Control of the structural landscape and neuronal proteotoxicity of mutant Huntingtin by domains flanking the polyQ tract
Figures
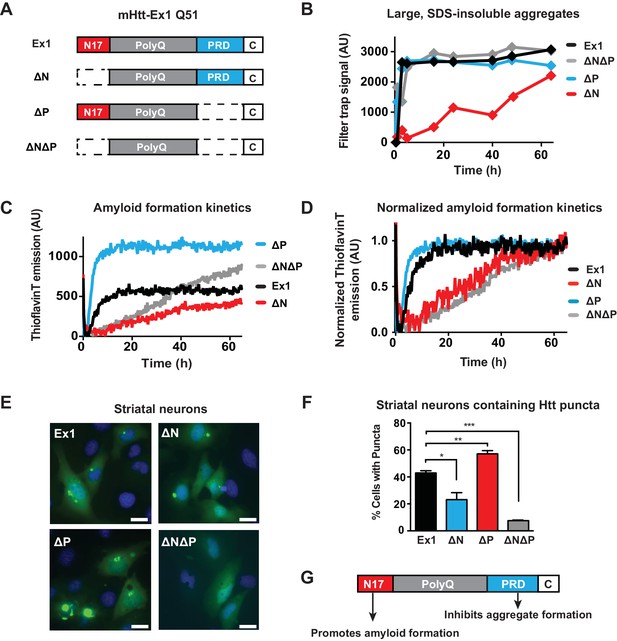
Flanking regions impact pathogenic mHtt aggregation propensity in vitro and in striatal neurons.
(A) Schematic representation of polyQ expanded mutant Htt-Ex1 variants (mHtt-Ex1) used in this study. mHtt-Ex1 contains N17 (red), an expanded polyQ tract (grey), the proline-rich domain ('PRD', blue), as well as a short, 10-amino acid C terminal tail ('C', white). Variants were generated by deleting the regions flanking the polyQ domain. An additional C-terminal S tag (not shown) was used for immunoblot detection of recombinantly produced mHtt. For recombinant expression of all mHtt variants, a 51-mer polyQ tract was used. (B) Kinetics of formation of SDS-insoluble, heat-stable aggregates for the mHtt-Ex1 variants as measured by the filter trap assay. Aggregation of purified recombinant mHtt-Ex1 variants from (A) was initiated by cleavage of a solubilizing N-terminal GST tag by TEV protease. All aggregation reactions were performed at a concentration of 3 µM. Data is representative of at least three independent experiments. (C) Rate of accumulation of amyloid aggregates for the mHtt variants measured by the ThioflavinT fluorescence assay. All variants were aggregated at a concentration of 3 µM. (D) Normalized curves of ThioflavinT amyloid aggregation kinetics from (C) used to compare kinetic rates. Variants without the N17 region (∆N, ∆N∆P) form amyloids much more slowly than variants with the N17 region (Ex1, ∆P). (E) Fluorescence images of each of the mHtt mutants transfected into the ST14a striatal neuron-derived cell line. mHtt variants were constructed similar as in (A) with a 51-mer polyQ length. Instead of a N-terminal GST and C-terminal S-tag, constructs had only a C-terminal GFP tag. Images were taken 48 hr post transfection. Scale bar is 20 µm. (F) Percentage of transfected cells containing aggregates for each of the Htt mutants as shown in (E). Similar to as seen in vitro deletion of the N17 region leads to overall less aggregation while deletion of the PRD leads to overall more aggregation. Data are mean ± SEM of three independent experiments counting at least 150 cells. *p <0.05, **p<0.005, ***p<0.001. (G) Summary model for how the N17 and PRD regions contribute to mHtt aggregation propensity.
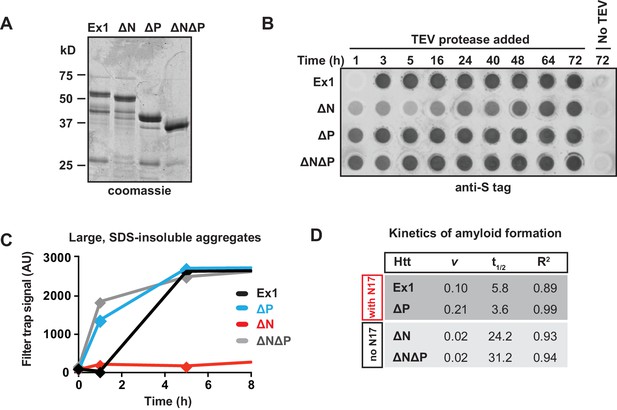
Additional data and modeling of aggregation kinetics.
https://doi.org/10.7554/eLife.18065.004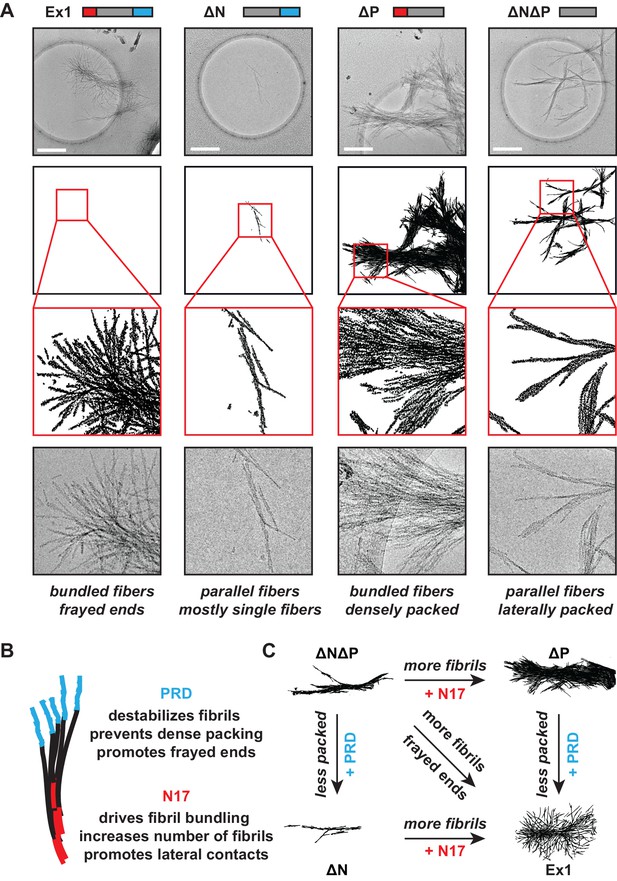
Morphology of mHtt aggregation highlight how N17 and PRD contribute to mHtt aggregation.
(A) Morphology of fiber aggregates from each of the mHtt mutants by cryo-EM. mHtt fibers were imaged 24 hr post initiation of aggregation. Scale bar is 500 nm. Top row is the cryo-EM micrographs. The second and third rows are traced annotations of the EM micrographs and zoom-ins of individual areas, respectively. Bottom row is the original cryo-EM micrograph of the zoomed-in area. (B) Summary of the N17 and PRD contributions to aggregation morphology and propensity. (C) Summary model for how the N17 and PRD regions direct morphology of mHtt aggregates. Morphology differences between ∆N∆P and Ex1 showcase how the flanking regions impact mHtt aggregation in a combinatorial manner.
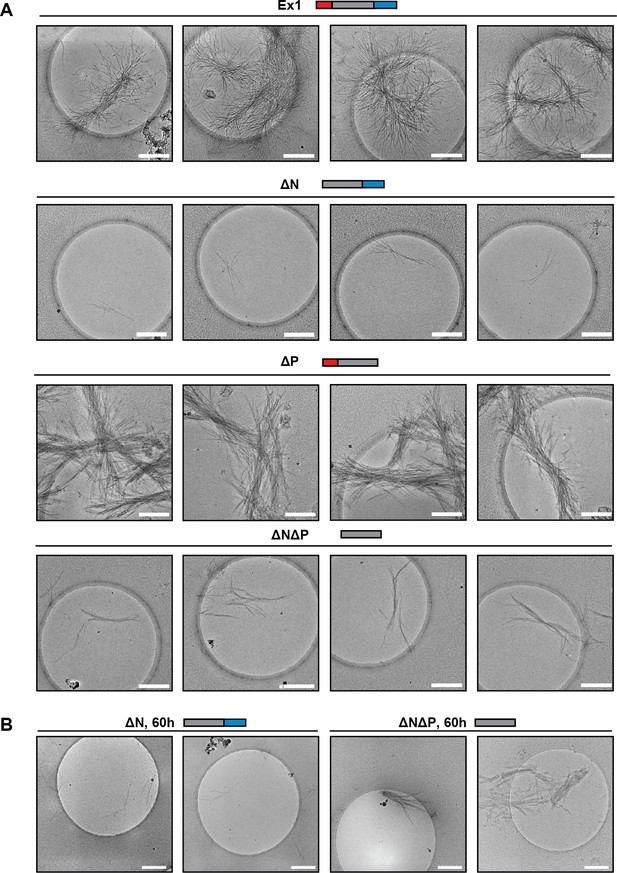
Additional cryo-EM images of mHtt variant aggregate morphology.
https://doi.org/10.7554/eLife.18065.006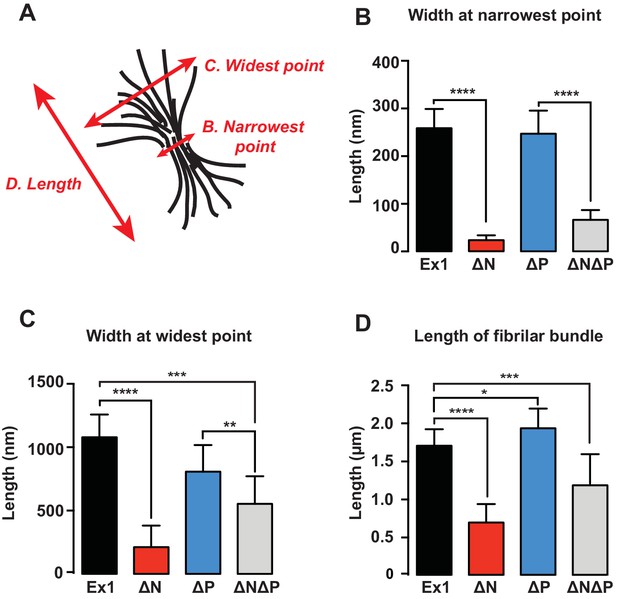
Quantification of mHtt variant aggregate morphology.
https://doi.org/10.7554/eLife.18065.007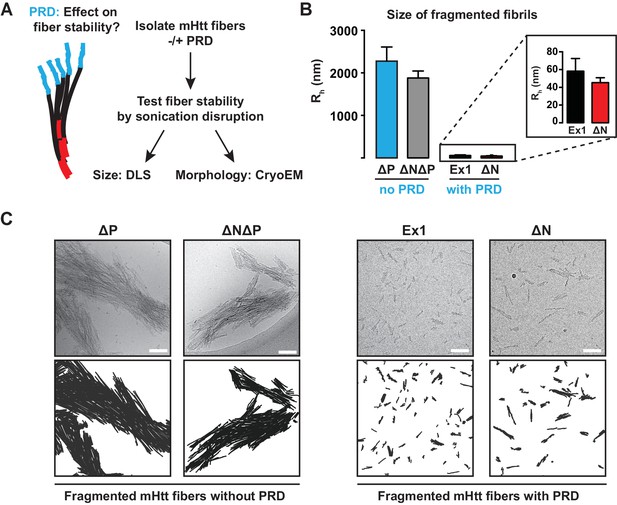
The PRD region mechanically destabilizes mHtt aggregates.
(A) mHtt fibers were isolated by centrifugation and sonicated to test their mechanical stability. Sonicated species were analyzed by cryo-EM imaging and Dynamic Light Scattering (DLS). (B) Size comparisons between sonicated mHtt aggregates lacking PRD (∆N∆P, ∆P) and mHtt variants with the PRD (Ex1, ∆N) as measured by DLS. Sonication of mHtt variants containing the PRD results in much smaller fiber fragments than those lacking PRD. Data are mean ± SEM. (C) Cryo-EM images of sonicated fibers for mHtt variants lacking PRD (∆N∆P, ∆P) or mHtt variants with the PRD (Ex1, ∆N). Scale bar is 100 nm.
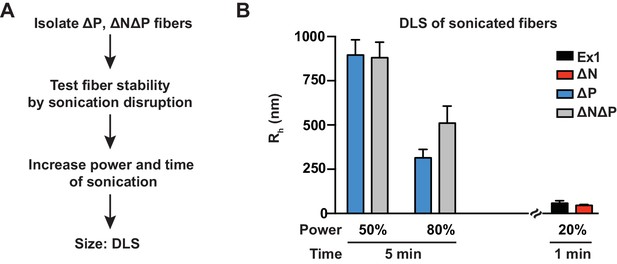
DLS data of sonicated mHtt variants at harsher conditions.
https://doi.org/10.7554/eLife.18065.009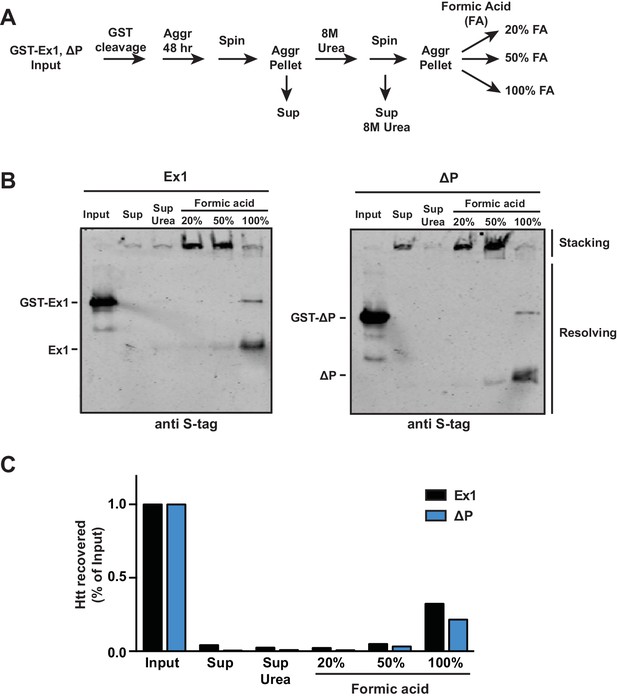
Comparison of Ex1 and ∆P susceptibility to urea and formic acid denaturation.
https://doi.org/10.7554/eLife.18065.010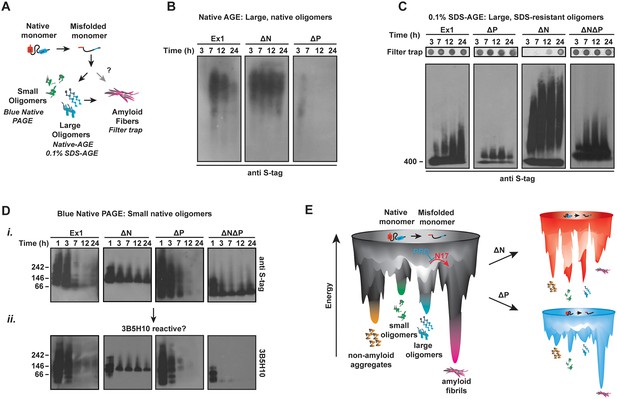
N17 and PRD direct the formation and stabilization of oligomer populations.
(A) Schematic of oligomer and aggregate populations generated during the mHtt aggregation pathway. We characterized the oligomer populations using Agarose Gel Electrophoresis (AGE) under either native or mildly denaturing, 0.1% SDS conditions or Blue-Native PAGE under native conditions. Oligomers were isolated by taking time-points of the in vitro mHtt aggregation reaction, conducted at the same conditions as in Figure 1. (B) Native-AGE showing native, large oligomers for the mHtt variants. ∆N generated a large population of mHtt oligomeric species that persisted through the aggregation reaction, whereas ∆P generated very few oligomeric species that disappeared quickly from the gel. Blot was immunoprobed for the C-terminal S-tag on the mHtt variants. (C) 0.1% SDS-AGE gel showing partially SDS-soluble, oligomers over 400 kDa. Blot was immunoprobed for the C-terminal S-tag on the mHtt variants. Large, SDS-insoluble mHtt aggregates do not enter the SDS-AGE gel, as shown by the reference filter trap (top row, filter trap). ∆N generates few aggregates because the protein remains trapped in the oligomeric range. (D) Blue-Native PAGE gel showing native, small oligomers below 400 kDa. Aggregation time-points were run in a Blue-Native PAGE gel and immunoprobed for the S-tag (i) and the 3B5H10 conformational antibody (ii). 3B5H10 only recognizes the smaller oligomers of the mHtt variants Ex1, ∆N, and ∆P. (E) Energy landscape model of the different conformational species in the mHtt-Ex1 aggregation pathway (left). Aggregation through the ∆N or ∆P pathways changes the energetic barriers between the oligomeric and fibrillar states of mHtt (right).
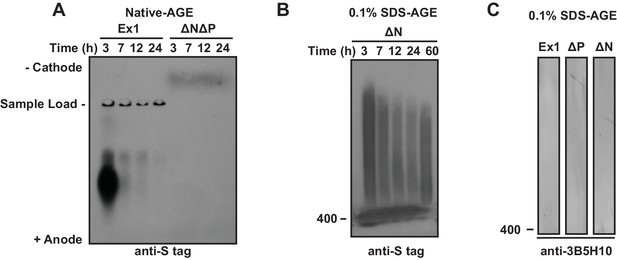
Additional 0.1% SDS-AGE and Native-AGE assays of mHtt-Ex1 variants.
https://doi.org/10.7554/eLife.18065.012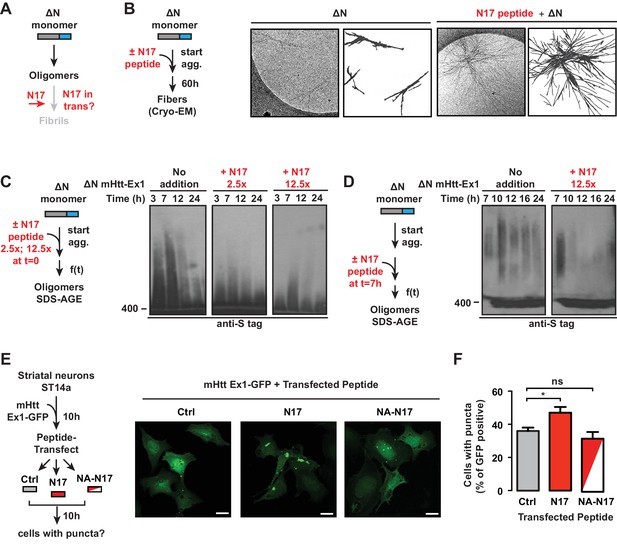
N17 is essential to promote transition of oligomers to amyloid aggregates.
(A) General schematic of trans N17 addition experiments to ∆N aggregation reaction to determine how N17 impacts the balance between stable, ∆N oligomers and amyloid fibrillar aggregates. (B) 12.5x excess of N17 peptide was added when ∆N aggregation was initiated. Then 60 hr after initiation of aggregation, the fibers were analyzed by cryo-EM. N17-seeded ∆N aggregates become more bundled and more resemble the morphology of Ex1 aggregates (Figure 2A). (C) 12.5x or 2.5x excess of N17 peptide was added in trans when ∆N aggregation was initiated. The impact of N17 peptide on ∆N oligomers was analyzed by 0.1% SDS-AGE gels and immunoprobed for the C-terminal S-tag. Trans addition of N17 promotes disappearance of these stable ∆N oligomers from the AGE gel. (D) 12.5x excess of N17 peptide was added in trans 7 hr after initiation of ∆N aggregation, allowing for the formation of ∆N oligomers before N17 addition. Impact of N17 peptide on ∆N oligomers was analyzed by 0.1% SDS-AGE gels and immunoprobed for the C-terminal S-tag. (E) Schematic of in vivo N17 addition experiment: ST14a striatal-derived neurons were transfected with the C-terminally GFP tagged mHtt-Ex1 (mHtt-Ex1-GFP). 10 hr after transfection, when expressed mHtt protein is still completely soluble, cells are protein transfected using the Xfect kit (Clontech) with N17 or a mutant N17 peptide (NA) that inhibits aggregation. After 10 hr, resulting cells containing GFP fluorescent puncta are counted. (F) Fluorescence microscopy of ST14a striatal neurons transfected with mHtt-Ex1-GFP and N17 variant peptides (left) and quantification of cells containing puncta (right). Data are mean ± SEM of three independent experiments with at least 200 cells counted in each condition. Scale bar is 20 µm. *p<0.05.
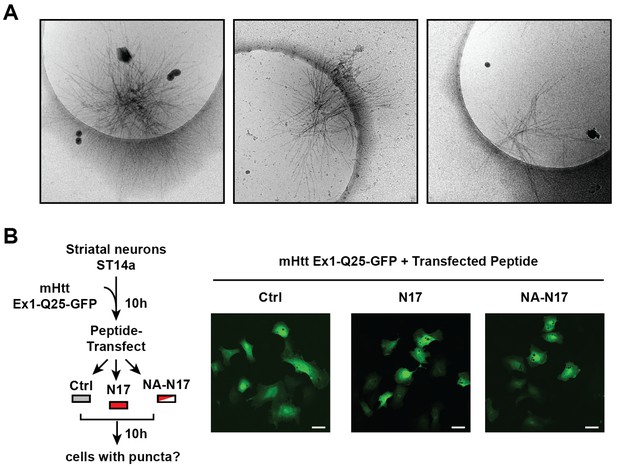
Additional cryo-EM images of ∆N aggregates with N17 peptide added in trans and transfection of N17-peptide into neurons expressing Htt-Q25.
https://doi.org/10.7554/eLife.18065.014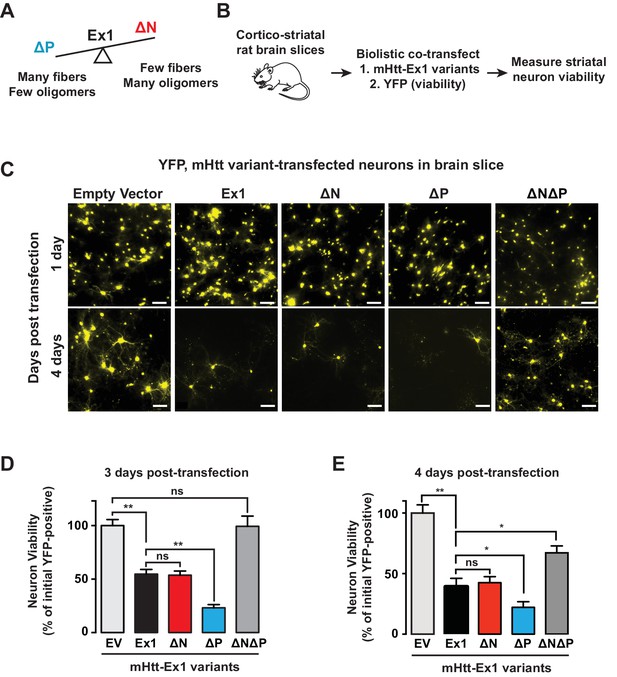
Assessing proteotoxicity of mHtt variants in corticostriatal brain slices.
(A) Compared to Ex1, ∆N forms many more oligomers and much fewer fibrillar aggregates, whereas ∆P forms many more fibrillar aggregates and much fewer oligomers. (B) Schematic of toxicity assay: Corticostriatal slices were prepared from rat brains and biolistically co-transfected with mHtt-Ex1 variants or an Empty Vector control (EV) and an independent YFP plasmid as a marker of cell viability. Viability of medium spiny neurons (MSNs) was visually assessed by cell morphology and YFP expression after 3–4 days post-transfection. (C) Fluorescence images of MSNs from the brain slices co-transfected with the mHtt variants and YFP. Images were taken 1 day (top row) and 4 days (bottom row) after transfection. Data are representative of at least three independent experiments. Scale bar is 20 µm. (D) Relative viability of MSNs transfected with mHtt variants 3 days after transfection. Data are mean ± SEM. Data are representative of at least three independent experiments. **p<0.0001 (E) Relative viability of MSNs transfected with mHtt variants 4 days after transfection. Data are mean ± SEM. Data are representative of at least three independent experiments. *p<0.01, **p<0.0001

Transfected Htt-Ex1 is only toxic in transfected MSNs at pathogenic Q-lengths.
https://doi.org/10.7554/eLife.18065.016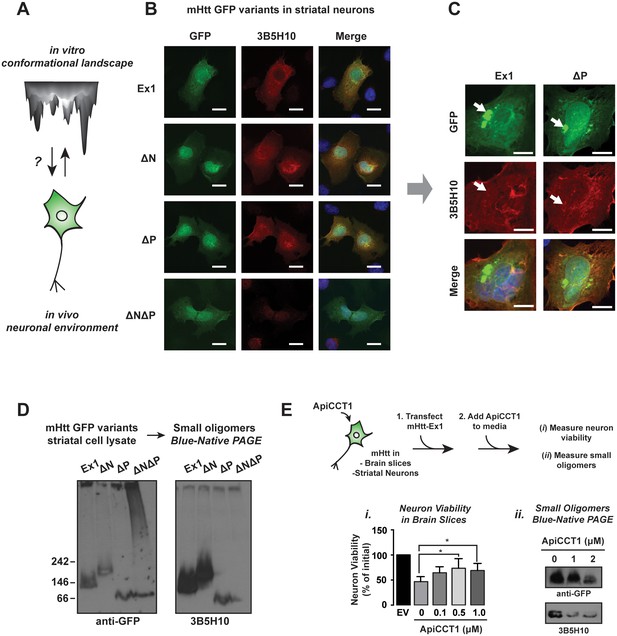
Impact of PolyQ flanking domains of the formation of soluble oligomers in vivo.
(A) We investigate the impact of the neuronal environment on the mHtt conformational landscape. (B) Confocal imaging of ST14a striatal-derived neurons transfected with mHtt-Ex1 variants C-terminally tagged with GFP. Cells were imaged in the GFP channel well as immunostained with the 3B5H10 polyQ conformational antibody. Scale bar is 20 μm. (C) Confocal images of Ex1 and ∆P-transfected ST14a cells containing puncta. Cells were imaged in the GFP channel as well as immunostained with the 3B5H10 polyQ conformational antibody. The 3B5H10 antibody shows no specific recognition of the GFP puncta. Scale bar is 10 μm. (D) Immunoblots of lysates from ST14a striatal neurons transfected with mHtt-Ex1 variants C-terminally tagged with GFP and run in Blue-Native PAGE gels. Blots were probed for GFP (left) and the 3B5H10 conformational antibody (right). (E) Schematic for ApiCCT1 addition experiment: Corticostriatal brain slices (i) and ST14a striatal-derived neurons (ii) were transfected with mHtt-Ex1 simultaneously with exogenous addition of ApiCCT1 to media. Then, viability of MSNs in the corticostriatal brain slices was measured (i) and 3B5H10-positive small oligomers in the striatal neuron extracts were characterized (ii). Data are mean ± SEM. **p<0.01.
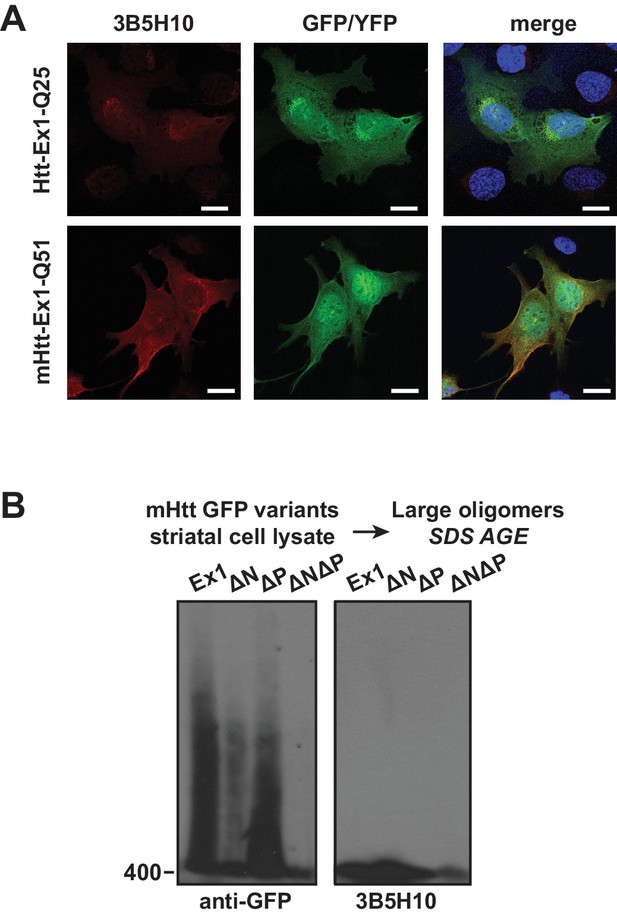
Immunofluorescence images and 0.1% SDS-AGE of ST14a cells transfected with Htt-Ex1 variants and immunoprobed with 3B5H10.
https://doi.org/10.7554/eLife.18065.018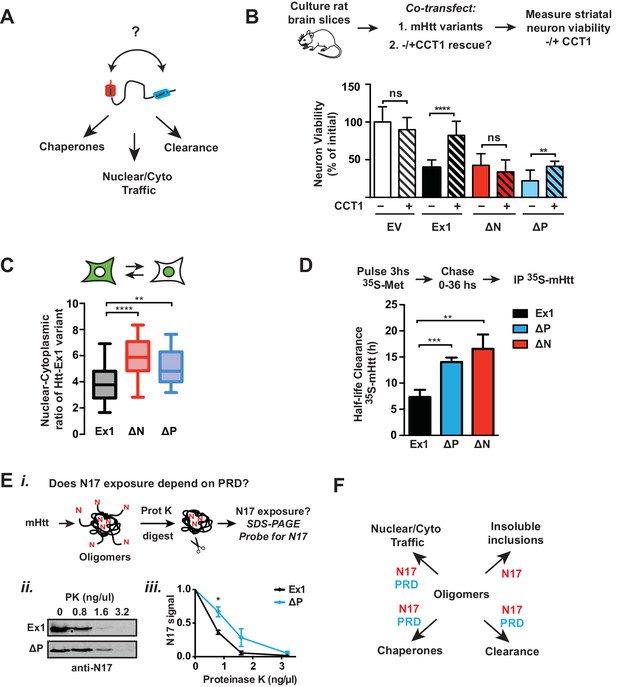
Flanking domains act synergistically in vivo to determine mHtt fate.
(A) Htt flanking domains engage various cellular proteostasis pathways (B) Relative viability of MSNs from rat brain slices biolistically co-transfected with the toxic mHtt variants, YFP, and the CCT1 subunit of the TRiC chaperonin. Viability was quantified 4 days after transfection. Data are mean ± SEM. Data are representative of at least three independent experiments. ****p<0.0001 (C) Nuclear and cytosolic distribution of GFP-tagged mHtt variants transfected into ST14a neurons. Distribution was quantified by measuring the ratio of mean intensity between nuclear and cytosolic fluorescent signals of individual cells. Data are mean ± SEM with at least 20 cells counted per condition. ****p<0.0001, **p<0.01 (D) 35S pulse-chase measuring soluble protein degradation of mHtt-Ex1 variants in transfected ST14a cells. After transfection, cells were pulsed with 35S methionine for 3 hr and chased with complete media. Respective time points were lysed and subject to a clearing spin to only characterize soluble mHtt. Soluble mHtt was immunoprecipitated using the GFP tag. (E) (i) Schematic of ProteinaseK (PK) digestion experiment: Ex1 and ∆P oligomers were generated in vitro, then digested with increasing concentrations of ProteinaseK. Protease-digested reactions were run in a SDS PAGE gel and probled for N17. (ii) SDS-PAGE gel of ProteinaseK-digested Ex1 and ∆P oligomers immunoprobled for N17. (iii) Quantification of the N17 signal from the SDS-PAGE gel in (ii). Data are mean ± SEM. *p<0.05. (F) Model for how the polyQ flanking regions contribute to mHtt protoestasis and cellular mechanisms for clearance of toxic species.
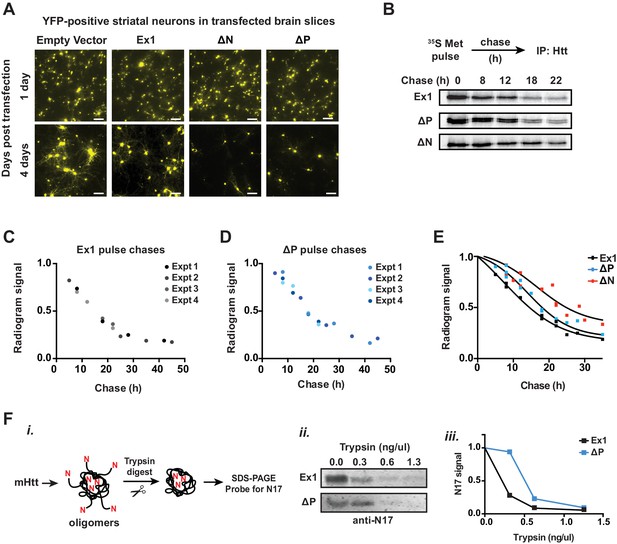
Trypsin sensitivity of Ex1 and ∆P oligomers and 35S pulse-chase measuring protein degradation for mHtt-Ex1 variants.
https://doi.org/10.7554/eLife.18065.020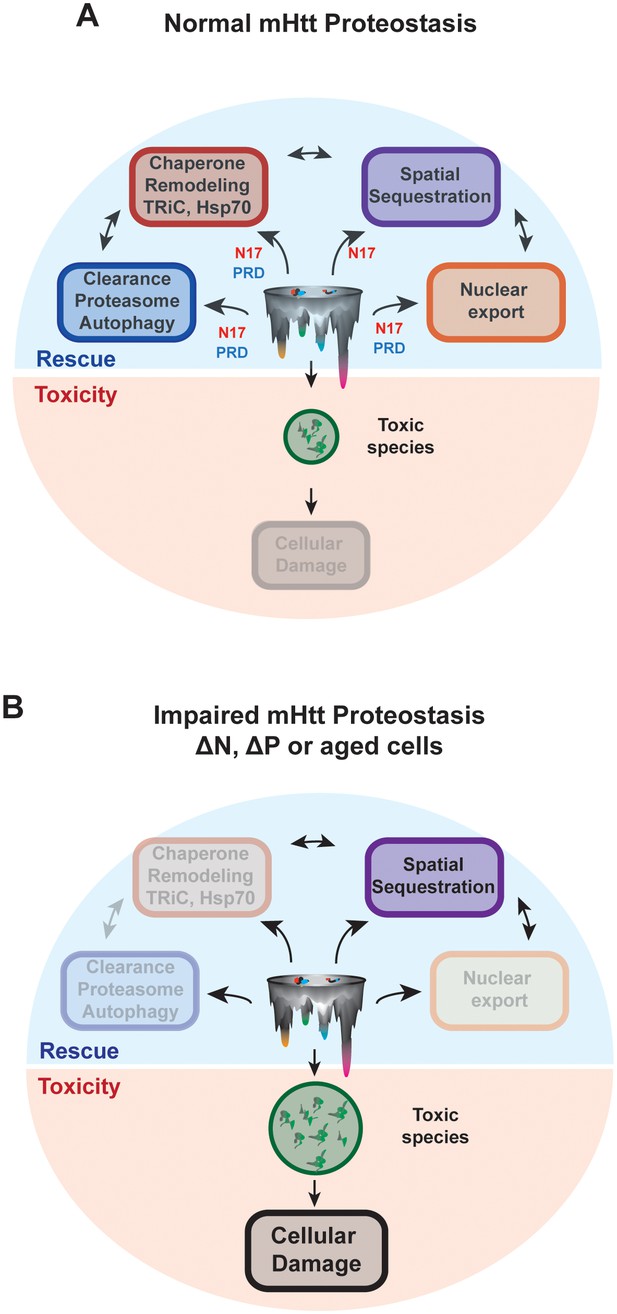
N17 and PRD control the conformational landscape and cellular fate of mHtt in vivo.
(A) Normal HD proteostasis can occur through the coordination of several protein quality control pathways to clear toxic species from the cell, minimizing cellular damage: (1) protein degradation, (2) remodeling through molecular chaperones, (3) spatial sequestration through insoluble aggregation, and (4) export of toxic species from the nucleus. (B) Impaired HD proteostasis either in aged systems or altered mHtt translation products, creating ∆N or ∆P-like constructs. Here, normal proteostasis pathways – such as interaction through molecular chaperones, protein degradation machinery or integrity of proper nuclear-cytoplasmic trafficking – are diminished. The only mode of clearance of toxic species is through spatial sequestration, resulting in the accumulation of mHtt aggregates. However, the kinetics of this process may be insufficient to clear all toxic, soluble species, thereby allowing their accumulation and amplifying cellular damage.
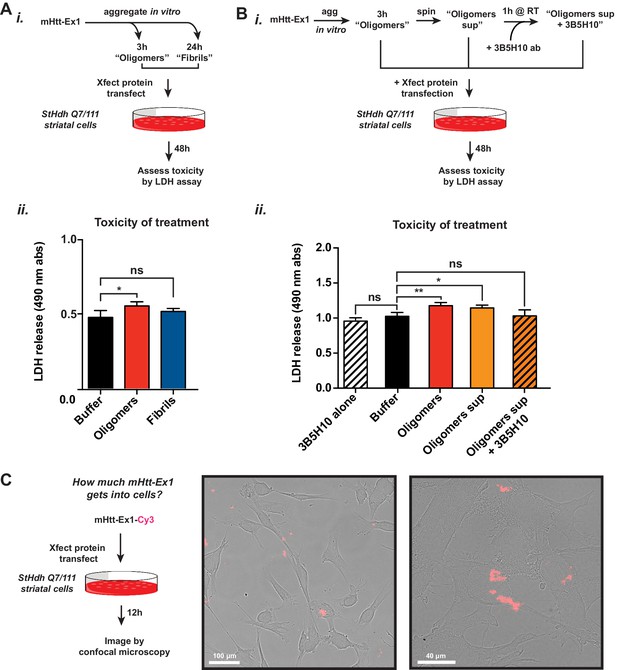
Exogenous addition of mHtt-Ex1 oligomers and aggregates to striatal cells
A. i. Experimental schematic of generating mHtt-Ex1 oligomers and aggregates in vitro and their exogenous addition to StHdhQ7/111 knock-in striatal cells. mHtt-Ex1 species were added to cells using protein transfection (Xfect kit, Clontech). Toxicity was assessed via LDH assay (Promega) 48h after their addition. ii. Measurement of LDH release as a proxy for cell death. Buffer control was the original sample buffer for the mHtt-Ex1 in vitro species. Data are mean ± SD. *p < 0.05. B. i.Experimental schematic. Experimental set-up was similar to (A) with the addition of a spin to procure just the soluble supernatant of the aggregation reaction after 3h. The supernatant was also pre-incubated with the 3B5H10 polyQ conformational antibody for 1h at RT to allow binding between any soluble oligomeric species and the 3B5H10 antibody. ii. Measurement of LDH release as a proxy for cell death. Data are mean ± SD. *p < 0.05, **p < 0.01 C.Left: Experimental schematic. mHtt-Ex1 was labeled with fluorescent Cy3-maleimide dye, then added to StHdh- Q7/111 striatal cells using the Xfect protein transfection kit, similar to (A) and (B). After 12h, cells were fixed and imaged via confocal microscopy (Right) to determine proportion of mHtt-Ex1 that gets into cells.