Regulation of two motor patterns enables the gradual adjustment of locomotion strategy in Caenorhabditis elegans
Figures
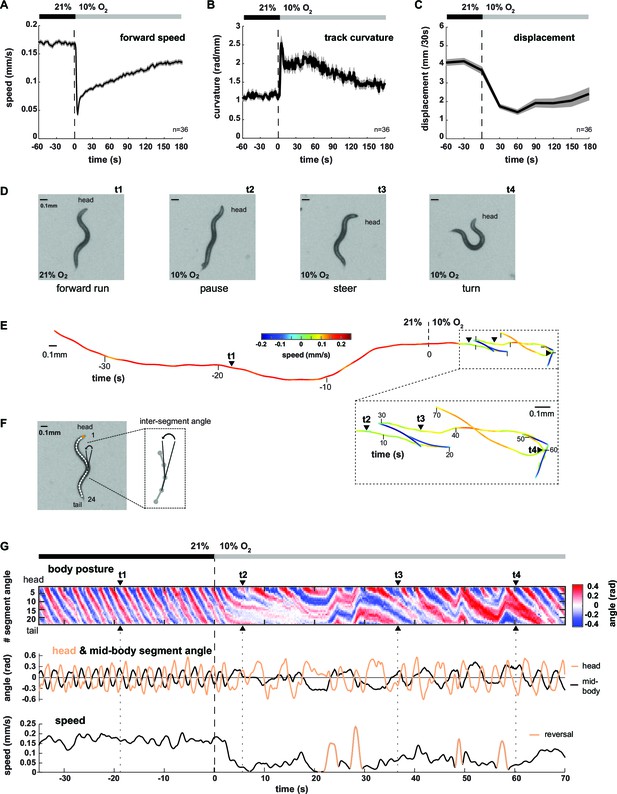
Worms transit from LDT to ARS after oxygen-sensory stimulation.
(A–C) Locomotion parameters from population assays of wild type (N2) worms stimulated with O2 downshifts from 21% to 10%. Traces show mean, shadings show SEM. n = 36 populations assays (~25 animals per assay). Time is relative to O2 downshift (A) Forward movement speed based on centroid coordinates (1 s binning). (B) Curvature of forward moving trajectories (1 s binning). (C) Centroid displacement between 30 s intervals. (D–G) Representative example of a wild type (N2) worm. t1-4 indicate corresponding time points. Time is relative to O2 downshift. (D) Single video frames: (t1) forward run at 21% O2, (t2) pause after O2 downshift, (t3) shallow and (t4) deep turning maneuver. (E) Trajectory of centroid position for 35 s at 21% and 70 s at 10% O2, respectively. Color indicates crawling speed; reverse movement is shown as negative speed. (F) Worm image overlaid with a skeleton. The inset shows how inter-segment angles were calculated. (G) Top: Body posture kymograph of 24 inter-segment angles from head (1) to tail (24). Middle: time course of representative head (#2) and mid-body angle (#11). Bottom: translational speed of the worm’s centroid. Reverse movement is indicated by color.
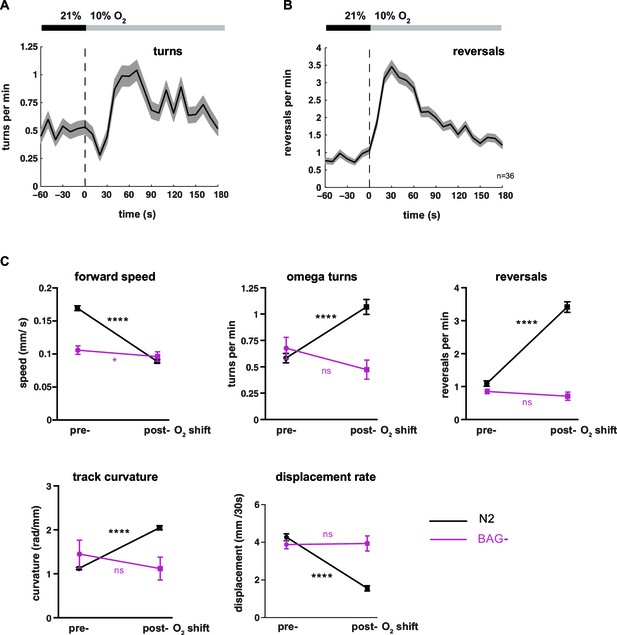
Behavioral transition from LDT to ARS after oxygen downshift depends on oxygen-sensory neurons BAG.
(A–B) Locomotion parameters from population assays of wild type (N2) worms stimulated with O2 downshifts from 21% to 10%. Traces show mean, shadings show SEM. Data bins are 10 s. n = 36 populations assays (~25 animals per assay). Time is relative to O2 downshift. (A) Omega turns and (B) reversals. (C) Quantifications of behavioral parameters presented in (A, B) and Figure 1A–C. Means ± SEM of wild type N2 (n = 36 assays) and BAG- worms (genetic cell ablation, n = 6 assays). Significance values are determined by paired t-tests (****p≤0.0001, *0.01<p≤0.05, ns p>0.05). Note that BAG-ablated worms (strain CX11697) additionally lack the ASG sensory neurons (Roger Pocock, pers. communication).
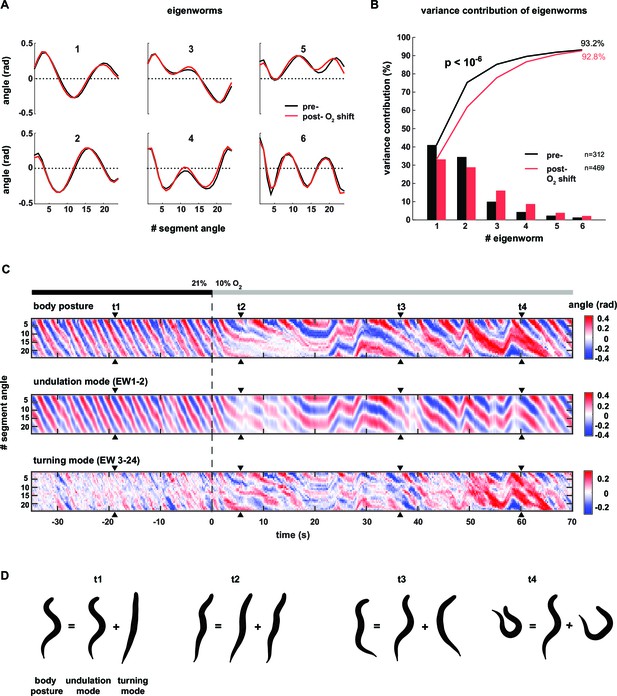
Decomposition of the body posture into undulation and turning motor patterns.
(A) The panels show the top six of 24 eigenworms (EWs) calculated from pooled segment angle time series of wild type (N2) worms. Black and red EWs are calculated from 60s intervals pre (n = 312) or post- (n = 469) O2 downshift respectively. (B) Bars show the variance explained by the EWs shown in A. Traces show cumulative values. The p-value was determined by a random resampling approach (see Materials and methods) and estimates an upper boundary of the probability that the difference between the cumulative variance distributions would occur by chance. (C) Example kymographs from the same worm shown in Figure 1D–G. Time is relative to O2 downshift. Arrowheads indicate time points t1-4 as in Figure 1. Top: body posture as in Figure 1G. Middle: undulation mode reconstructed from EW 1–2. Bottom: turning mode reconstructed from EW 3–24. (D) Worm shapes graphically reconstructed from body posture, undulation mode and turning mode at time points t1-4. Note that the worm’s posture is a linear sum of undulation and turning mode.

Characterization of undulation and turning modes by signal cross-correlation analysis.
Undulation and turning modes during forward movement from wild type N2 time series (n = 36 assays, ~25 animals per assay, yielding to n = 9358 time series > = 20 s duration) were analyzed by cross-correlation. Traces display mean ± standard deviation. Maximum absolute correlation and corresponding lag times are indicated. (A–D) Intra-mode cross-correlation between segment angles of head (segment angle #2) and mid-body (segment angle #11) (A, B) or head and posterior body (segment angle #19) (C, D), for undulation (A, C) or turning mode (B, D). (E,F) Inter-mode cross-correlation between undulation and turning mode for (E) head and (F) mid-body segment angle.
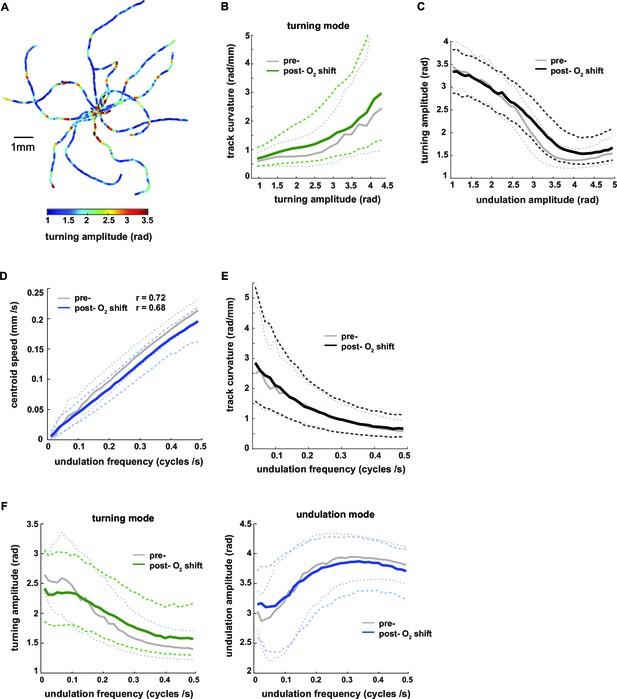
Turning amplitude is reciprocally regulated with undulation amplitude and undulation frequency.
(A) Exemplary worm trajectories during the 10% O2 interval aligned at their starting points with color code indicating turning amplitude. (B–F) The graphs show interdependencies of the indicated locomotion parameters during forward movement. Solid lines show the medians and dashed lines show interquartile ranges on the y–axis. Data are binned by the corresponding values on the x-axis. Tick marks indicate bin medians of the x-axis. Data from wild type N2 worms (n = 36 assays, ~25 animals per assay) during 3 min intervals pre- (gray) and post- (black or color) O2 downshift were analyzed. Very high values on the x-axis are omitted due to data sparseness. (B) Track curvature versus turning amplitude. Bin width = 0.2 rad. (C) Turning amplitude versus undulation amplitude. Bin width = 0.1 rad. (D) Centroid speed versus undulation frequency. Bin width = 0.015 cycles/s. Pearson correlation coefficients are indicated. (E) Track curvature versus undulation frequency. Bin width = 0.015 cycles/s. (F) Turning amplitude (left) or undulation amplitude (right) versus undulation frequency. Bin width = 0.015 cycles/s.
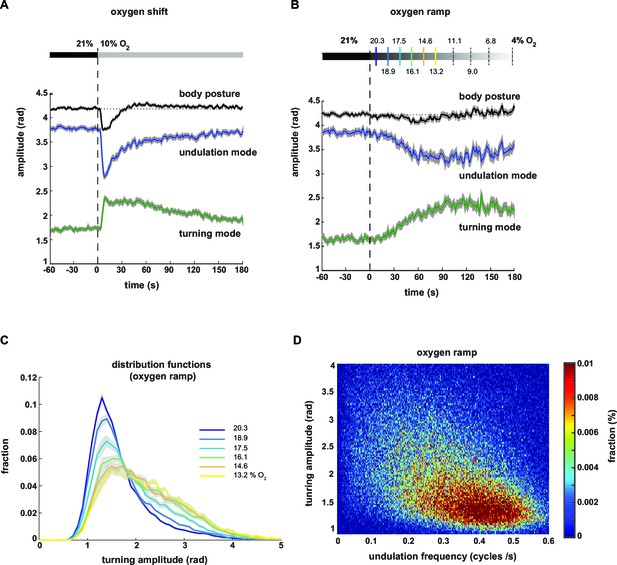
Undulation amplitude and turning amplitude are inversely and gradually regulated in response to O2 stimulation.
(A,B) Behavioral responses of wild type N2 worms during forward movement evoked by decreasing O2 concentrations. Traces show mean amplitude of body posture (black), undulation mode (blue) and turning mode (green); shadings indicate SEM. Time is relative to onset of O2 concentration change. (A) O2 downshift from 21% to 10% O2 (n = 36 assays, ~25 animals each) and (B) gradual O2 ramp from 21 to 4% O2 (n=15 assays, ~25 animals each). Some O2 concentrations during the ramp are indicated on top. Colors correspond to (C). (C) Trial-mean (± SEM) fractional distribution functions of turning amplitude from the same data shown in (B). All distributions are calculated from consecutive 15 s intervals during the ramp with amplitude bin width of 0.1 rad. The legend indicates the median O2 concentration during each interval. Color code corresponds to (B). (D) 2D density map showing the fractional distribution of forward undulation frequency versus turning amplitude from all worms (n ≈ 375) during the ramp assays shown in (B, C). Data from -60 s to 180 s were included. Bin width are 0.002 cycles/s and 0.025 rad. The y- and x-axes omit very high values due to data sparseness.
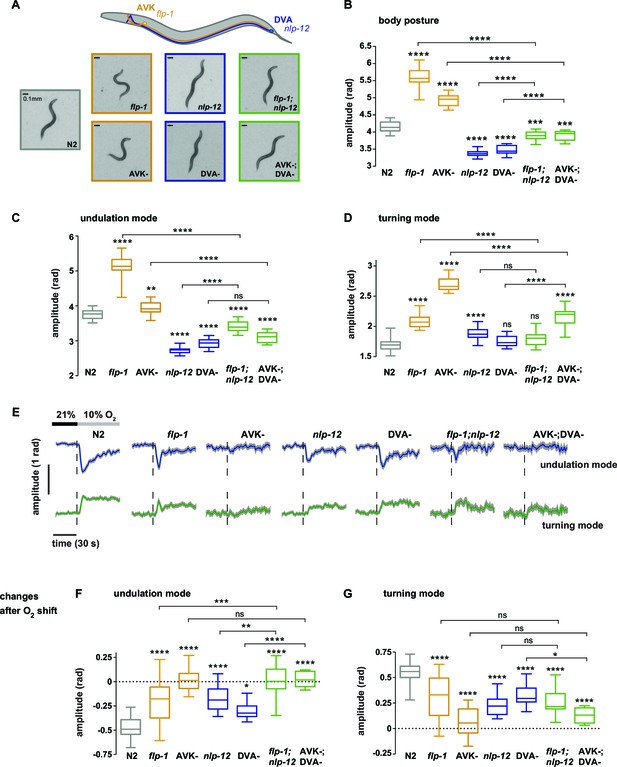
Regulation of undulation mode and turning mode through antagonistic peptidergic interneurons.
(A) Top: Anatomical sketch of interneurons AVK and DVA. All other panels show representative video frames during forward movement at 21% O2 of worms with indicated genotypes. flp-1 and nlp-12 are loss of function mutations. AVK- and DVA- denote transgenes driving caspase expression leading to cell death in the respective neuron. Note the effects of genotypes on body posture. Heads are pointing upwards. (B–D) Boxplots of amplitudes during forward movement measured during a 4 min interval at 21% O2 of the (B) body posture, (C) undulation mode and (D) turning mode. (E) Time profiles of mean undulation amplitude (blue) and mean turning amplitude (green). Shadings show SEM. Dashed lines indicate onset of O2 downshift. The traces are vertically aligned to emphasize differences in behavioral responses. The vertical bar indicates amplitude; the horizontal bar indicates time axis. (F, G) Boxplots showing changes of (F) undulation amplitude and (G) turning amplitude in response to the O2 downshift. Changes were calculated by subtracting the means of 60s intervals post-downshift from the means of 60s pre-downshift intervals. Boxplots in all panels display trial-median and interquartile range with 5–95 percentile whiskers. Asterisks on top of box plots indicate significance levels compared to wild type N2 and asterisks on top of brackets indicate significance levels for selected comparisons, using one-way-ANOVA with Sidak’s correction for multiple comparisons (****p≤0.0001, ***0.0001<p≤0.001, **0.001<p≤0.01, *0.01<p≤0.05, ns p>0.05). Number of experiments (~25 animals per assay): N2 n = 36, flp-1 n = 29, AVK- n = 21, nlp-12 n = 21, DVA- n = 13, flp-1;nlp-12 n = 12 and AVK-;DVA- n = 10.
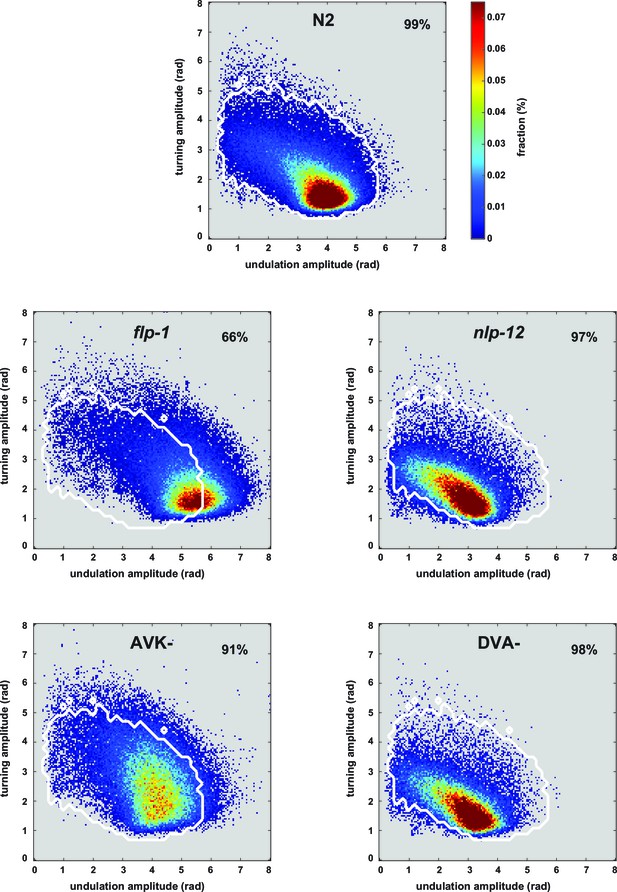
Postures of manipulated animals with mis-regulated motor patterns largely lie within the wild type N2 posture space.
2D density maps of the indicated strains showing the fractional distribution of forward undulation amplitude versus turning amplitude from all worms during the shift assays shown in Figure 5E. Data from -60 s to 60 s respective to the shift are included. Bin widths are 0.05 rad. White boundary encompasses 99% of wild type N2 fractional data. The fractions of data that lie within this boundary are indicated. Except for flp-1 mutants, >90% of the posture data are within the 99% posture space of wild type animals. This indicates that the postures of mutants and manipulated strains largely reflect postures that are exhibited, however less frequently, by wild type animals. Postures of flp-1 mutants that are outside the wild type profile exhibit mostly an increase in undulation amplitude.
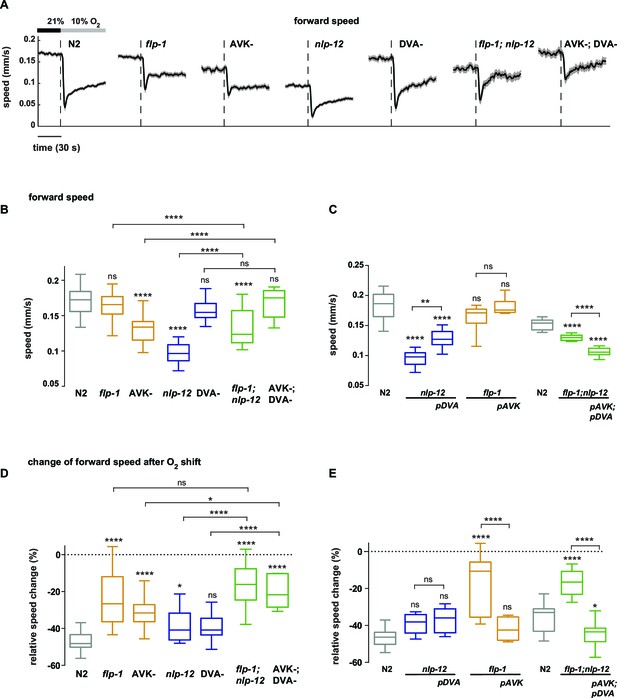
Regulation of locomotion speed through antagonistic peptidergic interneurons.
(A) Time profiles of mean forward locomotion speed. Shadings show SEM. Dashed lines indicate onset of O2 downshift. The horizontal bar indicates time axis. (B–E) Boxplots of forward locomotion speed (B, C) measured during a 4 min interval at 21% oxygen and (C, D) of the percent change in response to the oxygen downshift. Changes were calculated by subtracting the means of 60 s intervals post-downshift from the means of 60 s pre-downshift intervals divided by the means of the interval pre-downshift. Genotypes indicate transgenic rescue using cell specific promoters (pDVA, pAVK). Boxplots display trial-median, interquartile range and 5–95 percentile whiskers. Asterisks on top of box plots indicate significance levels compared to wild type N2 and asterisks on top of brackets indicate significance levels for comparisons with the respective mutant or selected genotypes, using one-way-ANOVA with Sidak’s correction for multiple comparisons (****p≤0.0001, ***0.0001<p≤0.001, **0.001<p≤0.01, *0.01<p≤0.05, ns p>0.05). Number of experiments (each ~25 animals): (B, D) N2 n = 36, flp-1 n = 29, AVK- n = 21, nlp-12 n = 21, DVA- n = 13, flp-1;nlp-12 n = 12 and AVK-;DVA- n = 10. (C, E) N2 n = 10, nlp-12 n = 6, pDVA: nlp-12 rescue under Pnlp-12 n = 11, flp-1 n = 11, pAVK: flp-1 rescue under Pflp-1 fragment n = 9, N2 n = 11, flp-1;nlp-12 n = 11, pAVK;pDVA: flp-1 rescue under Pflp-1 fragment & nlp-12 rescue under Pnlp-12 n = 11.

Gradual behavioral transitions depend on flp-1 and nlp-12 neuropeptide genes.
(A) Behavioral responses of flp-1; nlp-12 mutant worms during forward movement evoked by a gradual O2 ramp from 21 to 4% (n = 15 assays ~25 animals each). Traces show mean amplitude of body posture (black), undulation mode (blue) and turning mode (green); shadings indicate SEM. Time is relative to onset of O2 ramp. Some O2 concentrations during the ramp are indicated on top. Colors correspond to (B). (B) Trial-mean (± SEM) of fractional distribution functions of turning amplitude from the same data shown in (A). All distributions are calculated from consecutive 15 s intervals during the ramp with amplitude bin width of 0.1 rad. The legend indicates the median O2 concentration during each interval. Color code corresponds to (A).
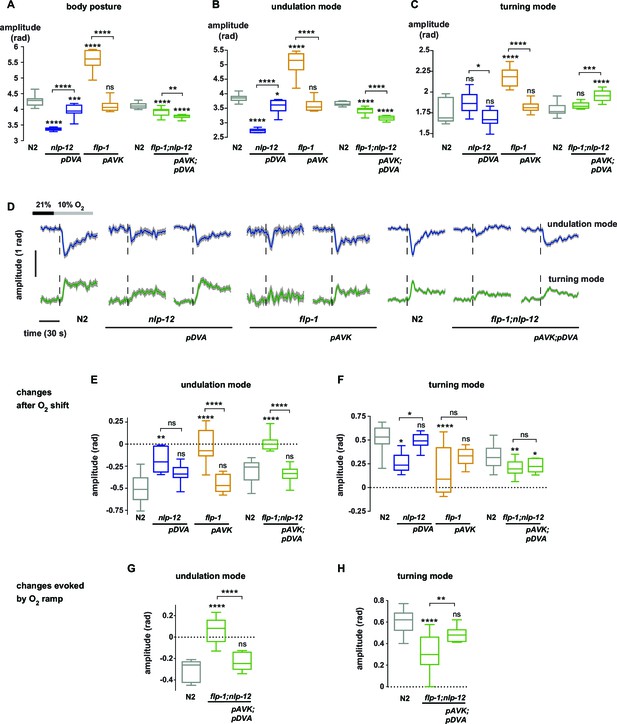
Peptidergic regulation of undulation and turning mode by flp-1 and nlp-12 through interneurons AVK and DVA.
(A–C) Boxplots of amplitude during forward movement measured during a 4 min interval at 21% O2 of the (A) body posture (B) undulation mode and (C) turning mode. (D) Time profiles of mean undulation amplitude (blue) and mean turning amplitude (green). Shadings show SEM. Dashed lines indicate onset of O2 downshift. The traces are vertically aligned to emphasize differences in behavioral responses. The vertical bar indicates amplitude; the horizontal bar indicates time axis. (E–H) Boxplots showing changes of undulation amplitude (E, G) and turning amplitude (F, H) in response to the O2 downshift (E, F) or to an O2 ramp (G, H). Changes were calculated by subtracting the means of 60 s intervals post-downshift from the means of 60 s pre-downshift intervals (E, F) or by subtracting the means of 60 s intervals at the end of the ramp from the means of 60 s intervals before ramp onset (G, H). Genotypes indicate transgenic rescue using cell specific promoters (pDVA, pAVK). Boxplots in all panels display trial-median, interquartile range and 5–95 percentile whiskers. Asterisks on top of box plots indicate significance levels compared to wild type N2 and asterisks on top of brackets indicate significance levels for comparisons with the respective mutant, using one-way-ANOVA with Sidak’s correction for multiple comparisons (****p≤0.0001, ***0.0001<p≤0.001, **0.001<p≤0.01, *0.01<p≤0.05, ns p>0.05). Number of experiments (~25 animals per assay): N2 n = 10, nlp-12 n = 6, pDVA: nlp-12 rescue under Pnlp-12 n = 11, flp-1 n = 11, pAVK: flp-1 rescue under Pflp-1 fragment n = 9, N2 n = 11, flp-1;nlp-12 n = 11, pAVK;pDVA: flp-1 rescue under Pflp-1 fragment & nlp-12 rescue under Pnlp-12 n = 11.
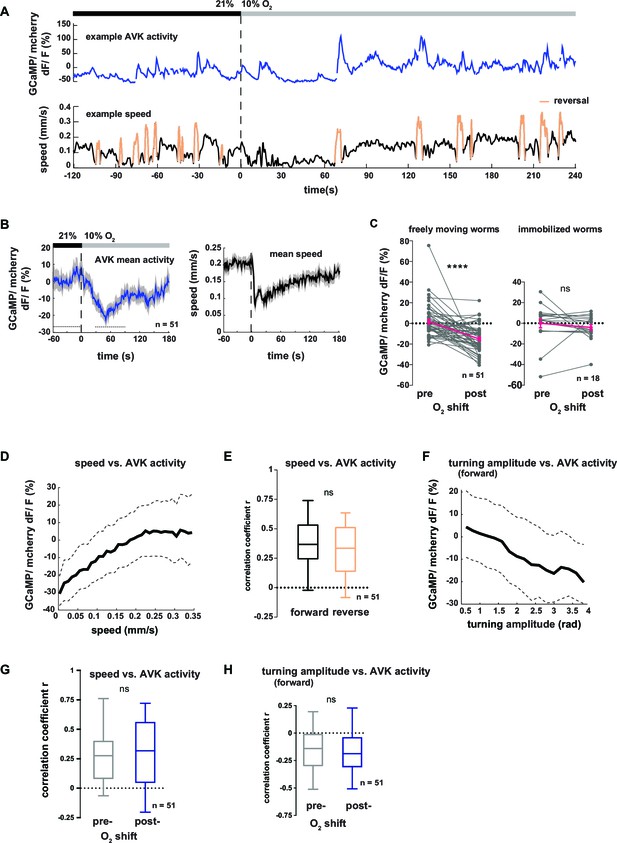
Neural activity of interneuron AVK reflects locomotion speed and suppression of turning motions.
(A–H) Neural activity of AVK neurons was measured in freely moving worms by calcium imaging and is displayed as normalized ratio of GCaMP5K/ mCherry fluorescence. (A) Exemplary recording of neural activity (top) and locomotion speed (bottom). Time is relative to O2 downshift. Periods of reverse movement are labeled in orange. (B) Mean AVK activity (left) and mean locomotion speed (right). Shadings indicate SEM. Time is relative to O2 downshift. n = 51 worms. (C) AVK neural activity changes in response to O2 downshift compared between freely moving worms and worms immobilized in a microfluidic device. Quantifications of mean activity during 60 s intervals pre- and post- O2 downshift indicated by dashed lines in (B). Values from single recordings are shown in gray and population means ± SEM are shown in magenta. AVK activity significantly decreased in freely moving animals, but did not change in immobilized worms (****p≤0.0001; ns, p=0.34, paired t-test). (D) Median (solid line) and interquartile range (dashed lines) of AVK activity versus binned locomotion speed (bin size = 0.01 mm/s). (E) Box plots of linear correlation coefficients between forward or reverse movement speed and AVK activity calculated for each recording (n = 51). Unpaired t-test shows no significant difference (ns, p=0.13). (F) Median (solid line) and interquartile range (dashed lines) of AVK activity during forward movement versus binned turning amplitude (bin size = 0.2 rad). (G,H) Box plots of linear correlation coefficients calculated from time intervals pre- and post- O2 downshift (n = 51 each). Paired t-tests show no significant differences. (G) Locomotion speed versus AVK activity; 2 min intervals (ns, p = 0.89). (H) Turning amplitude during forward movement versus AVK activity, 4 min intervals (ns, p=0.56). All boxplots display median, interquartile range and 5–95 percentile whiskers.
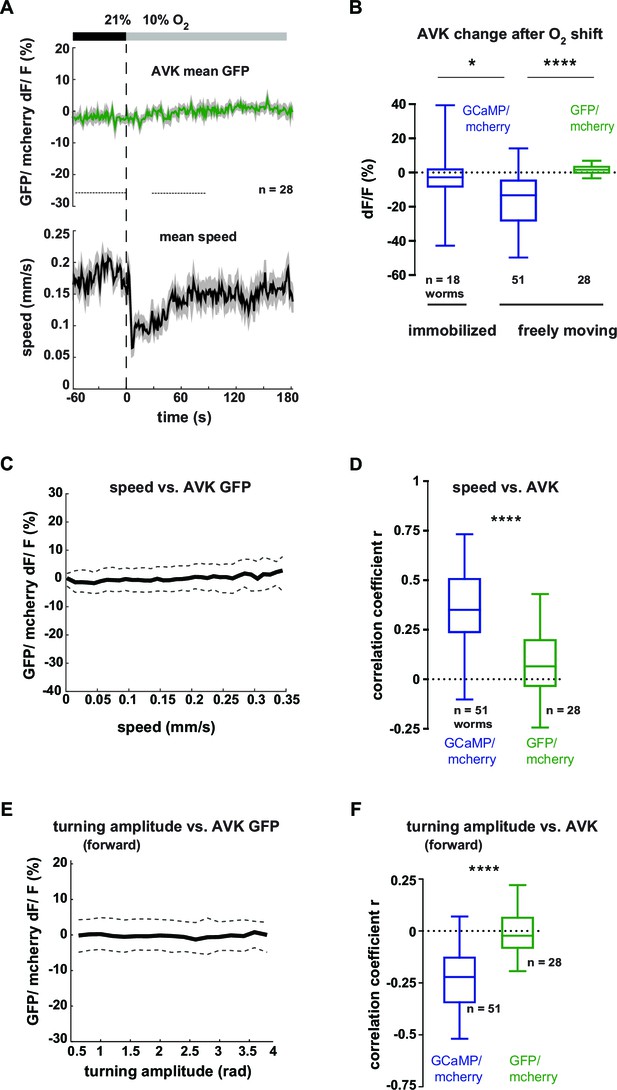
Neural activity changes of interneuron AVK are not derived from motion artifacts.
(A–F) Neural activity of AVK neurons was measured by calcium imaging and is displayed as normalized ratio of GCaMP5K/mCherry fluorescence or for control calcium-insensitive GFP/mCherry. (A) Mean AVK ratio (top) and mean locomotion speed (bottom) from GFP-expressing animals. Shadings indicate SEM. Time is relative to O2 downshift. n = 28 worms. (B) Boxplots showing changes of AVK ratio in response to the O2 downshift compared between freely moving worms expressing GCaMP or GFP and worms immobilized in a microfluidic device. Changes were calculated by subtracting the means of 60 s intervals post-downshift from the means of 60 s pre-downshift intervals, indicated by dashed lines in (A). Significantly smaller ratio changes of immobilized worms (*p = 0.015) or control GFP-expressing worms (****p<0.0001, one-way-ANOVA with Dunnett’s correction for multiple comparisons) compared to GCaMP-expressing freely moving worms. (C) Median (solid line) and interquartile range (dashed lines) of AVK GFP/mCherry ratio versus binned locomotion speed (bin size = 0.01 mm/s). (D) Box plots of linear correlation coefficients between speed and AVK ratio calculated for each recording (n as indicated). Total recordings of GCaMP-expressing worms show significantly stronger correlation than GFP-expressing worms (unpaired t-test ****p<0.0001). (E) Median (solid line) and interquartile range (dashed lines) of AVK ratio versus binned turning amplitude (bin size = 0.2 rad) during forward locomotion. (F) Box plots of linear correlation coefficients between turning amplitude and AVK ratio during forward movement calculated for each recording. GCaMP-expressing worms have significantly stronger correlation than GFP-expressing worms (unpaired t-test ****p<0.0001). Boxplots display median, interquartile range and 5–95 percentile whiskers.
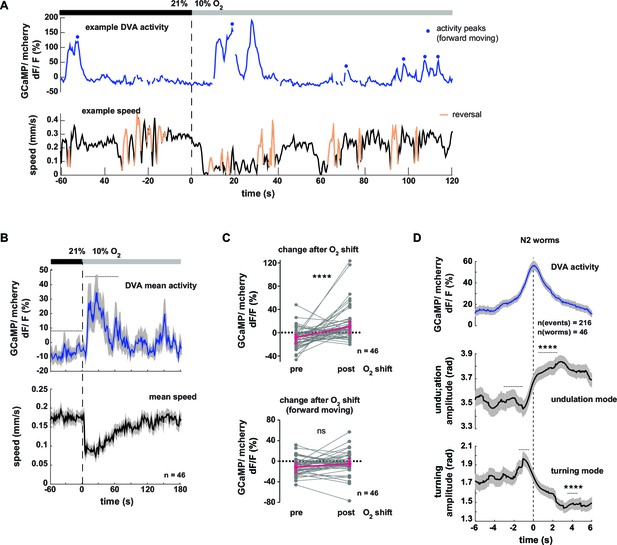
Neural activity of interneuron DVA is associated with the amplitude of undulation motions.
(A–D) Neural activity of DVA neurons was measured by calcium imaging in freely moving animals and is displayed as normalized ratio of GCaMP5K/mCherry fluorescence. (A) Exemplary recording of neural activity (top) and locomotion speed (bottom). Time is relative to O2 downshift. Periods of reverse movement are labeled in orange. Peaks in DVA calcium transient during forward displacement are marked by blue dots. (B) Mean DVA activity (top) and mean locomotion speed (bottom). Shadings indicate SEM. Time is relative to O2 downshift. n = 46 worms. (C) DVA neural activity changes in response to O2 downshift using all data (top) or only during forward displacement, i.e. excluding reverse movement and pause phases (bottom). Quantifications of mean activity during 60s intervals pre- and post- O2 downshift indicated by dashed lines in (B). Values from single recordings are shown in gray and population median ± interquartile range are shown in magenta. DVA activity significantly increases upon O2 downshift, but not when animals are moving forward (****p<0.0001; ns, p=0.12, Wilcoxon matched-pairs signed rank test). (D) Traces show mean undulation amplitude and turning amplitude triggered to DVA activity peaks (t = 0 s) during forward displacement (see blue dots in (A) for example events). Shadings show SEM. Number of events and worms is indicated. Wilcoxon matched-pairs signed rank tests indicate significant changes (****p≤0.0001) between 1 s or 2 s intervals pre- and post-event as indicated.
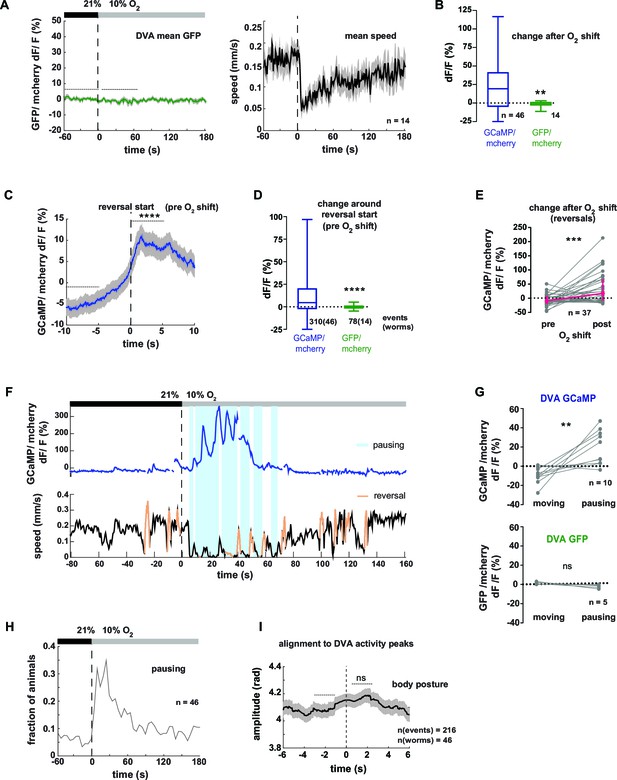
Neural activity of interneuron DVA is associated with reversals and pausing phases.
(A–I) Neural activity of DVA neurons was measured by calcium imaging and is displayed as normalized ratio of GCaMP5K/mCherry fluorescence or for control calcium-insensitive GFP/mCherry. (A) Mean DVA ratio (left) and mean locomotion speed (right) from GFP-expressing worms. Shadings indicate SEM. Time is relative to O2 downshift. n = 14 worms. (B) Boxplots showing changes of DVA ratio in response to the O2 downshift compared between GCaMP- and GFP- expressing worms. Changes were calculated by subtracting the means of 60s intervals post-downshift from the means of 60s pre-downshift intervals, indicated by dashed lines in (A). Mann-Whitney test shows significantly different changes (**p = 0.003). (C) Mean DVA activity of GCaMP-expressing worms triggered to reversal starts (t = 0) occurring during a 4 min interval at 21% oxygen. Shadings show SEM. Wilcoxon matched-pairs signed rank test compares DVA activity during 5s intervals before and after reversal start as indicated (****p<0.0001). (D) Boxplots showing DVA ratio change after reversal start of GCaMP- and control GFP- expressing animals. Mann-Whitney test shows significant difference (****p<0.0001). Numbers of events and worms are indicated. (E) DVA neural activity changes in response to O2 downshift using data only during reverse movement. Quantifications of mean activity during 60s intervals pre- and post- oxygen downshift. Values from single recordings are shown in gray and population median + interquartile range are shown in magenta. DVA activity significantly increases in GCaMP-expressing animals after the stimulus (***p=0.0002, Wilcoxon matched-pairs signed rank test). (F) Exemplary recording of neural activity (top) and locomotion speed (bottom). Time is relative to O2 downshift. Periods of reverse movement are labeled in orange. Phases when the animal paused are marked by blue shadings. (G) Quantifications of mean DVA neural activity during moving and pausing phases from worms that were pausing at least 60s of the recording time. Values from single recordings are shown in gray. Number of worms is indicated. DVA activity significantly differs in GCaMP-expressing animals (**p<0.0059) but not in GFP-expressing animals (ns p = 0.0625, Wilcoxon matched-pairs signed rank tests). (H) Fraction of GCaMP-expressing worms in pause phase. Time is relative to O2 downshift. n = 46 worms. (I) Mean amplitude of body posture triggered to DVA activity peaks (t = 0s) during forward displacement (same events as in Figure 8D). Shadings show SEM. Number of events and worms is indicated. Wilcoxon matched-pairs signed rank tests indicates no significant changes between 2 s intervals pre- and post-event as indicated (ns p = 0.086).
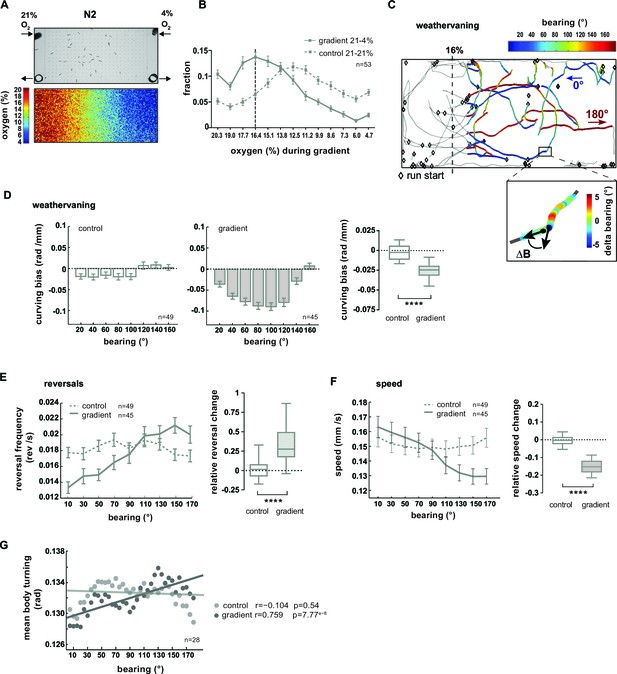
O2 chemotaxis involves regulation of turning, reversals and locomotion speed.
(A) O2 chemotaxis assay. Top: video frame capture showing worms in O2 gradient arena. Arrows indicate gas inlets and outlets. Bottom: Measured O2 gradient in the device. (B) Distributions (trial-means ± SEM) of wild type N2 worms along the length of the arena. Data binning on the x-axis is 1.3% O2. For gradient (solid line) or control (dashed line) assays either 21% and 4%, or 21% and 21% oxygen were applied at the inlets. Dashed line at 16.4% marks the bin center of the average peak accumulation during the gradient. n = 53 assays, 30-40 animals/assay. (C, D) Analysis of weathervaning chemotaxis. (C) Example trajectories of wild type N2 worms in the oxygen gradient. Color code indicates heading orientation (bearing) with respect to the O2 gradient: bearing towards or away from 16% O2 is defined as 0° or 180°, respectively. Gray trajectory sections were excluded from the analysis. Inset: example trajectory with color-code indicating the change of bearing ΔB. (D) Trial-mean curving bias ΔB per displacement (± SEM) under control (left) and gradient (middle) conditions as a function of bearing (20° binning). Right: quantification of curving bias averaged across a bearing range of 40°–150°. (E) Left: Trial-mean reversal frequency (± SEM) under control (dashed line) and gradient (solid line) conditions as a function of bearing (20° binning). Right: quantification of difference in mean reversal frequency below versus above 90° bearing. (F) Left: Trial-mean forward centroid speed (± SEM) under control (dashed line) and gradient (solid line) conditions as a function of bearing (20° binning). Right: quantification of difference in mean forward crawling speed below versus above 90° bearing. (G) Trial-mean mid-body (segment angle #11) signal amplitude of individual turning events under control (light gray) and gradient (dark gray) conditions as a function of bearing (5° binning). The linear fit and the correlation coefficients (r) are indicated; corresponding p-values indicate significance of correlation. The indicated sample sizes are n = number of experiments. All boxplots display trial-median, interquartile range and 5–95 percentile whiskers. Quantifications of control and gradient experiments are significantly different by Mann-Whitney test (****p<0.0001).
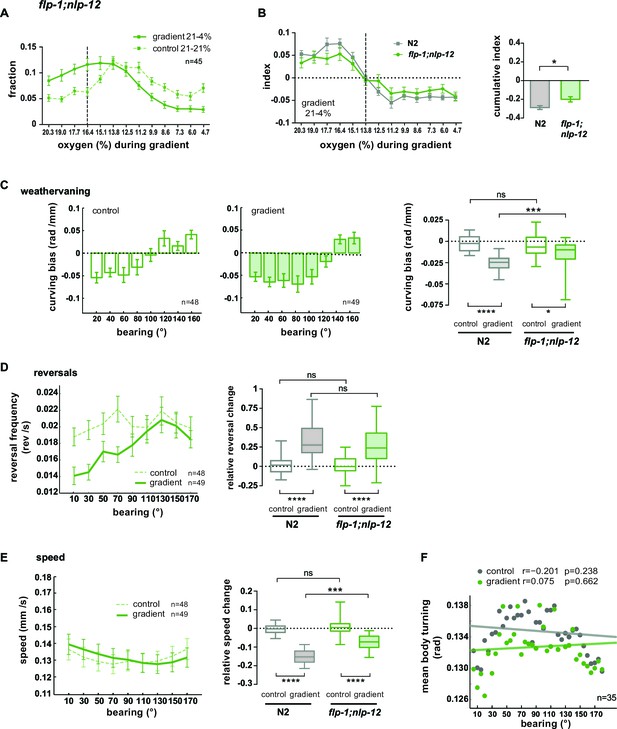
FLP1 and NLP-12 neuropeptides are implicated in the regulation of turning and locomotion speed during O2 chemotaxis.
(A) Distributions (trial-means ± SEM) of flp-1;nlp-12 mutants along the length of the arena. Data binning on the x-axis is 1.3% O2. For gradient (solid line) or control (dashed line) assays either 21% and 4%, or 21% and 21% oxygen were applied at the inlets. Dashed line at 16.4% marks the bin center of the average peak accumulation of wild type animals during the gradient. n = 45 assays, 30-40 animals/assay. (B) Left: Indices (trial-means ± SEM) of worm distributions along the length of the arena of wild type N2 (gray) and flp-1;nlp-12 mutant (green) animals, calculated by subtracting in every bin the fraction of the paired control experiment from the fraction of the respective gradient experiment. Dashed line at 13.8% O2 marks equal average accumulation during control and gradient conditions. Right: Cumulative indices (summed indices of all bins below 13.8%) of mutant and wild type N2 are compared by one-way-ANOVA with Dunnett’s correction (*p=0.035, ***p=0.0003). All strains shown in Figure 10—figure supplement 1D were included in the statistical analysis. (C) Mean curving bias (± SEM) of flp-1;nlp-12 mutants under control (left) and gradient (middle) conditions as a function of bearing (20° binning). Right: quantification of curving bias averaged across a bearing range of 40°–150°. (D) Left: Trial-mean reversal frequency (± SEM) of flp-1;nlp-12 mutants under control (dashed line) and gradient (solid line) conditions as a function of bearing (20° binning). Right: quantification of difference in mean reversal frequency below versus above 90° bearing. (E) Left: Trial-mean forward centroid speed (± SEM) of flp-1;nlp-12 mutants under control (dashed line) and gradient (solid line) conditions as a function of bearing (20° binning). Right: quantification of difference in mean forward crawling speed below versus above 90° bearing. (F) Trial-mean mid-body (segment angle #11) signal of individual turning events of flp-1;nlp-12 mutants under control (light gray) and gradient (dark gray) conditions as a function of bearing (5° binning). The linear fit and the correlation coefficients (r) are indicated; corresponding p-values indicate significance of correlation. The indicated sample sizes are n = number of experiments. All boxplots display trial-median, interquartile range and 5–95 percentile whiskers. Quantifications of control and gradient experiments per strain are compared by Mann-Whitney test. Comparisons between flp-1;nlp-12 mutant and N2 are performed with Kruskal-Wallis test with Dunn’s correction (****p≤0.0001, ***0.0001<p≤0.001, **0.001<p≤0.01, *0.01<p≤0.05, ns p>0.05). Results of wild type N2 worms (shown in gray) used for comparison to mutants rely on the same data shown in Figure 9.
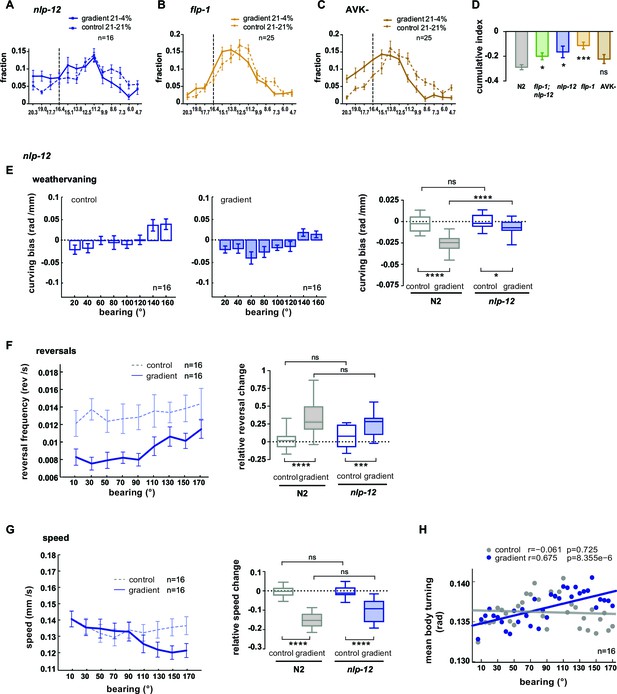
Regulation of turning during O2 chemotaxis depends on NLP-12 neuropeptides.
(A–C) Distribution (trial-means ± SEM) of manipulated worms along the length of the arena. Data binning on the x-axis is 1.3% O2. For gradient (solid line) or control (dashed line) assays either 21% and 4%, or 21% and 21% oxygen were applied at the inlets. Dashed line at 16.4% marks the bin center of the average peak accumulation of wild type animals during the gradient. Numbers of assays are indicated, 30-40animals/assay. (A) nlp-12 mutants, (B) flp-1 mutants, (C) AVK cell-ablated worms. (D) Indices were calculated by subtracting in every bin the fraction of the paired control experiment from the fraction of the respective gradient experiment. Cumulative indices (summed indices of all bins below 13.8%) of mutant strains are separately compared to wild type N2 by one-way-ANOVA with Dunnet’s correction (****p≤0.0001, ***0.0001<p≤0.001, **0.001<p≤0.01, *0.01<p≤0.05, ns p>0.05). Wild type (N2) and flp-1;nlp-12 data are from the same experiments shown in Figure 9B or 10A, B. (E) Trial-mean curving bias (± SEM) of nlp-12 mutants under control (left) and gradient (middle) conditions as a function of bearing (20° binning). Right: quantification of curving bias averaged across a bearing range of 40°–150°. (F) Left: Trial-mean reversal frequency (± SEM) of nlp-12 mutants under control (dashed line) and gradient (solid line) conditions as a function of bearing (20° binning). Right: quantification of difference in mean reversal frequency below versus above 90° bearing. (G) Left: Trial-mean forward centroid speed (± SEM) of nlp-12 mutants under control (dashed line) and gradient (solid line) conditions as a function of bearing (20° binning). Right: quantification of difference in mean forward crawling speed below versus above 90° bearing. (H) Trial-mean mid-body (segment angle #11) signal amplitude of individual turning events of nlp-12 mutants under control (light gray) and gradient (dark gray) conditions as a function of bearing (5° binning). The linear fit and the correlation coefficients (r) are indicated; corresponding p-values indicate significance of correlation. The indicated sample sizes are n = number of experiments. All boxplots display trial-median, interquartile range and 5–95 percentile whiskers. Quantifications of control and gradient experiments per strain are compared by Mann-Whitney test. Comparisons between nlp-12 mutant and N2 are performed with Kruskal-Wallis test with Dunn’s correction (****p≤0.0001, ***0.0001<p≤0.001, **0.001<p≤0.01, * 0.01<p≤0.05, ns p>0.05). Results of wild type N2 worms (shown in gray) used for comparison to mutants rely on the same data shown in Figure 9.
Videos
Wild type N2 C. elegans behavioral responses to O2 downshift.
Exemplary wild type (N2) worms from population behavioral assays at 21% ambient O2 and after a sudden shift at 10% O2. Time is relative to the O2 downshift. Note how the animals slow down their locomotory rate and up-regulate more complex and flexible postures. Time lapse 2x (20fps).
Animated undulation and turning mode shapes of a wild type N2 worm.
Reconstructed worm shapes of the decomposed undulation and turning modes and their superpositions from an exemplary wild type (N2) worm (same as in Figures 1D–G, 2C,D). Time is relative to the O2 downshift from 21% to 10%. Upper left panel shows original movie frame captures. The right panels show worm shapes reconstructed from undulation mode, turning mode and body posture time series shown in the kymographs below. The undulation mode corresponds to regular sinusoid-like worm shapes, whereas the turning mode corresponds to more strongly bent postures during shallow turns and omega turns. The diverse and complex postures observed are obtained by simple linear addition of undulation and turning shapes. Time lapse 1x (10fps).
Additional files
-
Source code 1
The zip archive contains source code written with MatLab to calculate skeletons and skeleton angles from binary worm images, perform eigenworm decomposition into undulation mode and turning mode, reconstruct worm images and movies from undulation mode and turning mode, and extract behavioral data with respect to spatial orientation (bearing direction, curving bias, reversals, speed, mean body turning).
See ‘readme.txt’ for an overview.
- https://doi.org/10.7554/eLife.14116.023