Mia40 is a trans-site receptor that drives protein import into the mitochondrial intermembrane space by hydrophobic substrate binding
Figures
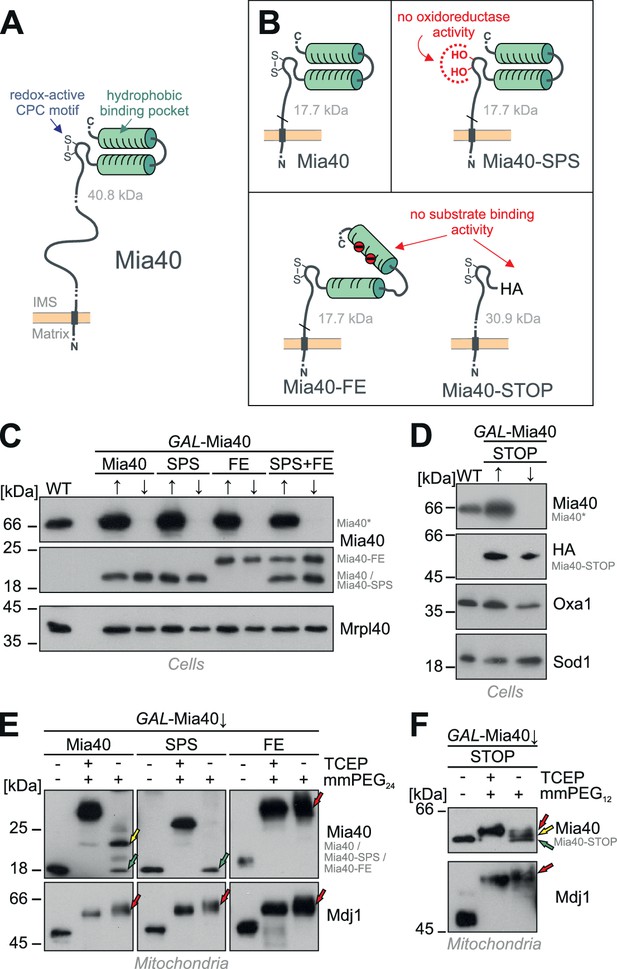
Generation of Mia40 mutants lacking either the oxidoreductase or substrate-binding activity.
(A) Schematic representation of the Mia40 structure. (B) Structure of the Mia40 variants used for complementation studies. Molecular masses of the matured (MPP-cleaved) variants are indicated. (C, D) The indicated variants were expressed in GAL-Mia40 strains. Cells were grown in synthetic lactic acid-based medium containing 0.5% galactose (↑) or glucose (↓) to induce or repress the GAL promoter, respectively. The expression levels of the indicated Mia40 variants were assessed by Western blotting. Due to the increased net charge, Mia40-FE shows a reduced migration on SDS-PAGE. Mia40* depicts the endogenous full-length Mia40. (E) To monitor the redox state of the Mia40 variants, proteins of the indicated strains were TCA-precipitated, denatured in SDS, treated with the reducing agent TCEP and the alkylating compound mmPEG24 and visualized by SDS-PAGE and Western blotting. Mdj1 is a matrix chaperone with 10 reduced cysteine residues which was used for control. Green arrows depict fully oxidized Mia40 species, yellow arrows the wild type Mia40 with the CPC reduced and the twin Cx9C structure oxidized, and the red arrow a Mia40 protein in which the twin Cx9C structure does not contain disulfide bonds. (F) The redox state of the Mia40-STOP variant was analyzed as described for E with the exception that mmPEG12 was used for alkylation. The arrowheads depict different redox states of the protein suggesting that Mia40-STOP is partially oxidized.
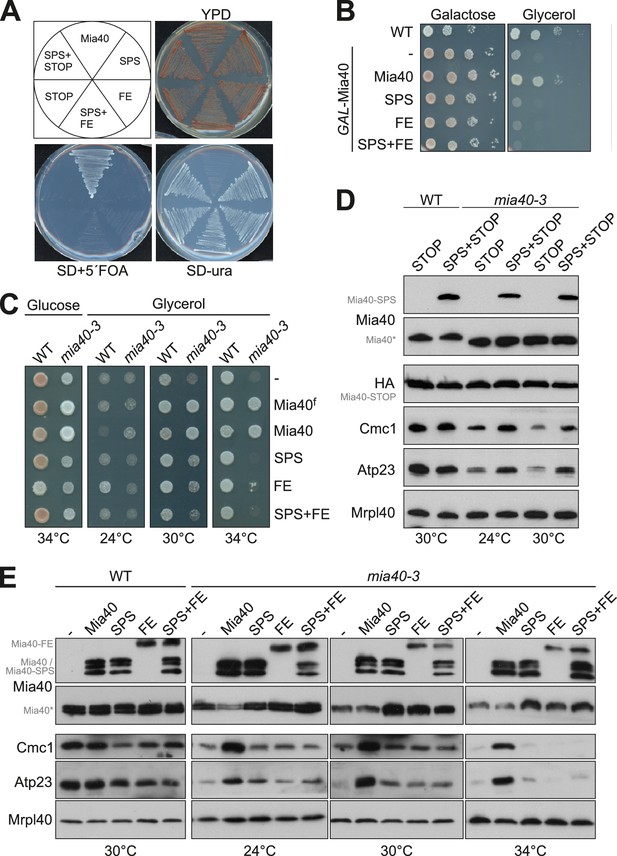
Mia40 mutants lacking either the oxidoreductase or the substrate-binding activity cannot cross-complement each other.
(A) Δmia40 cells containing MIA40 on a URA3 plasmid were transformed with plasmids for expression of the indicated Mia40 variants. After growth on uracil-containing medium, loss of the Mia40-containing URA3 plasmid was tested by growth on 5-fluoroorotic acid (5´FOA, which is converted to the toxic 5-fluorouracil in the presence of Ura3). Loss of the URA3 plasmid was lethal except upon expression of wild type Mia40, indicating that none of the mutated Mia40 variants rescued the Δmia40 mutants, even if expressed in combinations. (B) Expression of wild type Mia40 but not of the Mia40 variants rescued the inability of GAL-Mia40 mutants to grow on glycerol medium. (C) Wild type cells and the temperature-sensitive mia40-3 mutant were transformed with plasmids expressing full-length Mia40 (Mia40f) (cf. Figure 1A) or the indicated Mia40 variants (cf. Figure 1B). The mutated Mia40 variants did not allow growth at restrictive temperature. (D, E) Protein levels were analyzed by Western blotting showing that the different Mia40 variants were well expressed in the mia40-3 mutant. Please note that only the expression of wild type Mia40 resulted in the efficient accumulation of Mia40 substrates such as Cmc1 and Atp23. However, small amounts of Cmc1 and Atp23 were also observed in the Mia40-SPS but not in Mia40-FE or the Mia40-STOP cells.
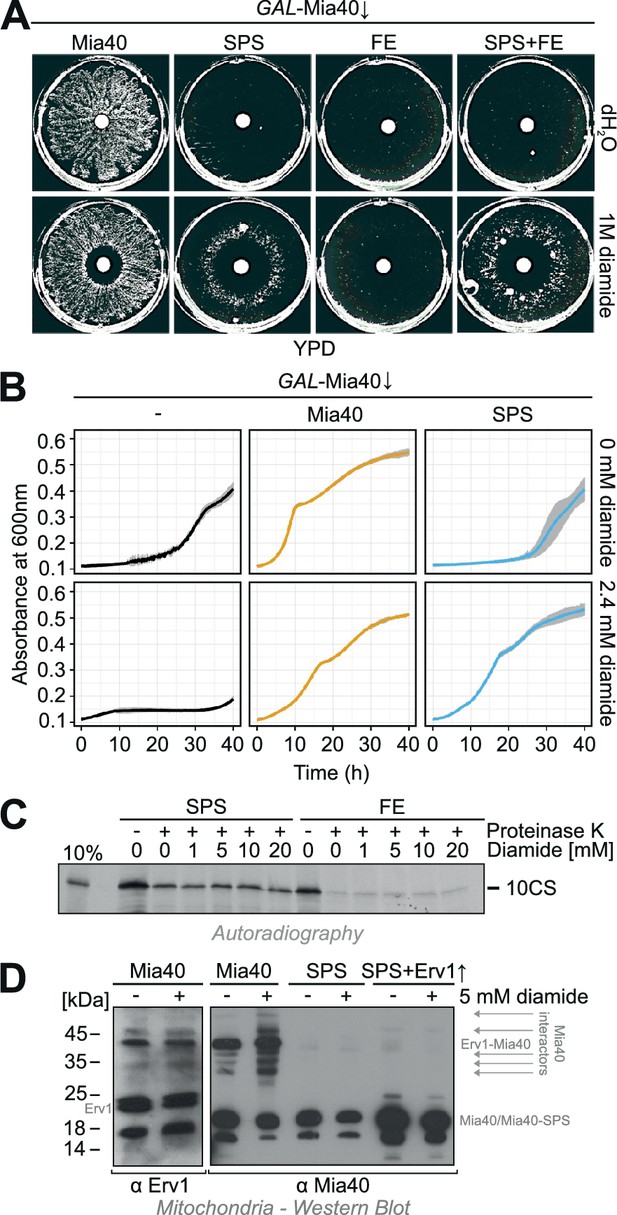
Diamide partially rescues the growth defect of the Mia40-SPS mutant.
(A) The indicated strains were grown in synthetic lactic acid-based medium containing 0.5% glucose for three days to deplete endogenous Mia40 and spread on glucose plates. 10 µl water or 1 M diamide was applied onto a filter dish in the middle of the plate. Note the ring-like growth of the Mia40-SPS mutant around diamide-containing filter. (B) GAL-Mia40 mutants lacking or containing plasmids for expression of the Mia40 or the Mia40-SPS variant were grown for 72 hr in synthetic lactic acid-based medium supplemented with 0.5% glucose and diluted to OD 0.1 in the same medium lacking or containing 2.4 mM diamide. Growth at 30°C under constant shaking was then analyzed continuously in a multiwell absorption reader (BioTek ELx808, BMG Labtech). Error bars represent SD with n = 4. (C) Mia40-SPS- and Mia40-FE-containing mitochondria were incubated in import buffer for 5 min in the presence of the indicated concentrations of diamide. Then the radiolabeled cysteine-less Atp23 variant 10CS was added. After incubation for 15 min at 25°C, non-imported protein was removed by protease treatment. Mitochondria were washed and subjected to SDS-PAGE and autoradiography. (D) Mitochondria were isolated from glucose-grown GAL-Mia40 cells expressing the indicated Mia40 variants. They were treated with or without 5 mM diamide for 10 min at 30°C, reisolated, resuspended in non-reducing sample buffer and analyzed by Western blotting. Many disulfide-linked adducts are observed with wild type Mia40, particularly after incubation with diamide, but not with Mia40-SPS, not even when Erv1 is overexpressed.
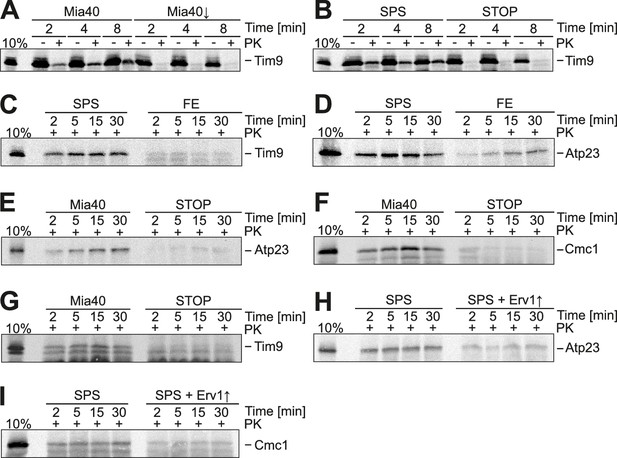
Substrate-binding by Mia40 is necessary and sufficient for protein translocation into the IMS.
(A) Depletion of Mia40 blocks import of Tim9. Radiolabeled Tim9 was incubated at 25°C for the times indicated with mitochondria isolated from Mia40-depleted cells lacking or containing a plasmid for expression of the Mia40 variant. After treatment with proteinase K (PK) mitochondria were reisolated, washed and subjected to SDS-PAGE and autoradiography. 10% of the radiolabeled protein used per import sample is shown for comparison. (B–I) In vitro import reactions with radiolabeled Tim9, Atp23 and Cmc1 into the mitochondria of the indicated strains.
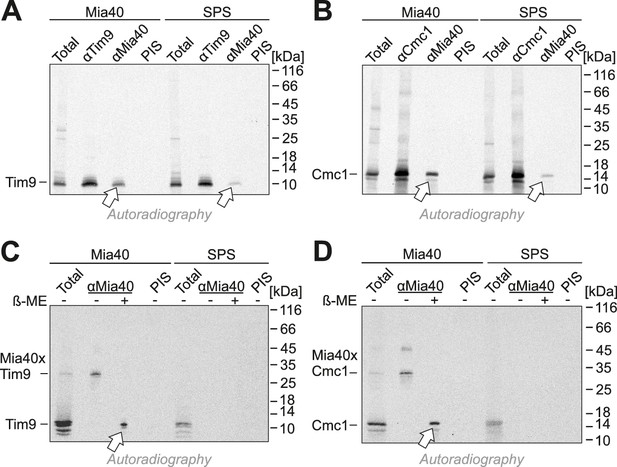
Mia40-SPS binds to import intermediates of Tim9 and Cmc1.
(A, B) Radiolabeled Tim9 and Cmc1 were incubated for 2 min with isolated mitochondria containing Mia40 or Mia40-SPS. Mitochondria were reisolated and lysed with 1% SDS. The extract was used for immunoprecipitation with Tim9-, Cmc1- or Mia40-specific antibodies or with preimmune serum (PIS). Disulfide bonds were reduced with DTT. Radioactive proteins were visualized by SDS-PAGE and autoradiography. Total samples contain 10% of the material used per immunoprecipitation reaction. Arrows depict radiolabeled proteins pulled down with Mia40 and Mia40-SPS. (C, D) Mia40- or Mia40-SPS-containing mitochondria were treated for 10 min with 5 mM DTT at 30°C, tenfold diluted and incubated with radiolabeled Tim9 or Cmc1 for 2 min. Reisolated mitochondria were lysed with 1% SDS before the extract was used for immunoprecipitation using Mia40-specific antibodies or preimmune serum for control. Reducing (+ ß-mercaptoethanol, ß-ME) or non-reducing samples were analyzed by SDS-PAGE and autoradiography. See figure supplements for additional panels.
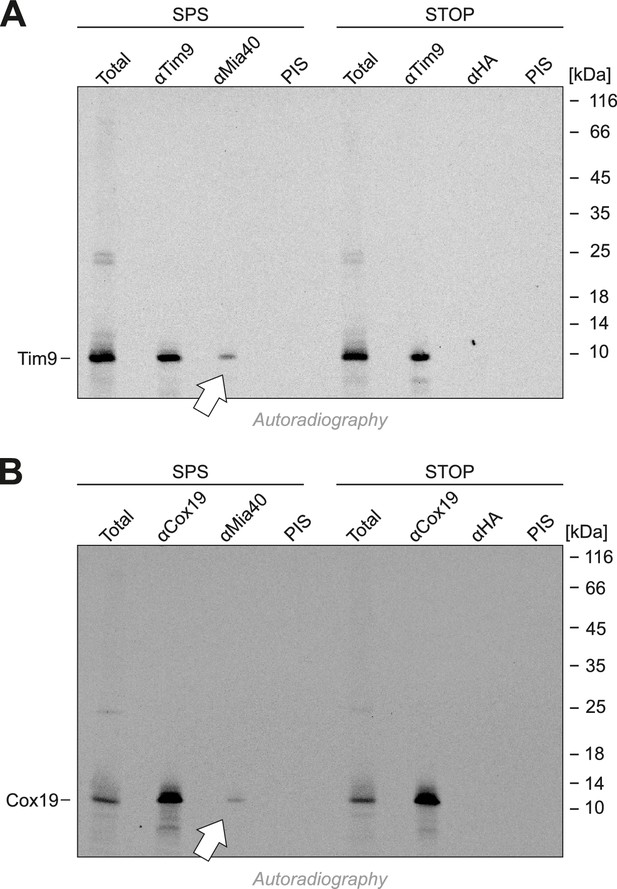
The substrate-binding cleft but not the redox-active CPC motif of Mia40 is sufficient for the import of Tim9 and Cox19.
(A, B) Radiolabeled Tim9 and Cox19 were imported for 2 min into isolated mitochondria containing Mia40-SPS or Mia40-STOP. Mitochondria were reisolated and lysed under native conditions with lysis buffer N (30 mM Tris/HCl pH 8, 100 mM NaCl, 0.1% Triton X-100, 2 mM PMSF), followed by a clarifying spin. The preimmune serum or antibodies against Tim9, Cox19, Mia40 or hemagglutinin were coupled to protein A-sepharose beads. The beads were incubated with the mitochondrial extract for 1 hr at 4°C, washed twice with lysis buffer N and once with 20 mM Tris pH 8, resuspended in reducing SDS-sample buffer and boiled for 5 min at 96°C. Samples were analyzed by SDS-PAGE and autoradiography. Total samples contain 10% of the material used per immunoprecipitation reaction.
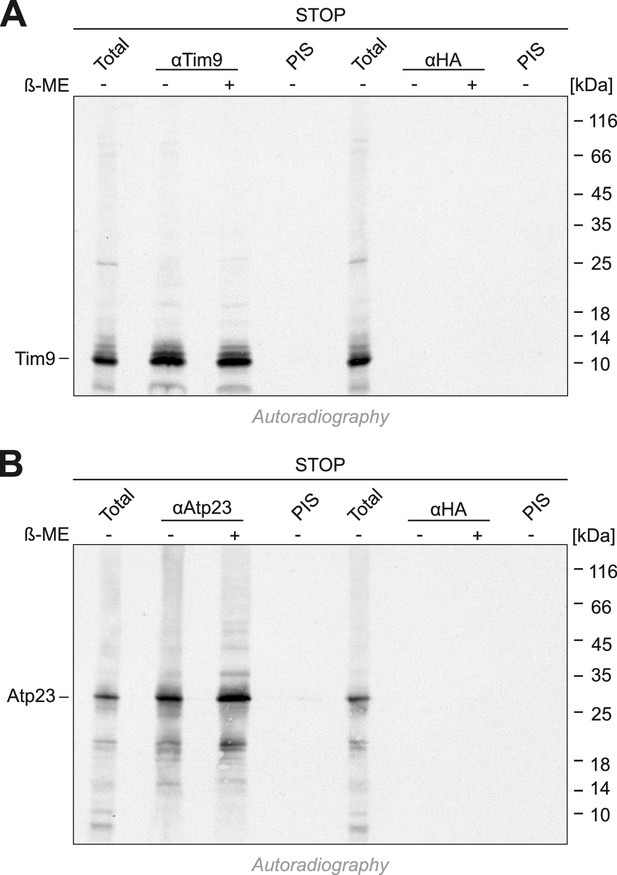
Cysteines in the CPC motif of Mia40 are dispensable for the import of Tim9 and Atp23.
(A, B) Radiolabeled Tim9 and Atp23 were imported for 2 min into isolated mitochondria containing Mia40-STOP. Mitochondria were reisolated and lysed with 1% SDS. The extract was used for immunoprecipitation with Tim9-, Atp23- or hemagglutinin-specific antibodies or with preimmune serum. Samples were loaded reducing or non-reducing as indicated, and analyzed by SDS-PAGE and autoradiography. Total samples contain 10% of the material used per immunoprecipitation reaction.
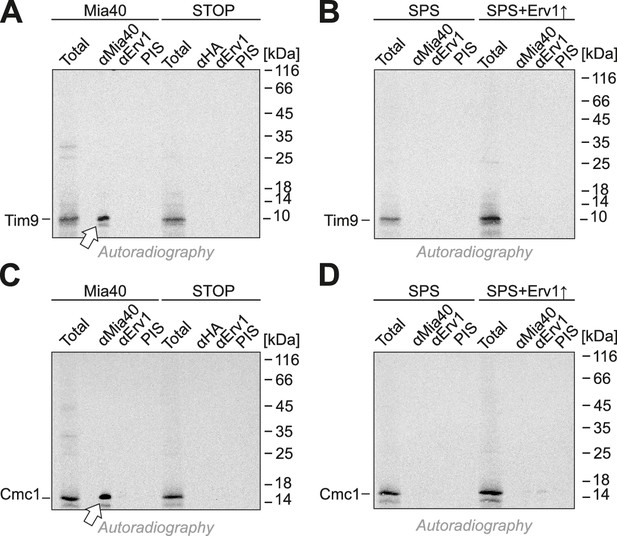
Tim9 and Cmc1 form mixed disulfides with Mia40 but not with Mia40-SPS or Mia40-STOP.
(A, B) Radiolabeled Tim9 or (C, D) Cmc1 were imported for 2 min into isolated mitochondria containing Mia40, Mia40-STOP, Mia40-SPS or Mia40-SPS+Erv1↑. Mitochondria were reisolated and lysed with 1% SDS, diluted in Triton X100 buffer and subjected to a clarifying spin. The preimmune serum or antibodies against Mia40, Erv1 or hemagglutinin were coupled to protein A-sepharose beads. The beads were incubated with the mitochondrial extracts over night at 4°C, washed, resuspended in reducing SDS-sample buffer and boiled for 5 min at 96°C. Samples were analyzed by SDS-PAGE and autoradiography. Total samples contain 10% of the material used per immunoprecipitation reaction.
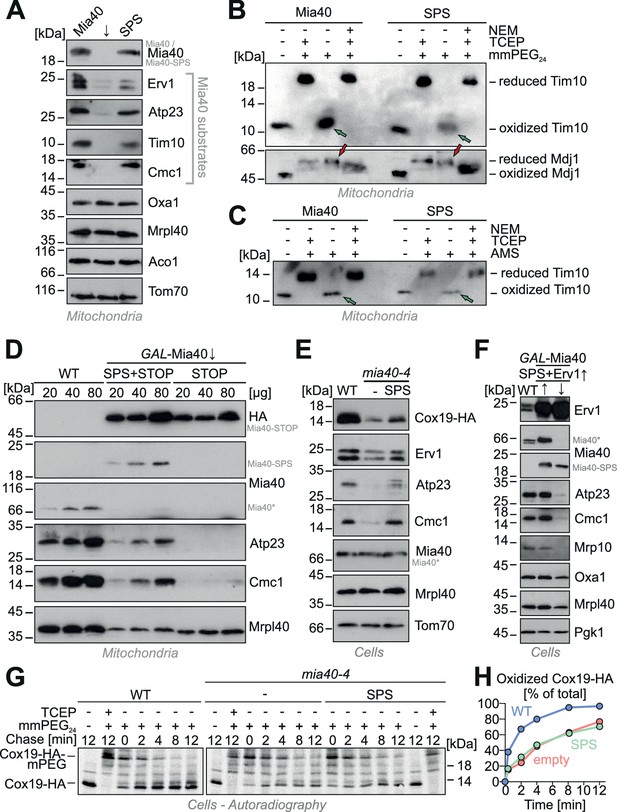
Expression of Mia40-SPS leads to the accumulation of Mia40 substrates in mitochondria.
(A) Mitochondria were isolated from Mia40-depleted cells (↓) which expressed Mia40 or Mia40-SPS. The steady state levels of Mia40 substrates and control proteins were analyzed by Western blotting. (B, C) To determine the redox state of Tim10, proteins of Mia40- and Mia40-SPS-containing mitochondria were TCA-precipitated, denatured in SDS, treated with or without the reducing agent TCEP and the alkylating compounds mmPEG24 (B) or AMS (C) and visualized by SDS-PAGE and Western blotting. An inverse shift was achieved by blocking reduced thiols with N-ethylmaleimide (NEM) prior to TCA precipitation. Green arrows depict fully oxidized Tim10, red arrows the matrix chaperone Mdj1 which does not contain disulfide bonds. (D) Western blots of mitochondrial extracts of the indicated strains. Please note that expression of Mia40-SPS but not of Mia40-STOP leads to accumulation of low levels of Atp23 and Cmc1 in mitochondria. (E) Wild type and mia40-4 cells were transformed with a Cox19-HA-expressing plasmid (Bode et al., 2015) allowing detection of Cox19 with hemagglutinin (HA) antibodies in whole cell extracts. Levels of Mia40 substrates and other mitochondrial proteins were analyzed by Western blotting in the indicated cells after growth at 34°C. (F) Western blot analysis of protein levels in wild type and GAL-Mia40 cells expressing Mia40-SPS variant and high levels of Erv1. Arrows up and down indicate the expression or depletion of Mia40 in this strain, respectively. (G, H) To follow the oxidation of Cox19 in vivo, cells of the indicated strains were pulse-labeled for 3 min with [35S]-methionine and chased with cold methionine for different times (Kojer et al., 2015). TCA-precipitated protein extracts were treated with mmPEG24, immunoprecipitated with hemagglutinin-antibodies and analyzed by SDS-PAGE and autoradiography.
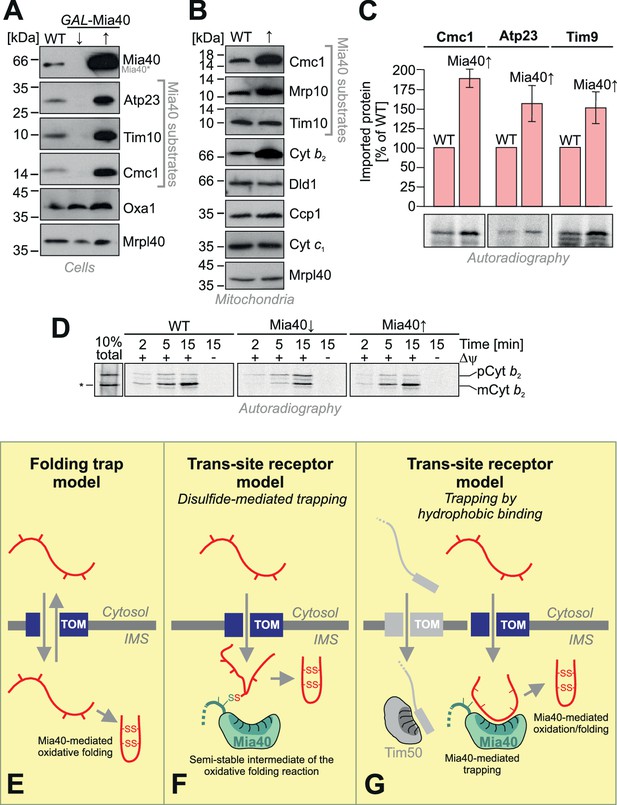
Mia40 is rate-limiting for the import of IMS proteins.
(A) Western blot analysis of mitochondrial protein levels in galactose-grown wild type (WT) cells and in GAL-Mia40 cells grown in lactic acid-based media containing 0.5% glucose (↓) or galactose (↑). Overexpression of Mia40 leads to strongly increased steady-state levels of its substrates such as Atp23, Tim10 and Cmc1. (B) Mitochondria were isolated from WT and GAL-Mia40 cells cultured as described in A. Steady state levels of IMS proteins were analyzed by Western blotting. Please note the increased level of cytochrome b2 upon overexpression of Mia40. (C) Quantifications of the amounts of imported radiolabeled proteins from in vitro import experiments with the Mia40 substrates Cmc1, Atp23 and Tim9. Error bars correspond to SEM with n = 4. Radioactive signals of one representative experiment are shown. (D) In vitro import reaction with radiolabeled cytochrome b2 into mitochondria of the indicated strains. A shorter (‘pseudomature’) form of cytochrome b2 that is formed by initiation at the second ATG codon is indicated by an asterisk. (E–G) Schematic representations of the ‘folding trap model’ and the ‘trans-site receptor’ models. According to the folding trap model, protein translocation into the IMS is driven by the formation of disulfides which prevents back-translocation into the cytosol. The ‘trans-site receptor model' suggests that translocation is driven by the affinity of incoming proteins to the substrate-binding pocket of Mia40. It was suggested that the disulfide-mediated trapping is essential for the translocation reaction. However, our results shown here suggest that the trapping via hydrophobic binding of the incoming polypeptide to Mia40 is essential and sufficient for the translocation reaction. Oxidative folding is only a subsequent reaction which can facilitate the release from Mia40 and increase the proteolytic stability of imported proteins. See discussion for details.