The endoplasmic reticulum, not the pH gradient, drives calcium refilling of lysosomes
Figures
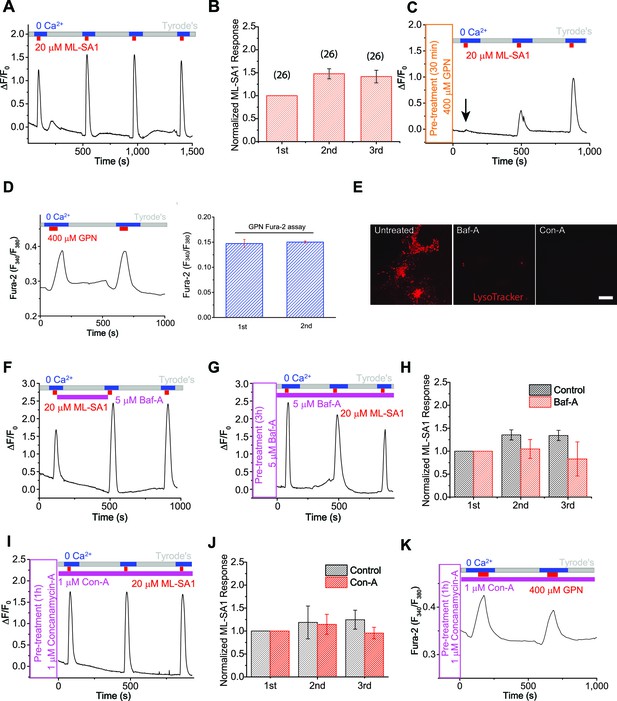
The proton gradient of the lysosome is not required for lysosomal Ca2+ store refilling.
(A) In HEK293 cells stably expressing GCaMP3-ML1 (HEK-GCaMP3-ML1 cells), bath application of the ML1 channel agonist ML-SA1 (20 µM) in a low or 'zero' Ca2+ (free [Ca2+]<10 nM) external solution induced an increase in GCaMP3 fluorescence (F470). After washout for 5 min, repeated applications of ML-SA1 induced responses that were similar to or larger than the first one. Note that because baseline may drift during the entire course of the experiment (up to 20 min), we typically set F0 based on the value that is closest to the baseline. (B) The average Ca2+ responses of three ML-SA1 applications at intervals of 5 min (n=26 coverslips; Figure 1—source data 1). (C) Pre-treatment with lysosome-disrupting agent GPN for 30 min abolished the response to ML-SA1 in HEK-GCaMP3-ML1 cells. Washout of GPN resulted in a gradual re-appearance of ML-SA1 responses. See quantitation in Figure 1—figure supplement 2K. (D) Repeated applications of GPN resulted in Ca2+ release that was measured with the Ca2+-sensitive dye Fura-2 (F340/F380) in non-transfected HEK293T cells. (E) Application of Bafilomycin-A (Baf-A, 5 µM) and Concanamycin-A (Con-A, 1 µM) quickly (<5 min) abolished LysoTracker staining, an indicator of acidic compartments. (F) Acute application of Baf-A (5 µM) for 5 min did not block Ca2+ refilling of lysosomes in HEK-GCaMP3-ML1 cells. (G) Prolonged pre-treatment (3 hr) with Baf-A did not block Ca2+ refilling of lysosomes. (H) Quantification of 1st (p value = 0.11), 2nd (p=0.01), and 3rd (p=0.004) ML-SA1 responses upon Baf-A treatment (n=8) compared to control traces (n=6; Figure 1—source data 1). (I) Prolonged treatment (1 hr) with Con-A did not prevent lysosomes from refilling their Ca2+ stores. (J) Quantification of 1st (p=0.90), 2nd (p=0.33), and 3rd (p=0.66) ML-SA1 responses with Con-A pre-treatment (n=3; Figure 1—source data 1). (K) Con-A did not reveal differences in Ca2+ refilling responses to repeated applications of GPN in untransfected HEK293T cells. Panels A, C, D, F, G, I, and K are the average of 30–40 cells from one representative coverslip/experiment. The data in panels B, H, and J represent mean ± SEM from at least three independent experiments.
-
Figure 1—source data 1
Source data of Figure 1B, H, J: The average Ca2+ responses to ML-SA1 applications under control (B), Baf-A1 treatment (H), and Con-A treatment (J).
- https://doi.org/10.7554/eLife.15887.003
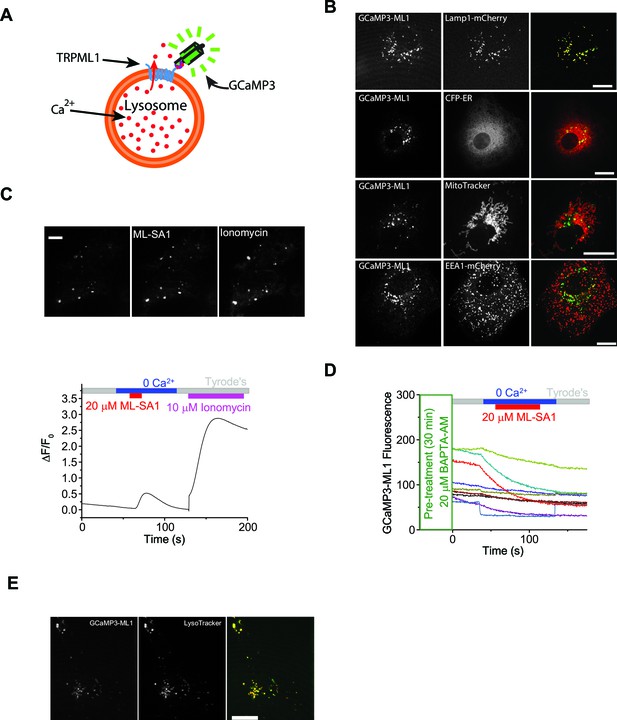
A lysosome-targeted genetically-encoded Ca2+ indicator to measure lysosomal Ca2+ release, store depletion, and refilling.
(A) Detection of lysosomal Ca2+ release by a genetically-encoded Ca2+ indicator (GCaMP3) fused directly to the N-terminus of ML1 (GCaMP3-ML1). (B) Co-localization analyses between GCaMP3-ML1 and various organellar markers, including Lamp1-mCherry, CFP-ER, Mito-tracker, and EEA1-mCherry. Scale bars = 5 μm. (C) In an HEK-GCaMP3-ML1 cell, both ML-SA1 and subsequent ionomycin induced GCaMP3 fluorescence increases in lysosomes shown by both fluorescence imaging and Ca2+ imaging. (D) BAPTA-AM pre-treatment abolished ML-SA1-induced responses in HEK-GCaMP3-ML1 cells. Panel C shows the average response of 30–40 cells from one representative experiment. (E) Cos7 cells transfected with GCaMP3-ML1 show strong co-localization with LysoTracker, which is highly selective for acidic organelles.
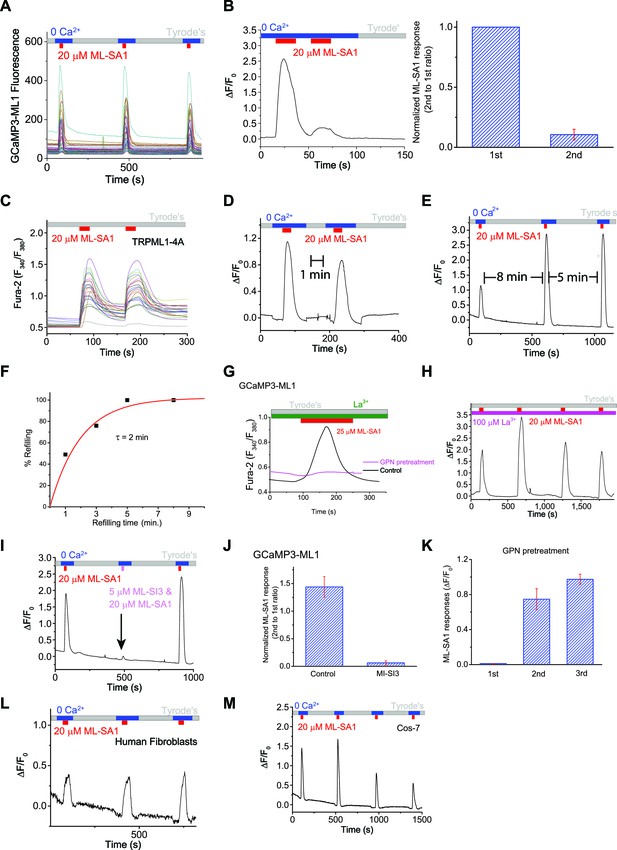
An assay to monitor lysosomal Ca2+ store depletion and refilling.
(A) Raw traces of ML-SA1-induced GCaMP3 Ca2+ responses of individual HEK-GCaMP3-ML1 cells on one coverslip. (B) Immediate re-application of ML-SA1 showed a nearly-abolished lysosomal Ca2+ release. (C) In HEK293T cells transfected with surface-expressed TRPML1-4A channels, repeated applications of ML-SA1 induced comparable responses. (D) After 1 min refilling time, application of ML-SA1 in HEK-GCaMP3-ML1 cells induced responses that were smaller than the first one. (E) The amount of Ca2+ released after 8 min of washout and refilling was similar to the amount released after 5 min. (F) Time-dependence of lysosomal Ca2+ store refilling. (G) In the presence of La3+ (100 μM), a membrane-impermeable TRPML blocker, GPN pretreatment abolished ML-SA1-induced responses in HEK-GCaMP3-ML1 cells. (H) Lysosomal Ca2+ refilling in the presence of La3+. (I) ML-SI3 (5 μM) reversibly inhibited ML-SA1-induced Ca2+ responses in HEK-GCaMP3-ML1 cells. (J) Normalized ML-SA1 responses with and without co-application of MI-SI3. (K) Quantification of ML-SA1 responses shown in Figure 1C. (L) Lysosomal Ca2+ refilling in human fibroblasts transfected with GCaMP3-ML1. (M) Lysosomal Ca2+ refilling in Cos-7 cells transfected with GCaMP3-ML1. Panel B, D, E, G, H, I, L and M show the average response of 30–40 cells from one representative experiment out of at least independent repeats.
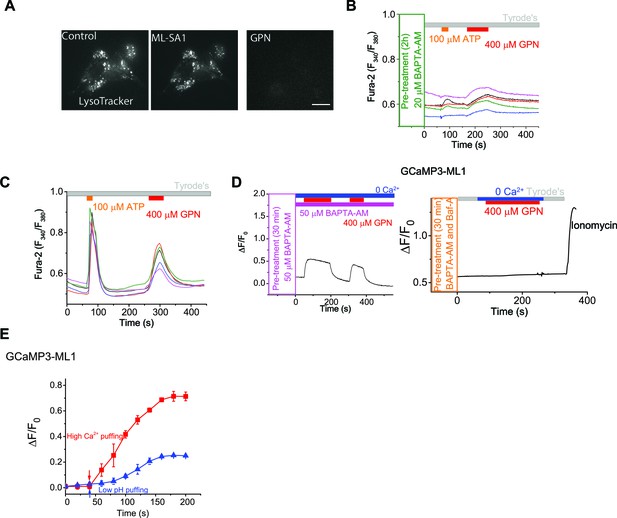
GPN and ML-SA1 have different effects on lysosome pH and GCaMP3 fluorescence.
(A) LysoTracker staining was not affected by ML-SA1 (20 μM), but was abolished by GPN (400 μM). Scale bar = 15 µm. (B) Pretreatment of a membrane-permeable form of Ca2+ chelator BAPTA (BAPTA-AM) for 2h abolished Fura-2 response to ATP in HEK293T cells. GPN still induced small increases in the Fura-2 signal in the same cells (also see panel C). (C) ATP and GPN Fura-2 responses in HEK293T cells. (D) GPN (400 μM) induced increases of GCaMP3 fluorescence in HEK-GCaMP3-ML1 cells that were pre-treated and kept in continuous presence of BAPTA-AM, and the increases were abolished by Baf-A1 co-treatment. Note that ionomycin was still able to induce GCaMP3 increases. Panels D show the average response of 30–40 cells from one representative experiment. (E) Representative traces showing GCaMP3 sensitivities to patch-electrode-based 'puffing' of low-pH and high-Ca2+ solutions to GCaMP3-ML1-expressing vacuoles isolated from HEK-GCaMP3-ML1 cells.
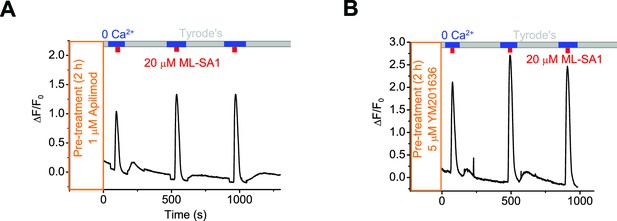
Inhibition of PI(3,5)P2 production does not prevent lysosomal Ca2+ refilling.
Representative Ca2+ imaging traces showing lysosomal Ca2+ refilling in GCaMP3-ML1 cells pretreated with Apilimod (A) or YM201636 (B). Panels A and B show the average response of 30–40 cells from one representative experiment.
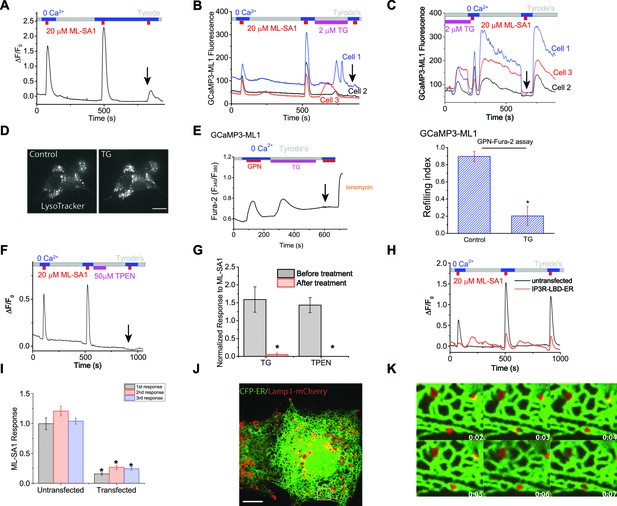
Lysosomal Ca2+ refilling is dependent on the endoplasmic reticulum (ER) Ca2+.
(A) Ca2+ refilling of lysosomes requires external Ca2+. (B) Dissipating the ER Ca2+ gradient using SERCA pump inhibitor Thapsigargin (TG) blocked lysosomal Ca2+ refilling in HEK-GCaMP3-ML1 cells. Three representative cells from among 30–40 cells on one coverslip are shown. Note that Ca2+ release from the ER through passive leak revealed after blocking SERCA pumps was readily seen in HEK-GCaMP3-ML1 cells, presumably due to the close proximity between lysosomes and the ER (Kilpatrick et al., 2013). (C) The effect of acute application of TG (2 μM) on the naïve ML-SA1 response and lysosomal Ca2+ refilling in HEK-GCaMP3-ML1 cells. Application of TG did not affect the naïve, initial response to ML-SA1, but did abolish the refilled response (see arrow). Control naïve response 1.39 ± 0.09 (n=3); Naïve response after TG 1.08 ± 0.07 (n=3); p=0.2024). (D) LysoTracker staining was not reduced by TG (2 μM). (E) Representative Ca2+ imaging trace and statistical data (right panel; Figure 2—source data 1) show that TG application reduced the second responses to GPN compared to the control shown in Figure 1D. (F) Chelating ER Ca2+ using 2-min TPEN treatment blocked Ca2+ refilling of lysosomes. (G) TG (p=0.008; n=5) and TPEN (p=0.001; n=5) abolished Ca2+ refilling of lysosomes (Figure 2—source data 1). (H) In HEK-GCaMP3-ML1 cells that were transiently transfected with the IP3R-ligand binding domain with ER targeting sequence (IP3R-LBD-ER), which significantly reduces basal [Ca2+]ER (see Figure 2—figure supplement 2E), ML-SA1 responses were reduced, compared to untransfected cells on the same coverslip. (I) The 1st (p=0.0014), 2nd (p=0.0004), and 3rd responses (p<0.0001) of GCaMP3-ML1 cells transfected with the IP3R-LBD-ER were significantly reduced compared to untransfected cells on the same coverslip (n=5; Figure 2—source data 1). (J) Lysosomes (labeled with Lamp1-mCherry) interact closely with the ER (labeled with CFP-ER). (K) Time lapse zoomed-in images of a selected region from J show the dynamics of ER-lysosome association (see an example in the boxed area). Panels A, F, H are the average responses of 30–40 cells from one representative experiment. The data in panel G represent mean ± SEM from five independent experiments.
-
Figure 2—source data 1
Comparisons of GPN (E) and ML-SA1 responses (G, I) under different pharmacological and genetic manipulations (Figure 2E,G,I).
Comparisons of responses to ML-SA1 (E) and GPN (G) (Figure 2—figure supplement 1E,G).
- https://doi.org/10.7554/eLife.15887.009
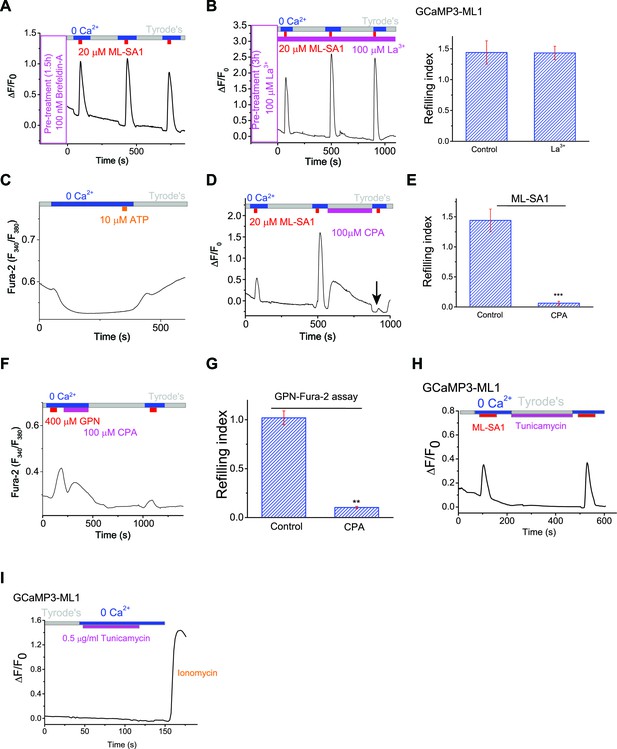
The ER Ca2+ store regulates lysosome Ca2+ stores.
(A) The effect of Brefeldin-A (100 nM) pretreatment on lysosomal Ca2+ refilling in HEK-GCaMP3-ML1 cells. (B) The effect of La3+ (100 μM) pre-treatment on lysosomal Ca2+ refilling in HEK-GCaMP3-ML1 cells. (C) The response to the endogenous P2Y receptor agonist ATP in HEK293T cells loaded with Fura-2 was abolished after perfusing cells with 0 external Ca2+ for 5 min. (D, E) SERCA pump inhibitor cyclopiazonic acid (CPA) (100 μM) blocked Ca2+ refilling of lysosomes (Figure 2—source data 1). (F, G) CPA markedly reduced the response to GPN in the Fura-2-loading cells. Note that the remaining response could be due to GPN-induced lysosomal H+ release, which resulted in a change of pre-lysosomal pH that may in turn caused a Fura-2 signal (Figure 2—source data 1). (H) Tunicamycin (0.1 μg/ml) application did not affect the second responses to ML-SA1 in GCaMP3-ML1 cells. (I) Tunicamycin (0.5 μg/ml) did not induce lysosomal Ca2+ releases. Panels A–I and K show the average response of 30–40 cells from one representative experiment.
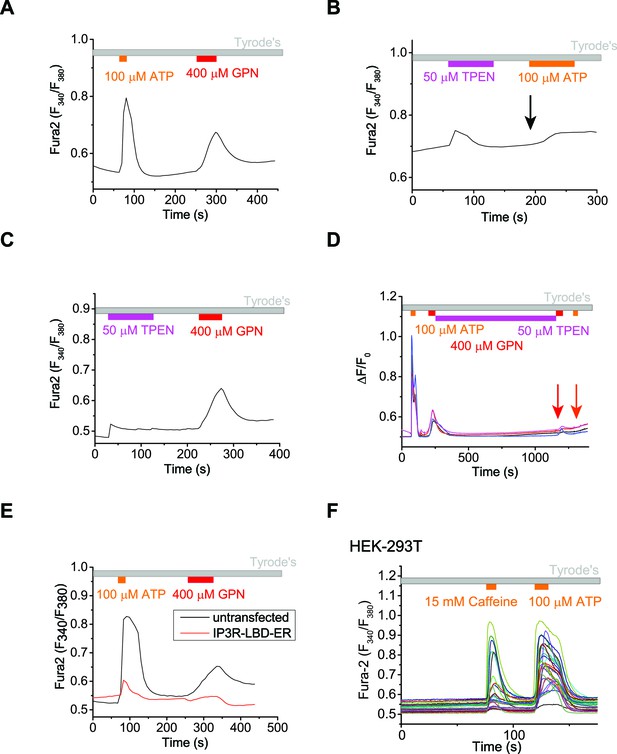
The ER Ca2+ store regulates lysosome Ca2+ stores.
(A) In un-transfected HEK293T cells, ATP induced Ca2+ release through IP3-receptors on the ER, and GPN induced lysosomal Ca2+ release. (B) A 2-min application of TPEN, a membrane-permeable chelator of luminal ER Ca2+, attenuated Ca2+ release from IP3-receptors stimulated by ATP in HEK293T cells. (C) A 2-min TPEN application did not significantly reduce GPN-induced lysosomal Ca2+ release in HEK293T cells. (D) Long-term TPEN treatment (20 min) abolished ER Ca2+ release upon ATP stimulation and GPN-induced lysosomal Ca2+ release in HEK293T cells loaded with Fura-2. (E) In HEK293T cells transfected with the IP3R-ligand binding domain with ER targeting sequence (IP3R-LBD-ER), the responses to ATP and GPN were reduced compared to un-transfected cells on the same coverslip. (F) Caffeine stimulates Ca2+ release from ryanodine receptors and ATP stimulates Ca2+ release from IP3Rs in HEK-GCaMP3-ML1 cells loaded with Fura-2. Panels A–C and E show the average response of 30–40 cells from one representative experiment.
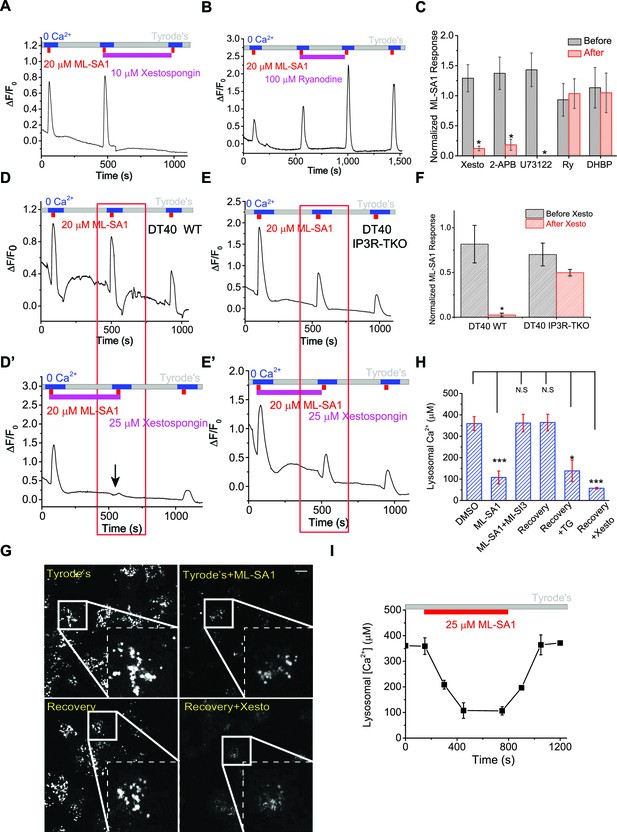
IP3-receptors on the ER are required for lysosomal Ca2+ store refilling.
(A) The IP3-receptor (IP3R) antagonist Xestospongin-C (Xesto, 10 μM) prevented Ca2+ refilling of lysosomes in HEK-GCaMP3-ML1 cells (p=0.007). Note that Xesto was co-applied with ML-SA1. (B) Ryanodine (100 μM), which blocks Ryanodine receptors at high concentrations, did not block Ca2+ refilling to lysosomes. Note that Ryanodine was co-applied with ML-SA1. (C) Quantification of the responses to ML-SA1 in HEK-GCaMP3-ML1 cells after treatment with Xesto, 2-APB (Figure 3—figure supplement 1K), U73122 (Figure 3—figure supplement 1L,M), Ryanodine (Ry), and DHBP (Figure 3—figure supplement 2A) (Figure 3—source data 1).(D) DT40 WT cells transiently transfected with GCaMP3-ML1 show Ca2+ refilling. (D’) IP3R antagonist Xesto completely blocked Ca2+ refilling of lysosomes in DT40 WT cells. (E) DT40 IP3R triple KO (TKO) cells transiently transfected with GCaMP3-ML1 also show Ca2+ refilling. (E’) Xesto did not block Ca2+ refilling of lysosomes in IP3R-TKO cells. (F) Quantification of ML-SA1 responses with or without Xesto in WT and IP3R-TKO DT40 cells (Figure 3—source data 1). (G) Representative images showing the effects of Xesto on the recovery of ML-SA1-induced responses in HEK-ML1 stable cells loaded with OG-BAPTA-dextran. La3+ was used to block external Ca2+ influx that could be mediated by surface-expressed ML1 in the overexpression system (see Figure 1—figure supplement 2G). (H) The effects of TG and Xesto on intralysosomal Ca2+ contents measured by OG-BAPTA-dextran (Figure 3—source data 1). (I) The effects of ML-SA1 on [Ca2+]Ly measured by OG-BAPTA-dextran. Panels A, B, D, D’, E, E’, F, F’ and H are the average of 30–40 cells from one representative experiment. The data in panels C, F and H represent mean ± SEM from at five independent experiments. The scale bar in panel G = 10 μm.
-
Figure 3—source data 1
Normalized ML-SA1 responses or lysosomal Ca2+ contents under pharmacological (C, H) or genetic manipulations (F) (Figure 3C, F, H).
The effects of Xesto on GPN responses in GCaMP3-ML1 (G) and MEF cells (J) (Figure 3—figure supplement 1G, J). ML-SA1 responses in GCaMP3-ML1-transfected WT and IP3R-TKO DT40 cells (Figure 3—figure supplement 2B). The effects of Xesto treatment on lysosomal Ca2+ changes induced by ML-SA1 (Figure 3—figure supplement 3E, G).
- https://doi.org/10.7554/eLife.15887.013
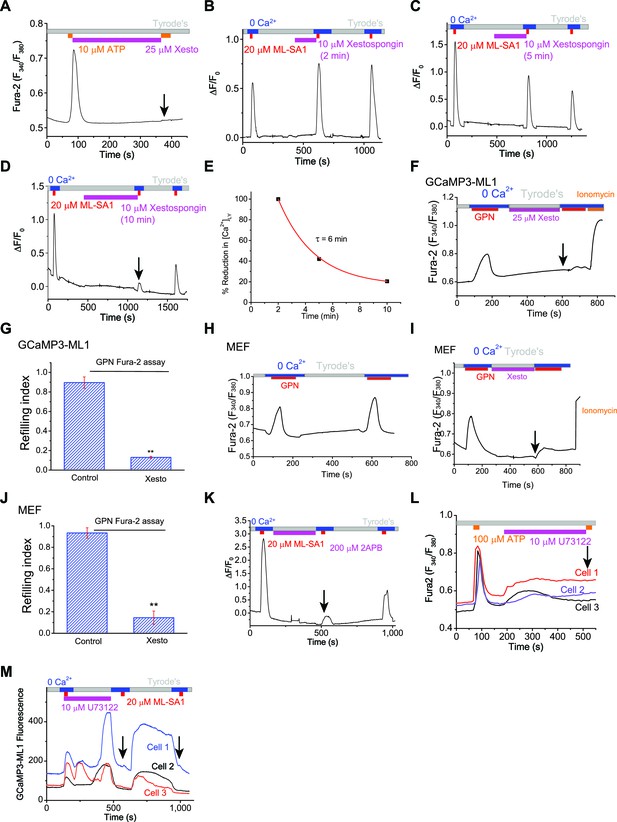
ER IP3-Receptors regulate Ca2+ refilling of lysosomes.
(A) Xesto application (5 min) blocked ER Ca2+ release in HEK293T cells loaded with Fura-2. (B) After 5 min of refilling, which is expected to fully refill the lysosomal Ca2+ stores, acute treatment of Xesto (10 μM) for 2 min did not significantly reduce lysosomal Ca2+ release. Lysosomal Ca2+ release was induced by ML-SA1 in HEK-GCaMP3-ML1 cells. (C) After 5 min of refilling of lysosomal Ca2+ stores, subsequent acute treatment of Xesto (10 μM) for 5 min slightly reduced lysosomal Ca2+ release. (D) After 5 min of refilling of lysosomal Ca2+ stores, acute treatment of Xesto (10 μM) for 10 min abolished lysosomal Ca2+ release. (E) Time-dependent depletion of lysosomal Ca2+ stores by pharmacological inhibition of IP3-receptors. (F, G) In contrast to control (Figure 1C), application of Xesto dramatically reduced Fura-2 responses to GPN in GCaMP3-ML1 cells loaded with Fura-2 (Figure 3— source data 1). (H) Fura-2 Ca2+ imaging of GPN responses in MEF cells. (I) Xesto application dramatically reduced the second response to GPN in MEF cells. (J) Average effects of Xesto on lysosomal Ca2+ refilling in MEF cells (Figure 3—source data 1). (K) IP3R antagonist 2-APB (200 μM) blocked lysosomal Ca2+ refilling (p=0.013; also see Figure 3C). (L) PLC inhibitor U73122 (10 μM) blocked Ca2+ release from IP3Rs stimulated by ATP. (M) U73122 treatment abolished Ca2+ refilling of lysosomes (p=0.0070). Panels A–D, F, H, I, K, L and M show the average response of 30–40 cells from one representative experiment.
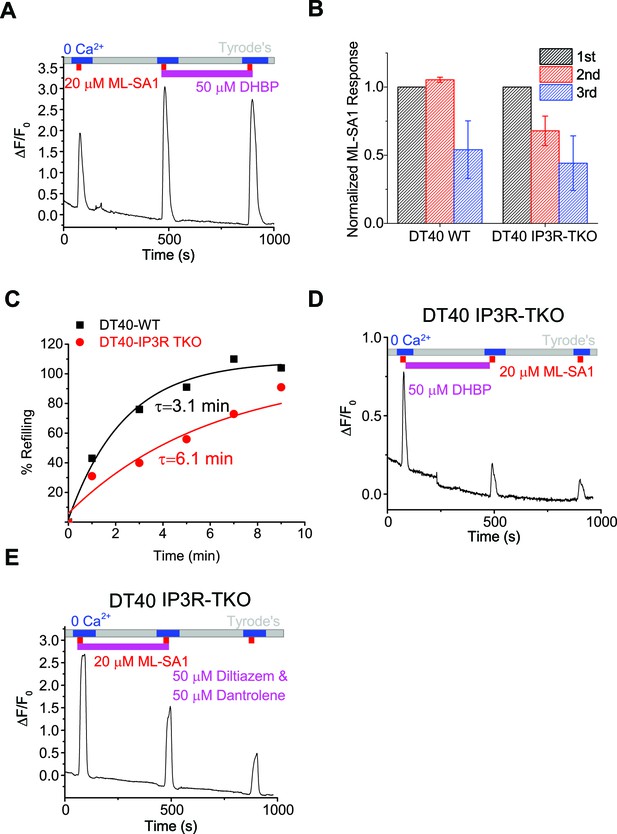
Lysosomal Ca2+ refilling is compromised in IP3R TKO DT40 cells.
(A) Ryanodine receptor blocker DHBP (50 μM) did not block Ca2+ refilling of lysosomes. (B) Quantification of the 1st, 2nd and 3rd ML-SA1 responses in GCaMP3-ML1-transfected WT and IP3R-TKO DT40 cells (Figure 3—source data 1). (C) Time-dependence of lysosomal Ca2+ store refilling in WT and IP3R TKO DT40 cells. (D) GCaMP3-ML1-transfected IP3R-TKO DT40 cells still showed refilling after 5 min of DHBP application to block RYRs. (E) RYR inhibitors Diltiazem (50 µM) and Dantrolene (50 µM) did not block lysosomal Ca2+ refilling in GCaMP3-ML1-transfected IP3R-TKO DT40 cells. Panels A, D and E show the average response of 30–40 cells from one representative experiment.
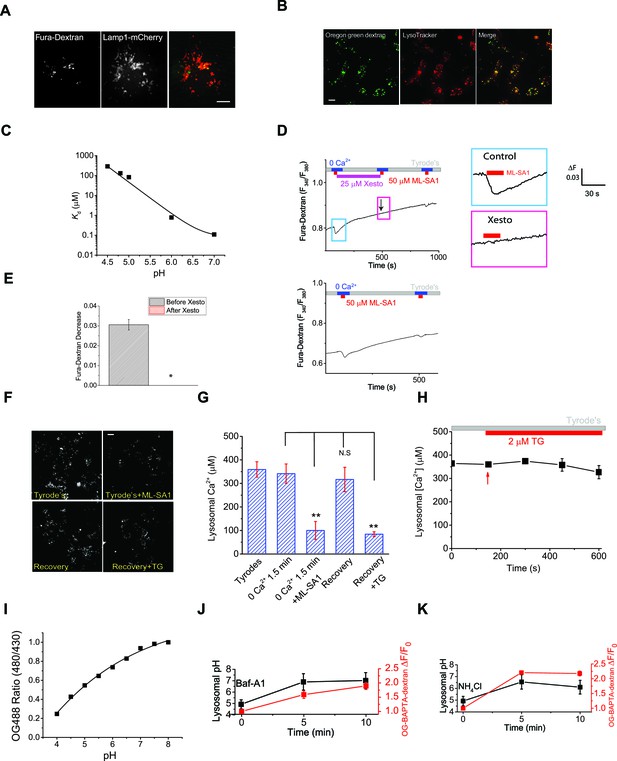
Measuring lysosomal Ca2+ release with lysosome-targeted luminal Ca2+ indicators.
(A) Fura-Dextran was pulse/chased into HEK293T cells transfected with Lamp1-mCherry. Fura-Dextran dyes were co-localized well with Lamp1-mCherry after 12 hr pulse and 4 hr chase, although not all lysosomes were loaded with the dye, evidenced by many Lamp1-mCherry vesicles without Fura-Dextran co-localization. Scale bar = 5 μm. (B) OG-BAPTA-dextran displayed better loading to lyssosomes and a high level of co-localization with LysoTracker. Scale bar =10 μm. (C) pH-dependence of the measured Kd values for OG-BAPTA-dextran. (D) Compared with the control, Xesto (25 μM) treatment for 5 min prevented Ca2+ refilling to lysosomes measured with Fura-Dextran, a lysosome-targeted luminal Ca2+ indicator. Right panels show the zoom-in images of ML-SA1-induced responses before and after Xesto treatment. (E) Quantification of ML-SA1 responses with or without Xesto in cells loaded with Fura-Dextran. Xesto significantly (p=0.026) blocked refilling as shown by no response to ML-SA1 after Xesto application during refilling (n=3; Figure 3— source data 1). (F) Representative images showing the effect of TG treatment on the recovery of ML-SA1-induced responses in OG-BAPTA-dextran loaded HEK-ML1 stable cells. (G) Average effects of TG on lysosomal Ca2+ refilling in OG-BAPTA-dextran loaded HEK-ML1 cells. Lysosomal Ca2+ release was induced by ML-SA1 in zero Ca2+ external solution (Figure 3—source data 1). (H) The effects of TG treatment on intra-lysosomal Ca2+ levels, measured by OG-BAPTA-dextran imaging. (I) A calibration curve for the pH-sensitive dye OG-488-dextran. (J, K) Baf-A1 (5 μM; J) and NH4Cl (10 mM; K) induced changes in both lysosomal pH and OG-BAPTA-dextran fluorescence.
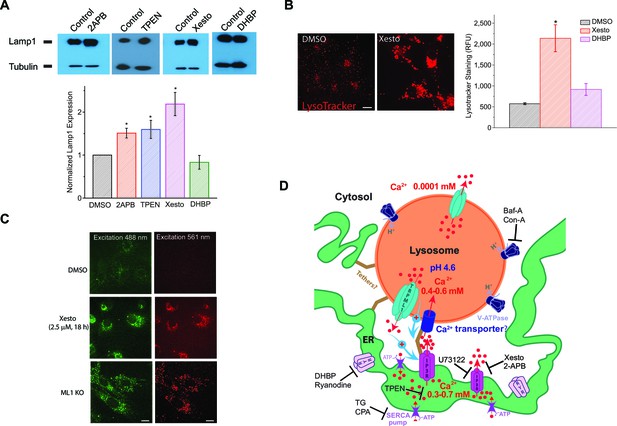
Blocking ER IP3-receptors Ca2+ channels refill lysosome Ca2+ stores to prevent lysosomal dysfunction.
(A) Upper panels: Western blotting analyses of Lamp1 in HEK293T cells treated with 2-APB (50 μM), TPEN (0.1 μM), Xesto (10 μM), and DHBP (5 μM) compared to DMSO for 24 hr (n=4 separate experiments for each condition). Lower panel: treating HEK293T cells with 2-APB (p=0.05) and Xesto (p=0.013), as well as TPEN (p=0.02), significantly increased Lamp1 expression. DHBP did not significantly change Lamp1 expression (p=0.23) (Figure 4—source data 1). (B) The effects of Xesto (10 μM, 18 hr; p=0.0001) and DHBP (50 μM, 18 hr; p=0.063) treatment compared to DMSO on the lysosomal compartments detected by LysoTracker staining in HEK293T cells (average of 20–30 cells in each of 3 experiments; Figure 4—source data 1). Scale bar = 15 μm. (C) The effect of Xesto (10 μM, 18 h) treatment on accumulation of the autofluorescent lipofuscin materials in non-transfected HEK293T cells. Autofluorescence was observed in a wide spectrum but shown at two excitation wavelengths (488 and 561 nm). ML1 KO MEFs are shown for comparison. Scale bar = 15 μm. (D) A proposed model of Ca2+ transfer from the ER to lysosomes. The ER is a Ca2+ store with [Ca2+]ER ~ 0.3–0.7 mM; lysosomes are acidic (pHLy ~ 4.6) Ca2+ stores ([Ca2+]Ly ~ 0.5 mM). IP3Rs on the ER release Ca2+ to produce a local high Ca2+ concentration, from which an unknown low-affinity Ca2+ transport mechanism refills Ca2+ to a lysosome. Unidentified tether proteins may link the ER membrane proteins directly with the lysosomal membrane proteins to maintain contact sites of 20–30 nm for purposes of Ca2+ exchange. Ca2+ released from lysosomes or a reduction/depletion in [Ca2+]Ly may, through unidentified mechanisms, 'promote' or 'stabilize' ER-lysosome interaction (Phillips and Voeltz, 2016; Eden, 2016). At the functional ER-lysosome contact sites, Ca2+ can be transferred from the ER to lysosomes through a passive Ca2+ transporter or channel based on the large chemical gradient of Ca2+ that is created when lysosome stores are depleted. Baf-A and Con-A are specific V-ATPase inhibitors; Xesto and 2APB are IP3R blockers; U73122 is a PLC inhibitor that blocks the constitutive production of IP3; DHBP and Ryanodine (>10 μM) are specific RyR blockers; TG and CPA are SERCA pump inhibitors; and TPEN is a luminal Ca2+ chelator.
-
Figure 4—source data 1
Source data of Figure 4A,B: Quantifications of Lamp-1 protein levels (A) or LysoTracker staining (B) under different experimental conditions and manipulations.
- https://doi.org/10.7554/eLife.15887.018