Body side-specific control of motor activity during turning in a walking animal
Figures
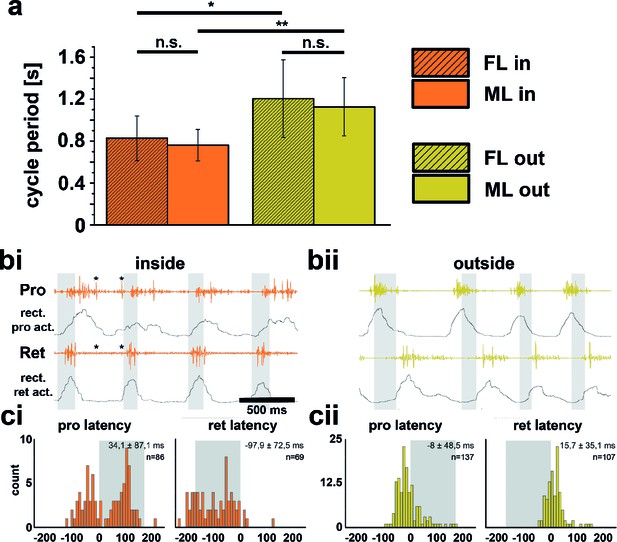
Differences between inside and outside middle leg (ML) activity in the intact tethered stick insect that is turning on the slippery surface.
(a) Significant difference between the periods of inside (orange) and outside (yellow) turning of the front (striped) and middle (solid) leg, and no significant differences between the periods of front and middle leg stepping on either the inside or the outside; asterisks mark significance levels: * p<0.05, ** p>0.01. (bi–ii) Original and rectified EMG traces of protractor (Pro) and retractor coxae (Ret) with stance and swing (grey shaded bars) monitored electrically. The example in bi (orange) shows examples of backward steps during inside stepping with the retractor being active mostly in swing. Asterisks (*) mark very weak crosstalk between the two channels; bii (yellow), original and rectified EMG traces of the same muscles during outside steps. Here the retractor is always active in stance. ci and cii. Protractor and Retractor latency of the first EMG spike during inside (ci, orange) and outside (cii, yellow) steps from N=5 animals. Note the bimodal distribution during inside steps due to the occurrence of forward and backward steps.
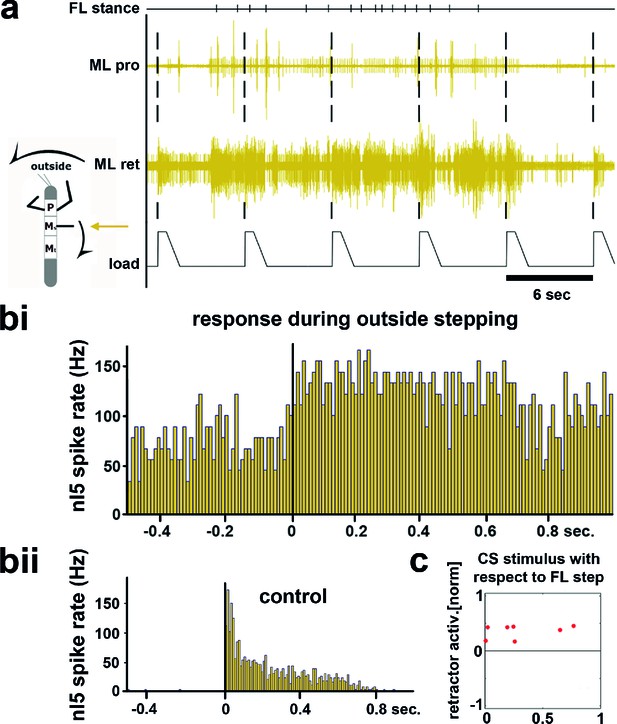
Influence of local load stimuli on mesothoracic motor activity during outside turning.
(a) Mesothoracic pro- and retractor activity (2nd and 3rd row, resp.) before, during and after a front leg outside walking sequence (FL stance begin marked in top row event channel) and simultaneous application of load stimuli to the mesothoracic leg stump (bottom trace). (bi) Peristimulus time histogram (PSTH) showing the increase in mesothoracic retractor MN activity in response to local load stimuli during FL outside stepping; note the increased background activity. (bii) PSTH of retractor activity timed to the begin of the CS stimulus ramp in the quiescent animal (control). (c) Increased retractor MN activity upon CS stimuli did not depend on the phase of the CS stimulus within the FL step cycle during outside steps for the animal shown in a and b; 0 on the y axis marks the normalized baseline response in the 100ms before the CS stimulus. Figure 2—figure supplement 1 shows the same data for all 13 animals. FL: front leg, ML: middle leg, pro: protractor, ret: retractor.
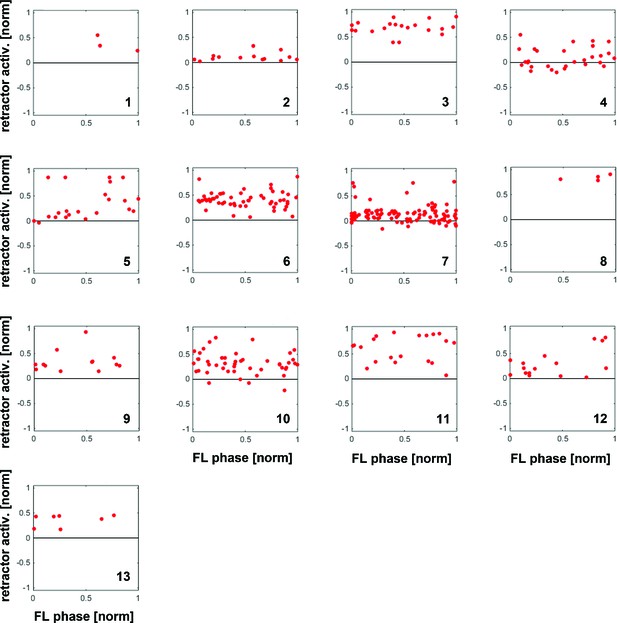
Distribution of response amplitudes of retractor MNs to CS stimuli throughout the FL step cycle during outside steps from all 13 animals, normalized to the activity in the 100ms preceding the CS stimulus.
Retractor activity mostly increased over already ongoing retractor activity without any phase preference.
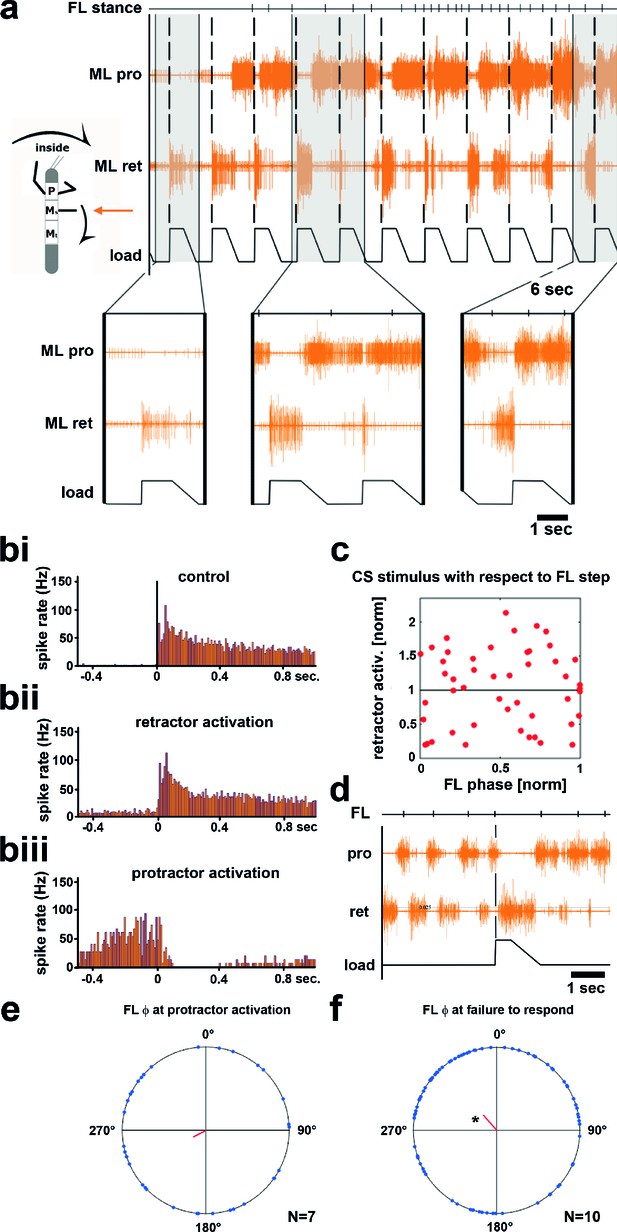
Influence of local load stimuli on mesothoracic motor activity during inside turning.
(a) Mesothoracic pro- and retractor activity (2nd and 3rd row, resp.) before and during a front leg inside walking sequence (front leg [FL] stance begin marked in top row event channel), and simultaneous application of load stimuli to the mesothoracic leg stump (bottom trace). Four example responses (grey shaded areas) with expanded time resolution are shown below, the first one during quiescence, the other three during inside steps of the ipsilateral FL. (bi–iii) PSTHs showing the forms of mesothoracic retractor MN response to local load stimuli during FL inside stepping: bi, peristimulus time histogram (PSTH) of retractor activity timed to the begin of the CS stimulus ramp in the quiescent animal. bii, retractor activation upon load stimulus; biii, termination of retractor activity (activation of protractor activity is not shown as PSTH). (c) Distribution of response strength of retractor MNs to CS stimuli throughout the FL step cycle during inside steps with retractor activation from the animal shown in a; note that there is no phase preference for either an increase or a decrease in retractor activation compared to controls, this was also found for all other animals (Figure 3—figure supplement 1). (d) Example for no response to a loading stimulus. (e) Phase plot showing the distribution of CS stimuli in the FL step cycle that lead to protractor activation from N=7 animals; no significant phase preference was detectable. (f) Phase plot showing the distribution of CS stimuli in the FL step cycle that did cause neither re- nor protractor activation from N=10 animals; a significant phase preference was detectable at 0.89. FL: front leg, ML: middle leg, pro: protractor, ret: retractor.
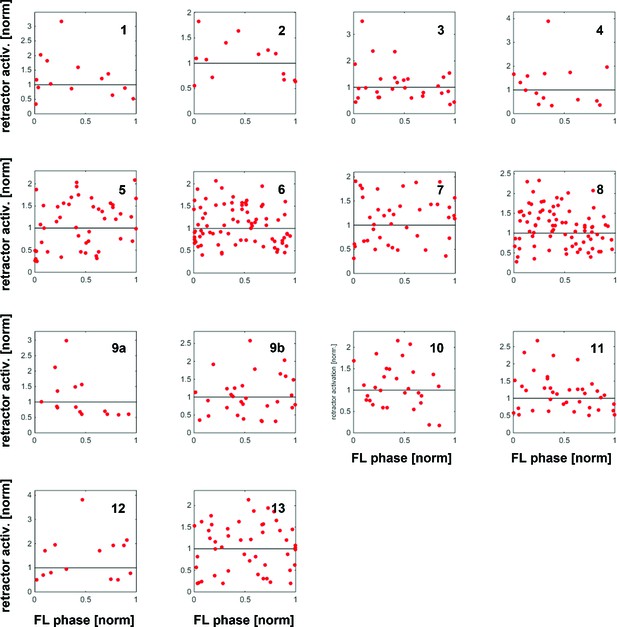
Distribution of response amplitudes of retractor MNs to CS stimuli throughout the FL step cycle during inside steps with retractor activation from all 13 animals (note that 9a and 9b constitute data from two files from one animal).
Maximum retractor activation in a time window of 150 ms after CS stimulation was normalized to the average maximum response during control stimulations.
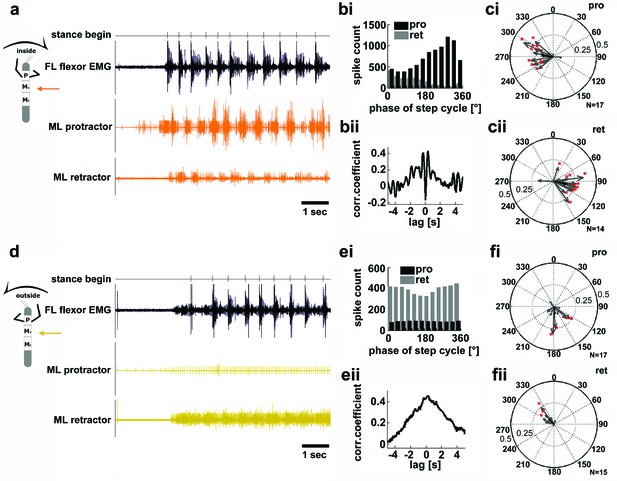
Motor output of the protractor and retractor coxae motor neuron pools in the deafferented mesothoracic ganglion during front leg inside and outside stepping.
(a) Flexor EMG recording of the front leg (FL, top trace, black) together with simultaneous recording of ipsilateral mesothoracic protractor and retractor nerve activity (2nd and 3rd row, resp., orange) during an inside stepping sequence. (bi) Phase histogram of protractor and retractor activity with respect to the step cycle of the FL. bii. Cross correlation function of protractor and retractor activity. (c) Polar plots of directionality vectors with respect to the step cycle of the ipsilateral FL for protractor (ci) and retractor (cii) from N=17 and N=14 animals, resp.. (d) Flexor EMG recording of the FL (top trace, black) together with simultaneous recording of ipsilateral mesothoracic protractor and retractor nerve activity (2nd and 3rd row, resp., yellow) during an outside stepping sequence from the same animal as in a. (ei) Phase histogram of protractor and retractor activity with respect to the step cycle of the FL. (eii) Cross correlation function of protractor and retractor activity. (f) Polar plots of directionality vectors with respect to the step cycle of the ipsilateral FL for protractor (fi) and retractor (fii) from N=17 and N=15 animals, resp..
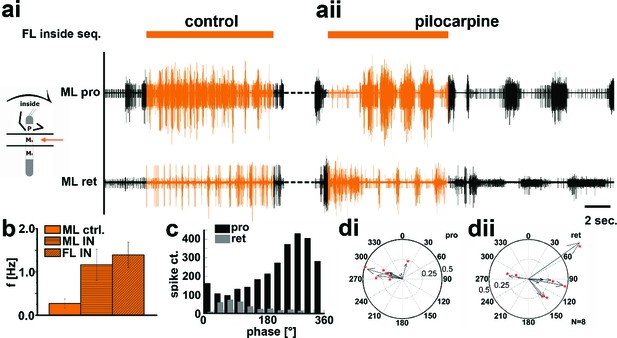
Motor output of the protractor and retractor coxae motor neuron pools in the deafferented control, and while the mesothoracic ganglion was superfused with a pilocarpine solution during front leg (FL) inside stepping in split-bath configuration.
(a) Traces show the time with and without FL activity (top trace) together with simultaneous mesothoracic protractor and retractor nerve recordings (2nd and 3th row, resp.) ipsilateral to the FL, in control conditions (ai) and with pilocarpine on the mesothoracic ganglion (aii) of the same animal. (b) frequency of the mesothoracic pilocarpine rhythm in the quiescent animal (clear bar), and during inside steps of the FL (horizontally shaded bar) from N=11 animals. In addition, diagonally shaded bar shows the frequency of the stepping FL from the eight animals where the FL EMG was recorded. In all 11 cases the frequency of the pilocarpine rhythm during stepping sequences was significantly (p<0.001) increased over that in the quiescent animal. (c) Phase histogram of protractor and retractor activity from the same animal with respect to the step cycle of the FL during pilocarpine superfusion. (d) Polar plots with directionality vectors of protractor (di) and retractor (dii) with respect to the step cycle of the ipsilateral FL when the mesothoracic ganglion was superfused with pilocarpine (N=8 animals).
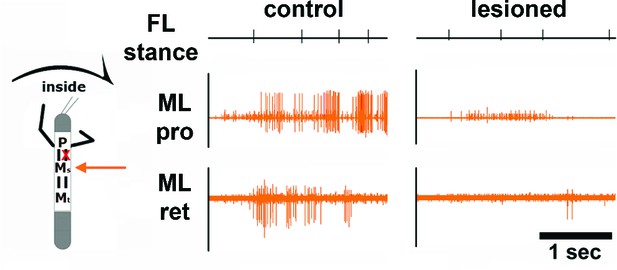
Effect of unilateral transsection of the connective between the pro- and mesothoracic ganglion during inside stepping of the ipsilateral front leg (FL).
Left panel: Activity in the mesothoracic ganglion during FL inside steps in the intact animal. Right panel: Activity in the mesothoracic ganglion during FL inside steps in the same animal after lesion of the connective. The residual activity after lesion is due to the activity of breathing MNs. Events mark stance begin in the ipsilateral FL.
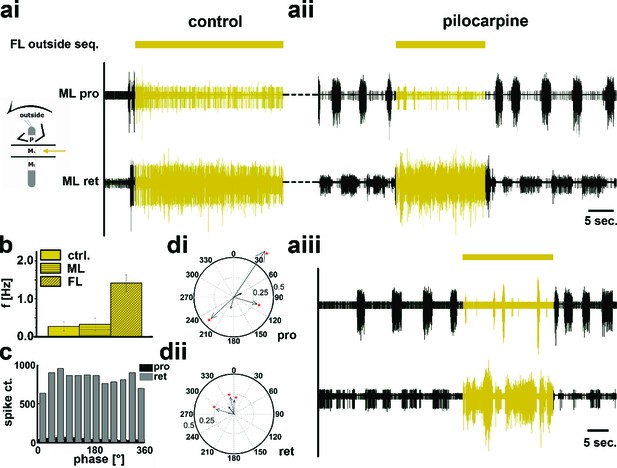
Motor output of the protractor and retractor coxae motor neuron pools in the deafferented control and when the mesothoracic ganglion was superfused with a pilocarpine solution during front leg (FL) outside stepping in split-bath configuration.
(a) Traces show the time with and without FL activity (top trace) together with simultaneous mesothoracic protractor and retractor nerve recordings (2nd and 3th row, resp.) ipsilateral to the FL, in control conditions (ai) and with pilocarpine on the mesothoracic ganglion of the same animal (aii), and in a 2nd animal (aiii). (b) Frequency of the mesothoracic pilocarpine rhythm in the quiescent animal (clear bar) and during inside steps of the FL (horizontally shaded bar) from N=8 animals. In addition, the diagonally shaded bar shows the mean FL stepping frequency from the five animals where the EMG was recorded. Note that except for 2 animals the frequency of the pilocarpine rhythm during stepping sequences was not significantly different from control in the quiescent animal. (c) Phase histogram of protractor and retractor activity with respect to the step cycle of the front leg during pilocarpine superfusion from the animal in aii. (d) Polar plots with directionality vectors of protractor (di) and retractor (dii) with respect to the step cycle of the ipsilateral FL when the mesothoracic ganglion was superfused with pilocarpine (N=5 animals).
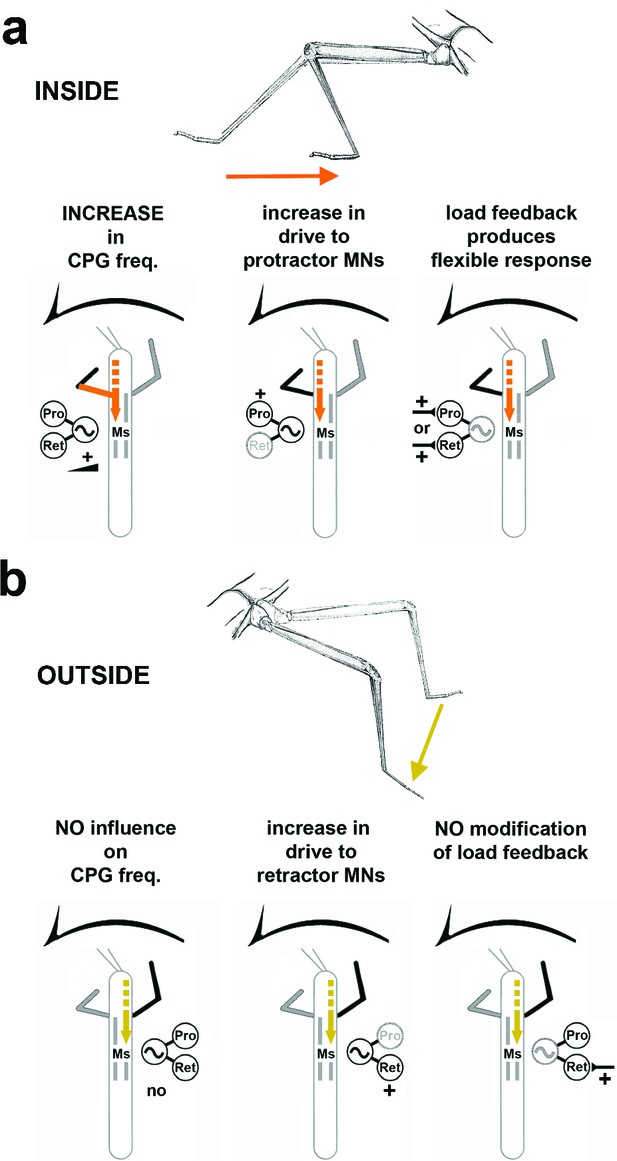
Summary of the observed effects on mesothoracic motor output and potential sources for the influences.
(a) Effects observed in the mesothorax ipsilateral to the INSIDE front leg. (b) effects observed in the mesothorax ipsilateral to the OUTSIDE front leg. Note that solid lines mark demonstrated origin through lesion experiments, while broken lines mark suggested probable origin from higher order brain regions in the head, as discussed.