NG2 glial cells integrate synaptic input in global and dendritic calcium signals
Figures
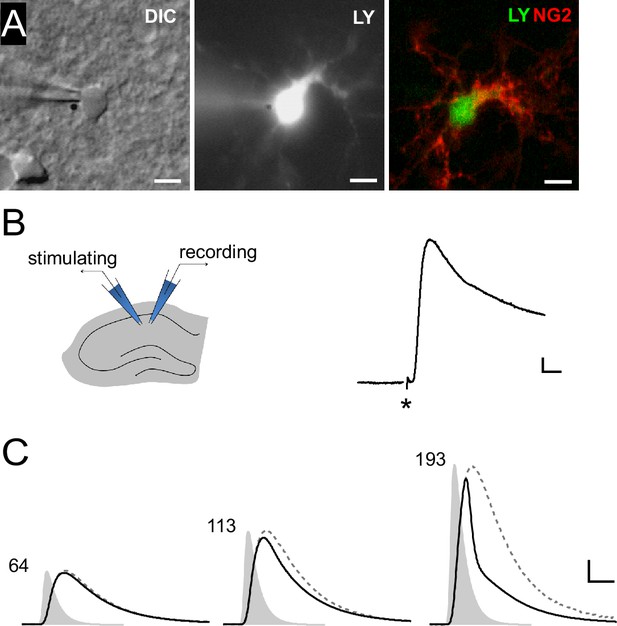
Synaptic potentials in NG2 cells can recruit voltage-gated ion channels.
(A) Identification of NG2 cells. Wide-field images of a lucifer yellow-filled cell expressing DsRed (not shown) in the acute slice preparation (left: DIC, middle: epifluorescence). Post-recording anti-NG2 staining confirmed the cell’s identity (right). Scaling: 5 µm. (B) Left: Experimental configuration used for Schaffer-collateral stimulation and whole-cell recording of postsynaptic potentials (PSPs) in NG2 cells. Right: Example PSP recording with the asterisk showing the time of the stimulation. Scaling: 5 mV, 5 ms. (C) Large mock PSPs (black lines) are shortened when compared to small mock PSPs (dashed lines, scaled for comparison). Injected currents derived from miniature excitatory postsynaptic currents (EPSCs) are shown as grey shaded areas and the numbers indicate their amplitudes in terms of glutamate quanta. The kinetic differences between the waveform of the injected current and the small mock PSP in the left panel (64 quanta) is due to filtering by the membrane capacitance. Scaling: 10 mV, 3 ms.
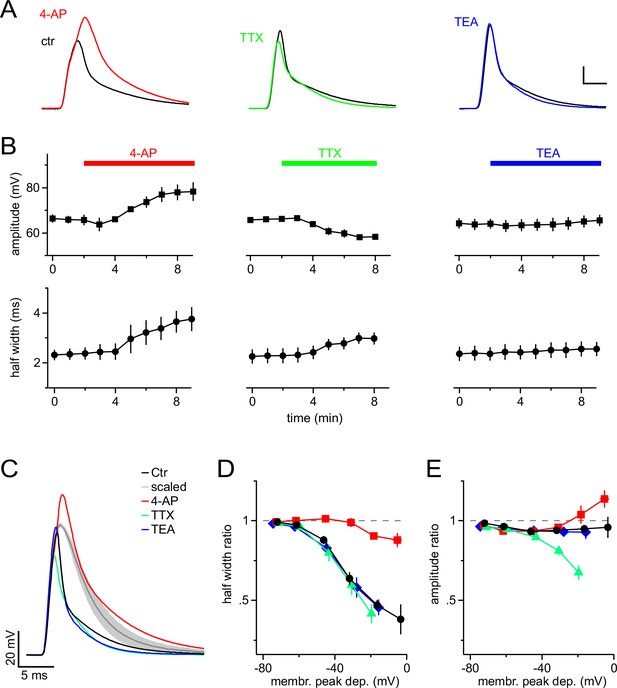
A-type K+ channels shorten mock PSPs in NG2 cells.
(A) Blocking A-type K+ channels with 4 mM 4-AP strongly broadens mock PSPs and increases their amplitude, while blocking Na+ channels with 1 µM TTX slightly reduces their amplitude. Application of 10 mM TEA to inhibit delayed-rectifier K+ channels shows no effect. Scalings: 15 mV, 3 ms. (B) Time-course of the average amplitude and half width of the mock PSPs during application of ion channel blockers. (C) Superimposed mock PSPs recorded after pre-treating cells with channel blockers. Cells were kept at −85 mV and mock current injection amplitude was chosen based on small passive PSP responses in each cell, such that they would be depolarized to −15 mV if there were no voltage-gated channels present. The grey line represents the average of the scaled passive responses for all examples shown (shaded area indicates standard error of the mean (SEM), n=4 cells). Note the difference between the grey and the other lines, illustrating the reproducibility of the effect of ion channels on the mock PSPs across different NG2 cells. (D) A-type channels shorten mock PSPs when NG2 cells are depolarized above −60 mV. The width of mock PSPs is normalized on the width of a passive mock PSP recorded in the same cell and plotted against the membrane potential reached during the peak of the PSPs. Gradually increased current injections were used to achieve larger depolarizations. Note that 4-AP almost completely removes shortening of PSPs. (E) A-type K+ channels and fast Na+ channels affect the amplitude of mock PSPs in opposite ways and cancel each other’s effect. Plot as in (D), except that the normalized amplitude is plotted on the left axis. Note that the control curve (black line) stays close to unity showing the fine balance between TTX- and 4-AP-sensitive channels. For D & E, n=14, 5, 7 and 6 cells for control, 4-AP, TTX and TEA group, respectively.
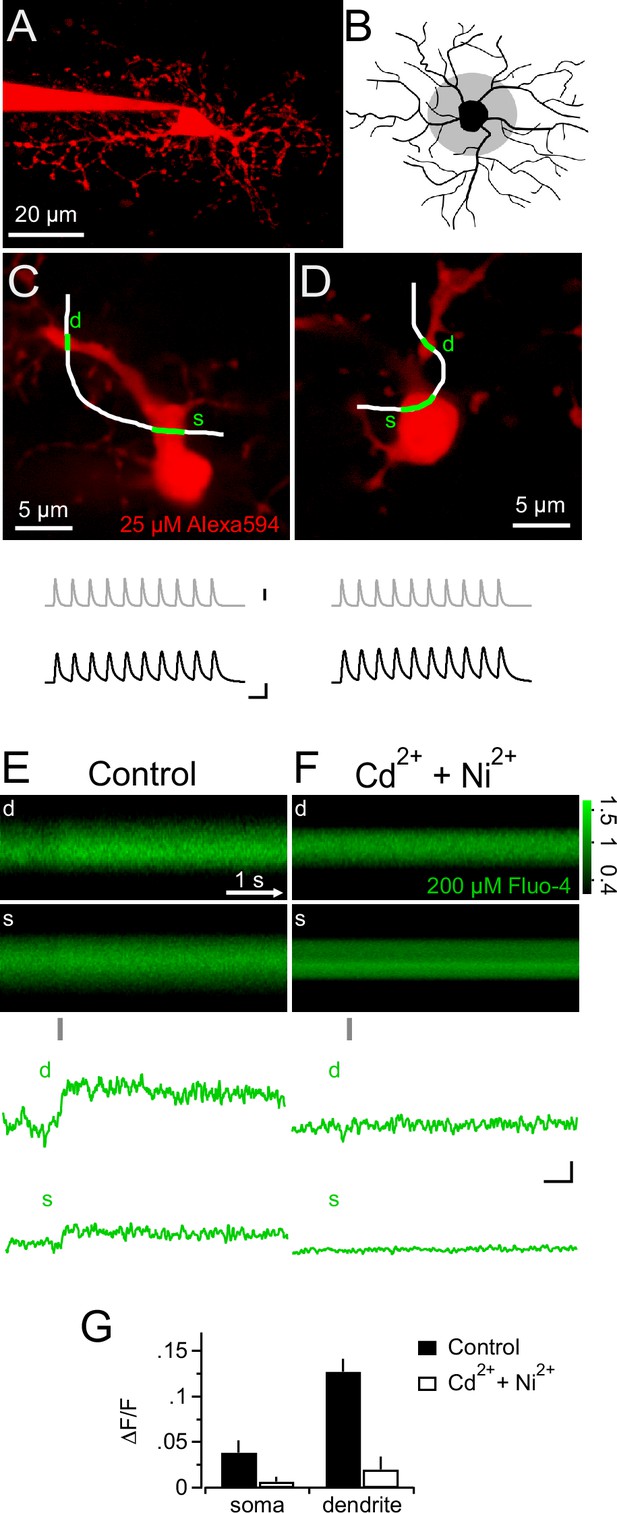
Synaptic depolarizations trigger Ca2+ signals in NG2 cells by recruiting VGCCs.
(A) Maximum intensity projection from a 2-photon image stack of an Alexa 594-filled NG2 cell during recording in a hippocampal slice. (B) Grey shaded area indicates region used for selecting line-scans. Lines were scanned within the inner one third of the dendritic tree. (C) Frame-scan (Alexa 594) illustrates configuration of freehand line-scan (white line) through soma(s) and dendrite (d) for a control group cell (n=7). Grey and black traces show the injected current waveform and the voltage response, respectively. The average amplitude of the first PSP was 78.0 ± 2.9 mV. Scaling: grey trace, 1000 pA; black traces, 40 mV, 10 ms (also apply to D). (D) As in (C) but for an NG2 cell bathed 10–15 min in 300 µM Cd2+ and 200 µM Ni2+ (n=5). The average amplitude of the first PSP was 89.5 ± 1.6 mV. (E) Rectangular images show the temporal sequence of the somatic and dendritic parts of the freehand line-scan of the cell shown in (C). Grey bar below the images indicates the time and duration of current injection. Both images show a clear fluorescence increase following current injection. Traces at the bottom (line-scan profiles) show spatial averages of line-scans of the Fluo-4 channel over time (d, dendrite, s, soma). Note the clear fluorescence increase after the current injection and that the current injection is very brief compared to the calcium signal and happens only during its rising phase. Line-scans and line-scan profiles are the average of three repetitions. (F) As is (E) but for an NG2 cell bathed 10–15 min in 300 µM Cd2+ and 200 µM Ni2+ (n=5). Note the absence of an indicator response after current injection in the presence of these VGCCs antagonists. Scalings also apply to E: 0.1 ∆F/F, 0.5s. (G) Ni2+ and Cd2+ potently block the peak amplitudes of both the somatic and the dendritic calcium transient.
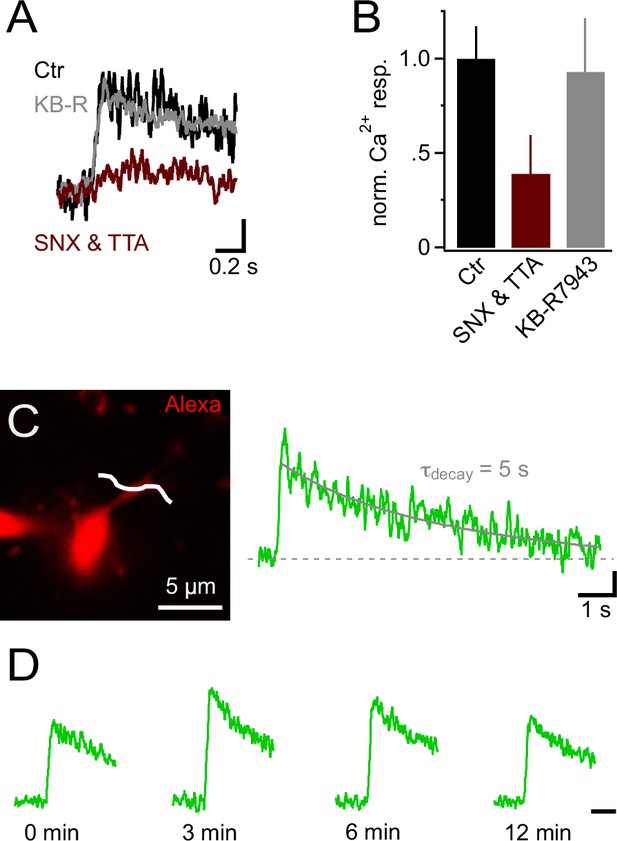
Ca2+ signals in NG2 cells are mainly generated by low-voltage-activated Ca2+ channels.
(A) Ca2+ signals evoked by trains of 10 PSPs (100 Hz) calculated from line-scans across proximal dendrites after 10 min in control or blocker-containing solution. Note the pronounced inhibitory effect of 1 µM SNX-482 (R-type VGCC blocker) and 30 µM TTA-P2 (T-type VGCC blocker) (maroon trace). The amplitudes of Ca2+ signals in each experiment have been normalized on the initial Ca2+ signals (0 min) obtained in the same cell before application of blockers or control solution (initial response not shown). Blocking Na+/Ca2+ exchangers with 10 µM KB-R7943 does not affect Ca2+ signals (grey trace). Scaling: 20% of 0 min Ca2+ signal peak amplitude, 0.2 s. (B) Summary bar graph showing that in SNX-482 and TTA-P2 only 39.1 ± 20.4% of the signal seen in control solution remains after 10 min (n=11, 5 and 5 for control, SNX&TTA and KB-R7943 groups, respectively). (C) 2-photon scan of proximal dendrite of NG2 cell used for recording the Ca2+ signal shown on the right. The white line indicates the position of line-scan. The long line-scan profile on the right illustrates the decay of the signal. The grey line indicates the exponential fit yielding τdecay. Scaling: 0.05 ∆F/F, 1 s. (D) Ca2+ signals can be repetitively evoked in NG2 cells. Line-scan profiles show recordings from the same dendritic location. The amplitudes of Ca2+ signals at each time point have been normalized on the initial Ca2+ signals (0 min). Scaling: 0.5 s.
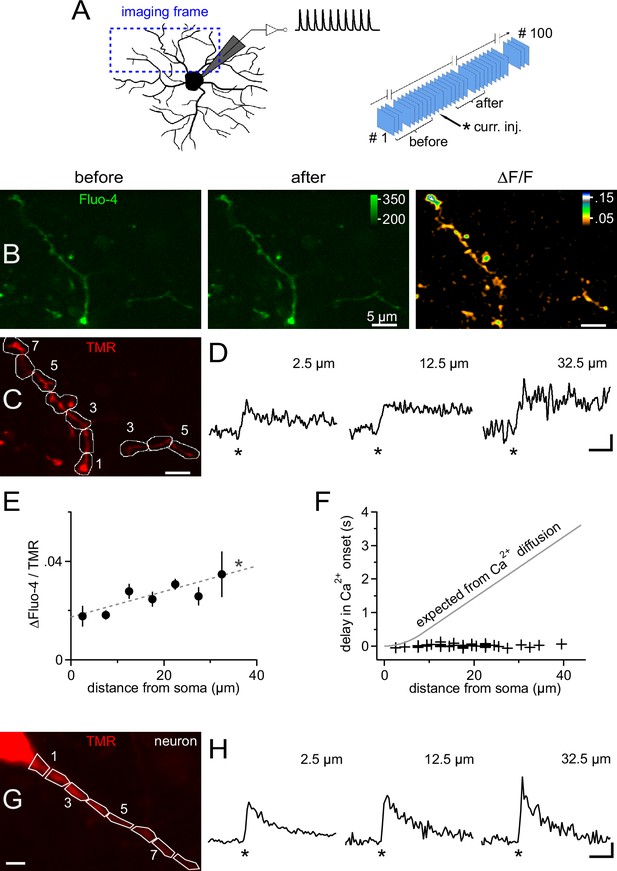
Ca2+ transients are global and instantly occur in distal dendrites of NG2 cells.
(A) Left: Scheme showing the strategy for selecting frames for fast time-lapse acquisition of calcium indicator dye-filled NG2 cells stimulated in current clamp mode (inset on top shows waveform of injected current, as used for Figure 3). Frame-scans include very proximal and terminal dendrites of NG2 cells. Right: Sequence of image acquisition (100 @ 30 Hz). Curly brackets denote frames being averaged for the analysis shown in panels (B–F). (B) Average frame-scan of Fluo-4 fluorescence before (left) and after (middle) mock PSPs. Note the increase in brightness along the entire dendrite. This is better seen in the right image displaying the normalized difference in fluorescence (∆F/F). (C) Corresponding frame-scans of the tracer tetramethylrhodamine-biocytin (TMR). ROIs used for analysis are drawn consecutively with 5 µm increments along the dendrite. ROIs (numbered sequentially) with similar distances to the soma edge are binned for quantification in (E). (D) Calcium signals rise rapidly and with undiminished amplitude at proximal and distal locations. Traces show the time course of the mean fluorescence of ROIs at three different distances expressed as a volume-normalized signal (Fluo-4/TMR) (n=13, 34 and 5 ROIs, respectively). Asterisks indicate time of current injection. Scaling: 0.02 Fluo-4/TMR, 500 ms. (E) Average Ca2+ transient amplitudes slightly increase with the distance from the soma across the dendritic tree (linear correlation test, n=141 ROIs from 12 time-lapse series of seven NG2 cells, R2=0.08; dashed grey line). Peak amplitude values of ∆Fluo-4/TMR were binned according to distance from soma (n=13, 22, 34, 28, 25, 13 and 5 ROIs, respectively). (F) Minimal delay of calcium signals even distally from the soma. Onset of Ca2+ signals was determined for each individual ROI using a template-fitting algorithm (see Materials and methods). If the calcium signal were to propagate by pure diffusion from the somatic site of current injection, substantial delays for remote positions would be expected as denoted by the grey line (see Materials and methods). In order to obtain a precise estimate of the onset of the signal, a detection threshold was chosen (3.0, see Materials and methods) to select signals very clearly standing out of the recording noise (44 ROIs from nine time-lapse series of seven cells). (G) Primary dendrite of a CA1 pyramidal neuron used for a comparative analysis of the dendritic occurrence of Ca2+ signals. ROIs were defined as in (C). Scaling: 5 µm. (H) Ca2+ signals generated in dendrites of CA1 pyramidal neurons, which are known to express dendritic VGCCs, display fast rise times and minimal delays similar to those observed for NG2 cells. Note, however, that neuronal Ca2+ signals decay more rapidly. Neurons were bathed in TTX to prevent action potentials and to make the experiment more comparable to NG2 cells (showing no action potentials). As for NG2 cells, neurons were stimulated with 1 10 pulse 100 Hz train of mock PSPs (n=3). Asterisks indicate time of current injection. Scaling: 1.0 ∆(Fluo-4/TMR)/(Fluo-4/TMR), 500 ms.
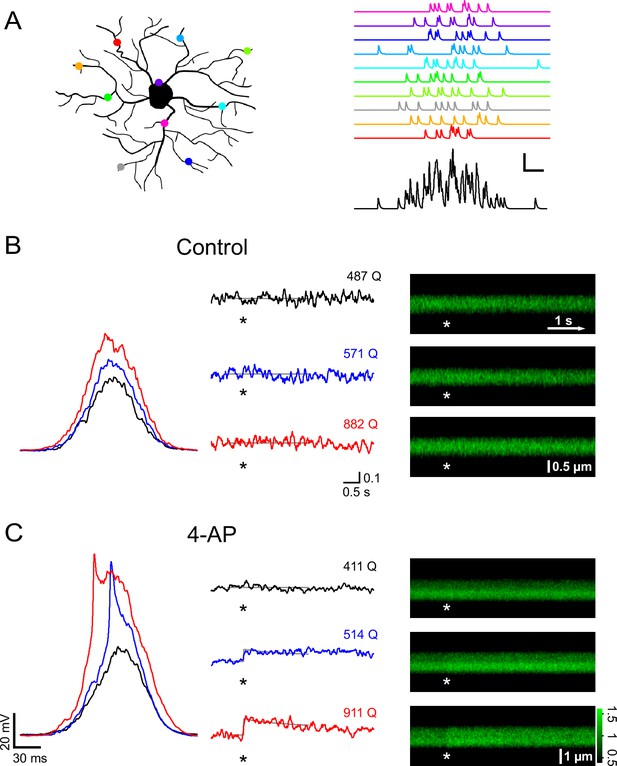
A-type channels restrictively gate Ca2+ signals that are induced by coincident firing of presynaptic neurons.
(A) Left: Cartoon illustrating how active synapses (colored dots) may be distributed across the dendritic tree of a NG2 cell. Right: synaptic currents resulting from the synapses color-coded on the left. Each synapse releases 10 vesicles randomly following a Gaussian distribution with a standard deviation of 25 ms. The black trace on the bottom shows the summation of the synaptic currents. Scaling: 20 pA, 20 ms. (B) Current clamp recording and 2-photon calcium imaging in the proximal dendrite of a control group NG2 cell. Randomly distributed mock synaptic currents produce a Gaussian-shaped voltage response in the soma (left), which scales with input strength. For each round of stimulation, we drew a new set of random numbers from the Gaussian distribution and generated a new current injection waveform by adding quantal currents at time points assigned by the random numbers. Numbers above the traces indicate the total number of quanta being injected (normalized to a reference Rin of 250 MΩ). Note that there is no increase in calcium-indicator fluorescence in the line-scan profiles (middle) or in the line-scans (right) even with the strongest stimulation (indicated by asterisks). Grey lines in the line-scan profiles show the fitted template (see Materials and methods). Same scale bars apply to B and C unless noted differently. Unit vertical scale bar: ∆F/F. (C) As in (B) but for a NG2 cell of the 4-AP treated group (4 mM, applied ~10 min before data acquisition). Note the prominent spikelet riding on the two stronger compound synaptic voltage responses, which were never seen without the A-type channel blocker. Further, calcium responses are more readily seen at lower injection strengths. The line-scan on the bottom right shows a very clear response occurring immediately with the stimulation. Grey lines represent fitted templates. Color scale indicates the level of fluorescence in line-scans normalized on the pre-stimulus baseline.
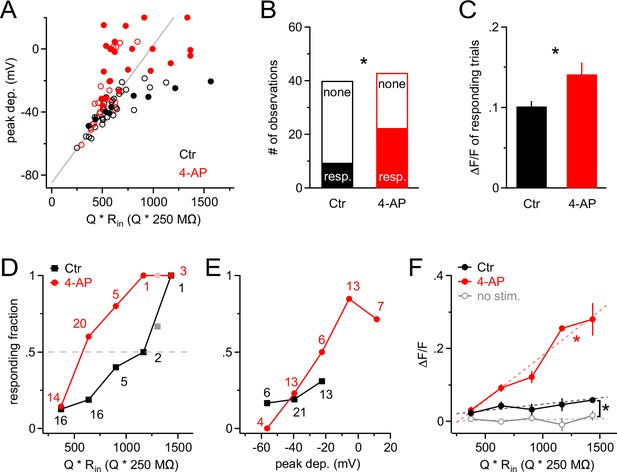
A-type K+ channels strongly increase the threshold for synaptic input to generate Ca2+ signals in NG2 cells.
(A) Scatter plot of the peak of the depolarization response to Gaussian-shaped mock PSP injections shown in Figure 6 versus injection strength for control (black; n=40 trials from nine cells) and 4-AP (red; n=43 trials from seven cells) groups. Peak depolarization is determined as the maximum membrane depolarization reached during a Gaussian-shaped mock PSP. Filled and empty circles indicate trials with and without a Ca2+ response, respectively. The grey line indicates how the voltage response size would grow with injection strength in the absence of VGCs. Note that the more strongly NG2 cells are depolarized, the more frequently responding trials are observed, and that 4-AP facilitates the occurrence of large depolarizations at comparable injection strengths (symbols above grey line). The average injection strengths are comparable between the control (615 ± 40.5 Q * Rin[Q * 250 MΩ]) and 4-AP (640 ± 40.8 Q * Rin[Q * 250 MΩ]) groups. (B) The fraction of responding trials is significantly larger in the 4-AP group. (C) Ca2+ responses are also significantly larger in 4-AP-treated NG2 cells (Student’s t-test). Averages are calculated across responding trials only. (D) The fraction of Ca2+-responding trials increases with injection strength to almost unity in both groups. However, the stimulus–response curve is shifted to the left in the presence of 4-AP, such that at much weaker synaptic input, 50% of dendrites respond with a Ca2+ signal (dashed grey line indicates 50% level). Data from individual dendrites have been binned (number of trials per bin indicated by numbers). Lighter color symbols on the right end of the curve denote values resulting from combining trials from the last two bins in each group. (E) Data as in (D) but plotted against the peak of the depolarization instead of input strength. The control group (black) covers only a small depolarizing voltage range and shows no obvious difference to the responding fraction of dendrites from the 4-AP group, indicating that 4-AP primarily changes the voltage response but not the voltage-dependence of the Ca2+ signal. (F) The amplitude of the average Ca2+ response of the whole population of NG2 cell dendrites increases linearly with the strength of mock synaptic input if A-type K+ channels are blocked (linear correlation test, R2=0.68; dashed red line). Averages were obtained across all trials including non-responding dendritic segments. The amplitude of Ca2+ signals in the control group is significantly larger than that in the no-stimulus group (Paired t-test). The grey data line represents an average of no-stimulus trials across both control and 4-AP groups.
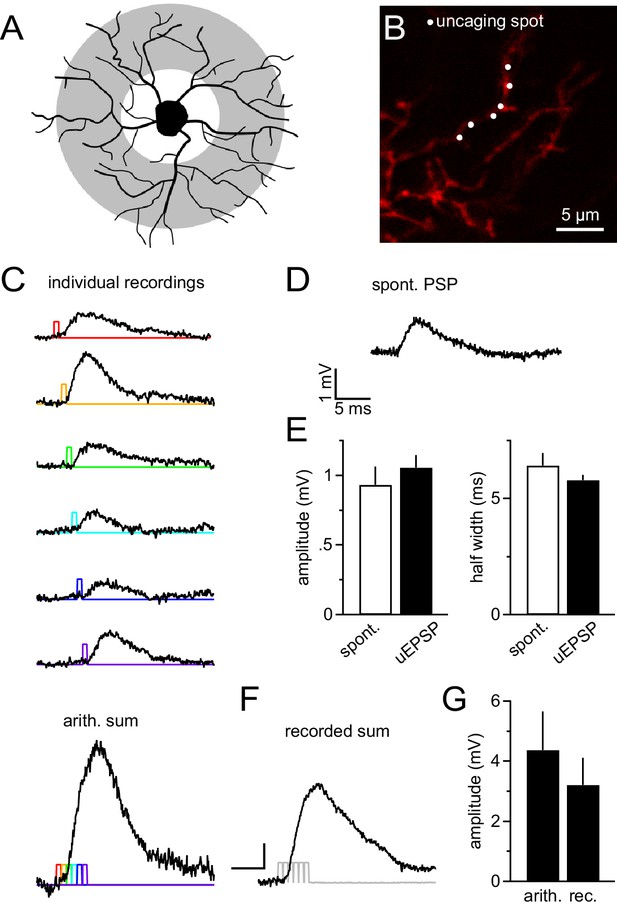
NG2 cell dendrites are highly sensitive to local 2-photon glutamate uncaging.
(A) Grey denotes the target area for 2-photon glutamate uncaging spots on NG2 cells. (B) Dye-filled NG2 cell in acute slice scanned with 2-photon microscopy (25 µM Alexa 594). Six uncaging spots (white dots) were placed along a dendritic segment and were stimulated either sequentially (C) or nearly instantaneously (F). (C) Individual voltage responses (uncaging EPSPs, uEPSPs) of the NG2 cell held in current clamp around –85 mV evoked by sequential photo-release of glutamate at the six spots shown in (B). Color-coded lines indicate the timing of the laser light pulses. The trace at the bottom represents the arithmetic sum of the individual voltage responses. To make this sum comparable to the 'recorded sum' shown in (F), traces were slightly time-shifted (as indicated by the colored laser pulses) to match the unavoidable delay of laser pulses when aiming at near instantaneous uncaging. (D) Example recording of a spontaneously occurring PSP in NG2 cells. Note that these PSPs are almost indistinguishable from uEPSPs with respect to their amplitude and kinetics. (E) Quantification of amplitude and half width of uEPSP (n=51 of five cells) and spontaneous fast PSPs (n=9 of eight cells). Note the similarity between groups. (F) uEPSP evoked by almost simultaneous uncaging at six positions (0.1 ms interval between spots to provide time for moving the laser scanning mirrors). Scaling: 1 mV, 5 ms. (G) Arithmetic and recorded sums are very comparable (n=6 dendrites from two cells, Paired t-test). To correct for any time-dependent run-down (found to be ~10%), the sequence of individual responses was acquired before and after the simultaneous six-pulse uncaging and the bar graph depicts the average of both.
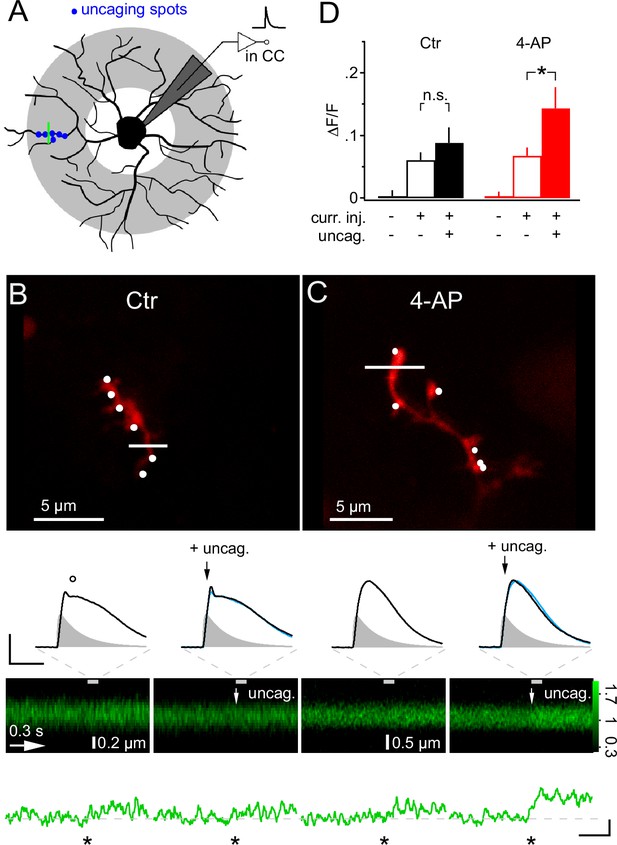
Local glutamate uncaging triggers 4-AP-dependent rapid Ca2+ signaling in NG2 cells dendrites.
(A) NG2 cells were patch-clamped and stimulated with mock PSPs in current clamp mode. Dendritic target area for uncaging and imaging is shaded in grey. In this experiment, we used a quantal IPSC waveform to generate the mock PSP. The green line indicates strategy for selecting line-scans, typically within the region of the uncaging spots (blue dots). (B) Adding glutamate uncaging to mock PSP stimulation does not alter the Ca2+ response in control conditions. Frame-scan shows the position of the six uncaging spots (white dots, 0.1 ms or 1 ms interval) and the line-scan used to quantify any Ca2+ response of Fluo-4 (white line). Second row, left: The voltage response is hardly increased when simultaneous glutamate uncaging at the six indicated positions is added to the mock PSP current injection. Note the small dip (circle) after the peak of the voltage response, which is caused by activation of A-type K+ channels. Right: The first uncaging pulse is added 5 ms after the start of the current injection, denoted by black arrows. The blue line indicates the control mock PSP for comparison (scaling: 20 mV, 40 ms). Injected current waveform derived from miniature IPSCs are shown as grey shaded areas. Note that the voltage responses are brief compared to the Ca2+ signal and are shown on an expanded time scale indicated by the grey dashed lines. Third and fourth row: The line-scans (the grey bar indicates the interval during which the voltage responses have been acquired) and corresponding spatially averaged profiles (green traces on bottom) do not show visually identifiable responses. However, note that the Ca2+ increases generated by mock PSP injection alone and by combined application of mock PSP and local glutamate uncaging (indicated by white arrow) were not significantly different (asterisk indicates time of current injection). (C) When A-type K+ channels are inactive, local glutamate uncaging significantly boosts dendritic Ca2+ signal. Panels as in B but for an NG2 cell from the 4-AP group. Note that in the presence of 4-AP, glutamate uncaging (black and white arrows) hardly increases the voltage response when compared to the response to mock PSP injection alone (left). The small dip seen in control conditions after the peak of the depolarization is absent. The line-scans and the line-scan profiles show a clear Ca2+ response when mock PSP and six spots of glutamate uncaging are co-applied. Scaling: 0.2 ∆F/F, 0.3 s. (D) Increase in fluorescence of the calcium indicator dye Fluo-4 is significantly boosted by additional uncaging if A-type K+ channels are inhibited. ∆F/F values were calculated from periods before and soon after (100 ms) the stimulation or during baseline period with no stimulation of line-scan profiles shown in (B) and (C). Control: n=26 dendrites of 10 cells; 4-AP: n=30 dendrites of 16 cells.
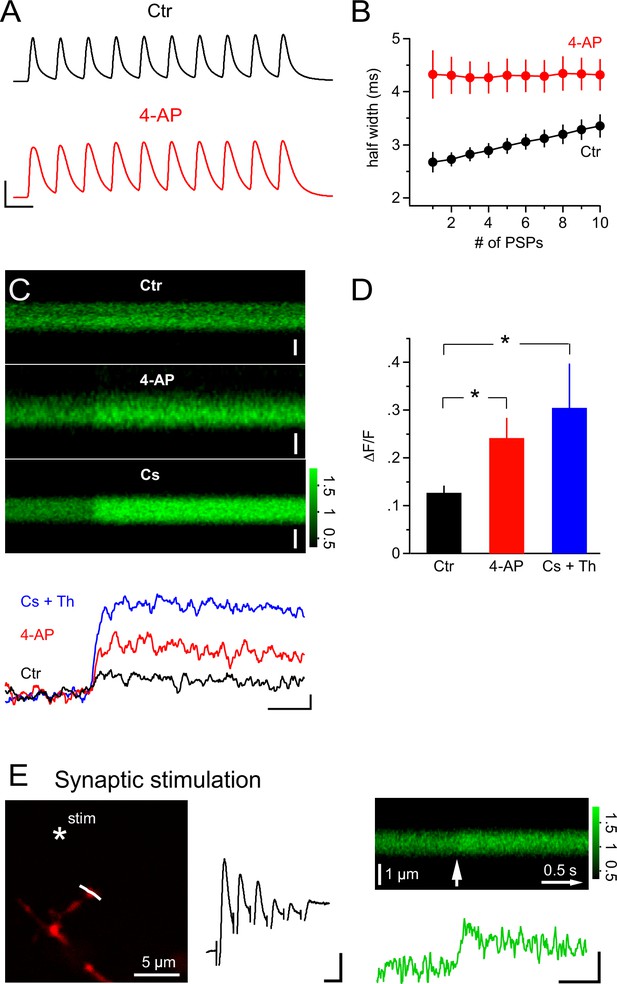
PSPs inactivate A-type K+ channels and synaptic stimulation induces Ca2+ transients independent of Ca2+ stores.
(A) Example recordings of trains of mock PSPs (100 Hz, 100 ms) in control and 4-AP groups. Note that the half-width of PSPs in the control group progressively increases during the train. By contrast, PSPs in the 4-AP group are broader in the beginning and their half-width does not increase during the train. Scaling: 50 mV, 10 ms. (B) Activity-dependent broadening of PSPs in a train of the control group. Average half-width of PSPs (n=7) is smaller in the control group and approaches that of PSPs in the 4-AP group (n=8) during a 10 stimuli train. (C) 2-photon line-scans (top) and corresponding profiles (bottom) of Fluo-4 fluorescence in dendrites of NG2 cells during injection of mock PSPs in control, 4-AP and Cs+ groups. Note the more pronounced Ca2+ signal in the presence of 4-AP, which is probably due to the increased width of PSPs enhancing Ca2+ tail currents. Scaling: 1 µm (control), 0.5 µm (4-AP) and 1 µm (Cs+) for line-scans; 0.1 ∆F/F, 0.5 s for line-scan profiles. All line-scans and line-scan profiles shown are the average of three repetitions. Note that 1 µM thapsigargin was included in the pipette solution for the Cs+ group. (D) The average ∆F/F induced by a 100 Hz 100 ms train of PSPs is significantly increased in the 4-AP group. (Student’s t-test: control vs 4-AP group). Blocking K+ conductance by using Cs-gluconate-based internal solution also significantly increases the ∆F/F induced by train PSPs (Wilcoxon-Mann-Whitney test: control vs Cs+ group, see Materials and methods). (E) Left: Frame-scan (Alexa 594) shows the position of the stimulating electrode (asterisk) and the line-scan (white line) used to quantify the fluorescence response of the Ca2+ indicator dye Fluo-4 (200 µM). Middle: Electrical response measured at the soma of the NG2 cell held in current clamp mode. Note the small amplitude of the response, which typically ranged between 5.1 and 10.1 mV (5 or 6 stimuli at 100Hz). Scaling: 2 mV, 10 ms. Right top: Average line-scan of Fluo-4 signal from three repetitions of local synaptic stimulation at the location shown in (A). The white arrow indicates the time of the first of six synaptic stimulations. Right bottom: Synaptically induced Ca2+ signal calculated from line-scan showed similar kinetics and amplitude as calcium signals evoked by current injection or glutamate uncaging. The presence of 1 µM thapsigargin in the pipette solution excludes the potential contribution of internal calcium stores to the Ca2+ response. Scaling: 0.2 ∆F/F, 0.5 s.
Additional files
-
Supplementary file 1
The information of all statistical tests used in the text or figures, including the sample size, the names of the statistical tests, exact P values and additional information.
- https://doi.org/10.7554/eLife.16262.012