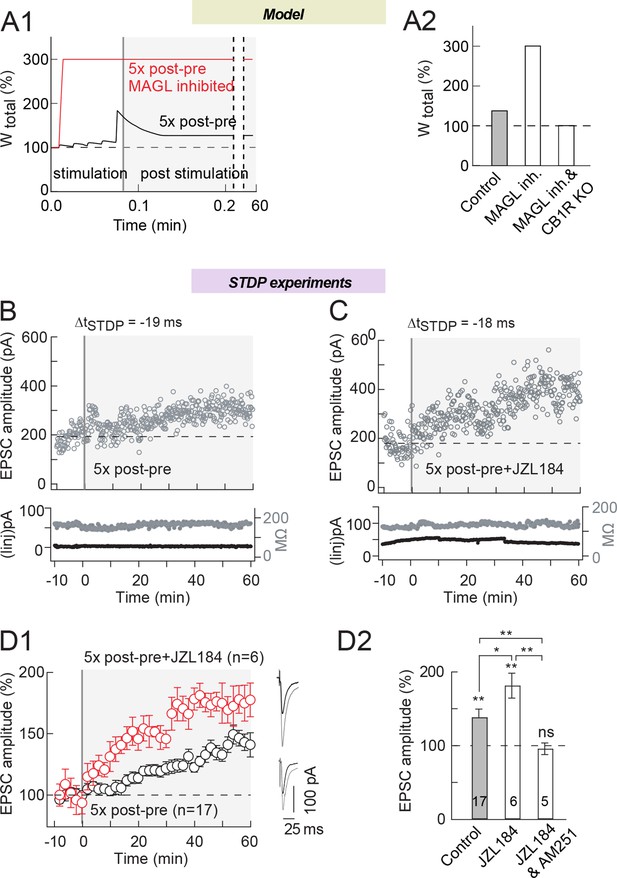
MAGL inhibition increases eCB-tLTP magnitude induced by 5 pairings.
(A) Model prediction for eCB-LTP amplitude induced by Npairings=5 post-pre pairings with ΔtSTDP=-15 ms. (A1) In control (full black line), the synaptic weight increases during the 5 s stimulation protocol (white background) and stabilizes afterwards (gray background) to a moderate tLTP. When eCB production is amplified in the model by MAGL inhibition and DAG-Kinase activity (full red line), the amplitude of the tLTP resulting from the same stimulation is drastically amplified. In the model, MAGL and DAG-Kinase inhibition were simulated by fixing the value of the maximal rates of each enzyme to 0 and 5%, respectively, of their default values listed in Supplementary file 1C. (A2) Summary bar graph of the tLTP amplitude predicted by the model for Npairings=5 post-pre pairings, ΔtSTDP=-15 ms. (B) Corresponding example of experimental tLTP induced by 5 post-pre pairings. Top, EPSC strength before and after pairings (before pairings: 145±4 pA; 45–55 min after pairings: 247±5 pA; increase of 70%). Bottom, time courses of Ri (before, 165±1 MΩ; after, 170±1 MΩ; change of 3.0%) and injected current (Iinj) (before, 2±0.2 pA; after, 3±0.1 pA; change of 0.7%) for this cell. (C) MAGL inhibition by JZL184 (1.5 μM) led to an increase of tLTP magnitude. Example of tLTP induced by 10 post-pre pairings with bath-applied JZL184. Top, EPSC strength before and after pairings (before pairings: 180±6 pA; 45–55 min after pairings: 412±7 pA; increase of 129%). Bottom, time courses of Ri (before, 116±1 MΩ; after, 126±1 MΩ; change of 8.6%) and injected current (Iinj) (before, -13±1 pA; after, -8±1 pA; change of 2.8%) for this cell. (D) Summary of tLTP induced by 5 post-pre pairings in control conditions and with JZL184 treatment. 10/17 and 6/6 cells showed significant tLTP in control and in JZL184, respectively. Normality was assumed for the ctrl 5x post-pre data (test not passed). (E) Summary bar graph illustrates that tLTP magnitude was increased by MAGL inhibition (JZL184) while prevented by CB1R inhibition (JZL184 1.5 μM +AM251 3 μM). Representative traces are the average of 15 EPSCs during baseline (black traces) and 50 min after STDP protocol (grey traces). *p<0.05. ns: non-significant.
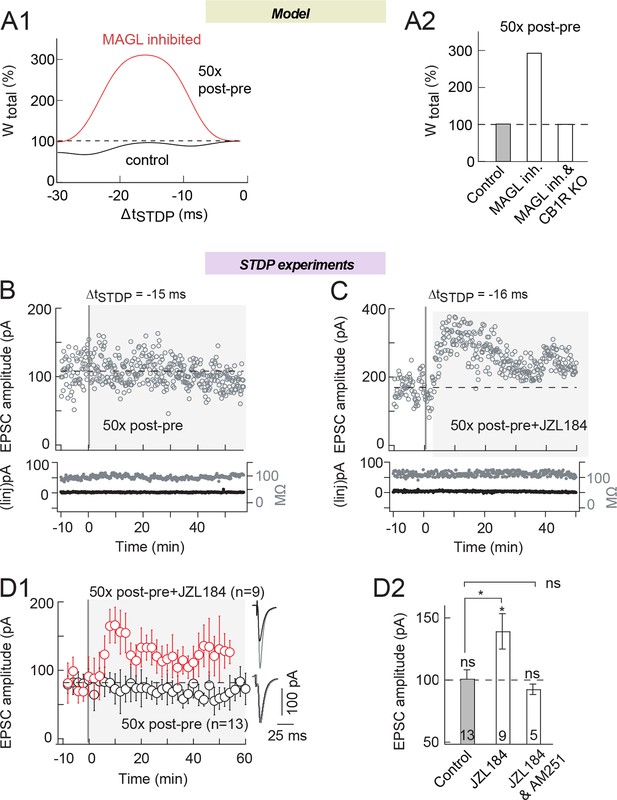
MAGL inhibition unveils eCB-tLTP expression with 50 pairings.
(A) Model prediction for the plasticity induced by Npairings=50 post-pre pairings. (A1) In control (full black line), the synaptic weight is unchanged by 50 post-pre pairings for 0>ΔtSTDP>-25 ms. Amplified eCB production due to MAGL inhibition (full red line), uncovers a large-amplitude tLTP. In the model, MAGL inhibition was emulated by setting its maximal rate to 40% of its default value (Supplementary file 1C). (A2) Summary bar graph of the tLTP amplitude predicted by the model for Npairings=50 post-pre pairings at ΔtSTDP =-15 ms. (B) 50 post-pre pairings did not induce significant plasticity. Example of the absence of plasticity observed when 50 post-pre pairings were applied. Top, EPSC strength before and after pairings (before pairings: 108±3 pA; 45–55 min after pairings: 106±2 pA; change of 2%). Bottom, time courses of Ri (before, 92±1 MΩ; after, 91±1 MΩ; change of 0.1%) and injected current (Iinj) (before, 2±0.2 pA; after, 2±0.1 pA; no detectable change) for this cell. (C) 50 post-pre pairings induced tLTP with MAGL inhibition. Example of tLTP induced by 50 post-pre pairings with bath-applied JZL184 (1.5 μM). Top, EPSC strength before and after pairings (before pairings: 170±4 pA; 45–55 min after pairings: 243±4 pA; increase of 43%). Bottom, time courses of Ri (before, 110±1 MΩ; after, 113±1 MΩ; change of 2.7%) and injected current (Iinj) (before, 4±0.3 pA; after, 3±0.2 pA; change of 0.6%) for this cell. (D) Summary of synaptic weight along time induced by 50 post-pre pairings in control conditions and with JZL184 treatment. 4/13 and 8/9 cells showed significant tLTP in control and in JZL184, respectively. (E) Summary bar graph illustrates that MAGL inhibition allowed tLTP to be expressed, which was CB1R-mediated since prevented by AM251 (3 μM). Representative traces are the average of 15 EPSCs during baseline (black traces) and 50 min after STDP protocol (grey traces). *p<0.05. ns: non-significant.
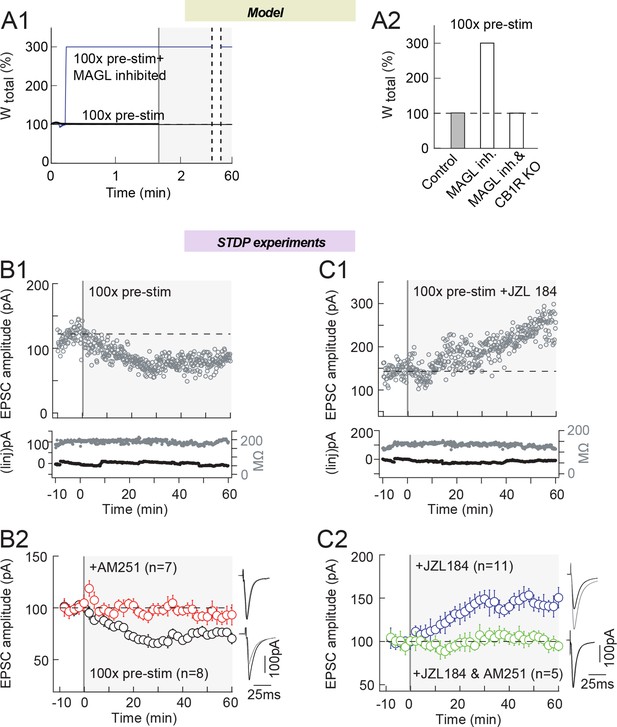
MAGL inhibition shifts eCB-LTD into eCB-LTP, induced by 100 presynaptic stimulations.
(A) Model prediction for the changes in synaptic weight induced by 100 presynaptic stimulations. (A1) In the absence of postsynaptic activity, 100 presynaptic stimulations in the model do not change the synaptic weight in control conditions (full black line), but MAGL inhibition and DAG-Kinase activity (full red line) generates eCB amounts that are large enough to trigger LTP. MAGL and DAG-Kinase inhibition were obtained by fixing the value of the maximal rates of each enzyme to 0 and 5%, respectively, of their default values listed in Supplementary file 1C. (A2) Summary bar graph of the LTP amplitude predicted by the model for 100 presynaptic stimulations in the absence of postsynaptic stimulations. (B) Experimentally, 100 presynaptic stimulations alone (i.e. without paired postsynaptic stimulation) induced significant LTD. (B1) Example of LTD induced by 100 presynaptic stimulations. Top, EPSC strength before and after pairings (before pairings: 118±2 pA; 45–55 min after pairings: 78±1 pA; decrease of 34%). Bottom, time courses of Ri (before, 187±1 MΩ; after, 189±1 MΩ; change of 1.1%) and injected current (Iinj) (before, 2±0.6 pA; after, 3±0.4 pA; change of 0.8%) for this cell. (B2) Summary of LTD induced with 100 presynaptic stimulations; 6/8 cells showed significant LTD. This 2-AG-mediated LTD was prevented by AM251 (3 μM, n=7); 6/7 cells showed no significant plasticity. (C) MAGL inhibition by ZZL184 (1.5 μM) shifts eCB-LTD, induced by 100 presynaptic stimulations, into eCB-tLTP. (C1) Example of LTP induced by 100 presynaptic stimulations with MAGL inhibition. Top, EPSC strength before and after pairings (before pairings: 143±2 pA; 45–55 min after pairings: 224±2 pA; increase of 57%). Bottom, time courses of Ri (before, 131±1 MΩ; after, 131±2 MΩ; no significant change) and injected current (Iinj) (before, -3±1 pA; after, -12±1 pA; change of 6.3%) for this cell. (C2) Summary of LTP induced with 100 presynaptic stimulations; 7/11 cells showed significant LTP. This 2-AG-mediated LTD was prevented by AM251 (3 μM, n=5); 5/5 cells showed no significant plasticity. Representative traces are the average of 15 EPSCs during baseline (black traces) and 50 min after STDP protocol (grey traces). *p<0.05. ns: non-significant.
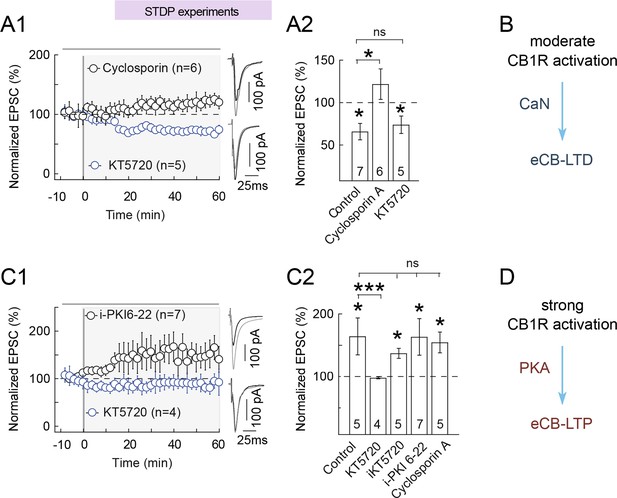
eCB-tLTP maintenance relies on presynaptic PKA activation.
(A-B) eCB-tLTD is CaN-dependent. (A1) Summary of plasticity induced by 100 pre-post pairings with bath applied KT5720 (1 μM) or with cyclosporin (1 μM). eCB-tLTD was prevented with CaN (cyclosporin A) inhibition but unaffected with PKA inhibition (KT5720); 0/6 and 4/5 cells showed LTD with cyclosporin A and KT5720, respectively. (A2) Summary bar graphs illustrate that eCB-LTD maintenance involves the activation of presynaptic CaN by a CB1R-triggered signal and was independent of the activation of presynaptic PKA. (B) Main conclusion scheme: eCB-LTD is triggered by moderate prolonged levels of CB1 activations and requires active CaN. Normality was assumed for the cyclosporine A & KT5720 data (test not passed). (C-D) eCB-tLTP is PKA-dependent. (C1) Summary of plasticity induced by 10 post-pre pairings with bath applied KT5720 (1 μM) or with i-PKI6-22 (20 μM). eCB-tLTP was prevented with bath-applied KT5720 but unaffected with i-PKI6-22, a cell-impermeant PKA inhibitor applied intracellularly in the postsynaptic neuron; 0/4 and 6/7 cells showed LTP with KT5720 and i-PKI6-22, respectively. (C2) Summary bar graphs illustrate that eCB-tLTP depends on presynaptic PKA activation since it was prevented by bath-applied KT5720 but unaffected when KT5720 or PKI6-22, was applied intracellularly in the postsynaptic neuron (i-KT5720, i-PKI6-22). Cyclosporin A had no effect on eCB-tLTP, showing that it was CaN-independent. Thus, eCB-tLTP maintenance involves the activation of presynaptic PKA by a CB1R-triggered signal. Normality was assumed for the ctrl & KT5720 data (test not passed). (D) Main conclusion scheme: eCB-LTP is triggered by large levels of short duration of CB1R activation and requires presynaptic active PKA. Representative traces are the average of 15 EPSCs during baseline (black traces) and 50 min after STDP protocol (grey traces). Error bars represent SD. *p<0.05. ns: not significant.
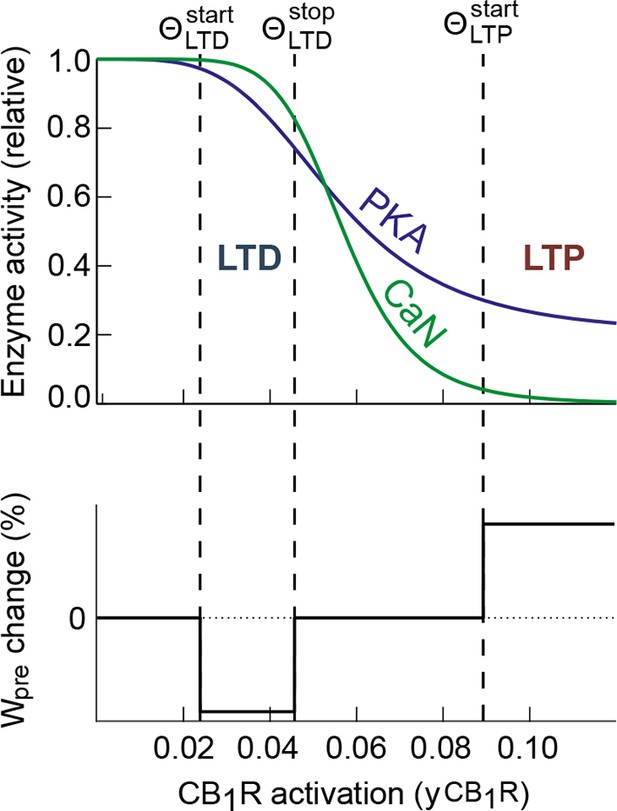
Schematic of a possible mechanism for PKA-CaN control of eCB-dependent bidirectional STDP.
CB1R is a Gαi GPCR which activation by eCB reduces the activity of PKA. In the striatum, CB1R activation also inhibits presynaptic VSCC, which is expected to reduce presynaptic calcium and, potentially, calcium-activated calcineurin. Therefore, CB1R activation may reduce both PKA and CaN activity. The top panel gives a schematic illustration of this effect in a case where the kinetic parameters are such that the ratio between PKA and CaN activity changes when CB1R activation increases: CaN/PKA > 1 for intermediate CB1R activation and switches to CaN/PKA < 1 for large activation. Our experimental data for eCB-dependent STDP and its control by CB1R activation (bottom) are compatible with the hypothesis that eCB-LTD would be expressed when CaN/PKA > 1 whereas CaN/PKA < 1 leads to eCB-LTP.
Additional files
-
Supplementary File 1
Parameters of the mathematical model
- https://doi.org/10.7554/eLife.13185.017
-
Supplementary File 2
Modeling smooth thresholds for eCB-dependent plasticity
- https://doi.org/10.7554/eLife.13185.018