Nucleophile sensitivity of Drosophila TRPA1 underlies light-induced feeding deterrence
Figures
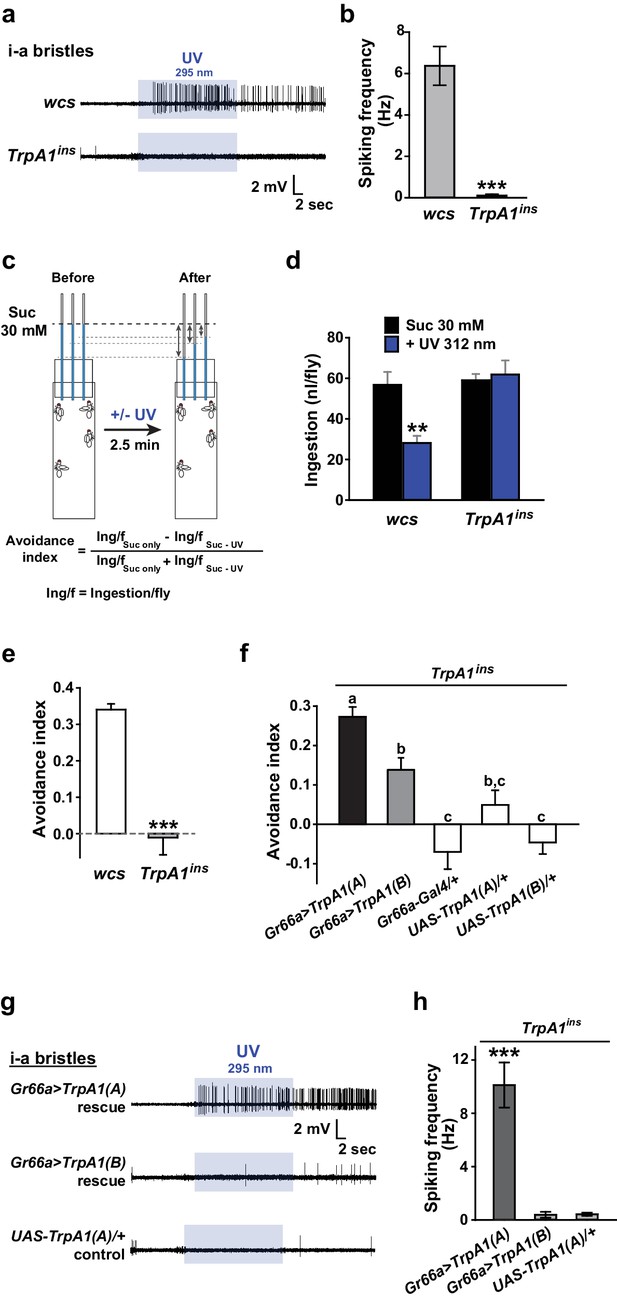
UV-induced taste neuron firing and feeding deterrence require TrpA1(A) but not TrpA1(B).
(a) Representative UV responses from i-bristles of the indicated genotypes. wcs: white canton S (wild type). TrpA1ins: TrpA1 knockout flies. Recording taken under 5.2 mW/cm2 UV illumination is marked by purple boxes. (b) Averaged data from a (n = 4–5). (c) Schematic illustration of modified Café assays used to test UV-induced feeding deterrence. (d) Ingestion amount/fly with or without 1.3 mW/cm2 312 nm UV illumination in wcs and TrpA1ins. (e) Suppression of feeding by UV illumination in the indicated genotypes, presented as an 'avoidance index' (n = 5–6). (f) Reintroduction of transcript variants TrpA1(A) and TrpA1(B) cDNAs differentially restores UV avoidance of TrpA1ins. Letters indicate statistically distinct groups (p<0.05, n = 8–12, Tukey’s test). (g) Isoform-dependent rescue of UV-evoked neuronal responses in TrpA1ins. (h) Summary of g (n = 5–6). *p<0.05, **p<0.01, ***p<0.001, Tukey’s, Student’s t- or Mann-Whitney U tests.
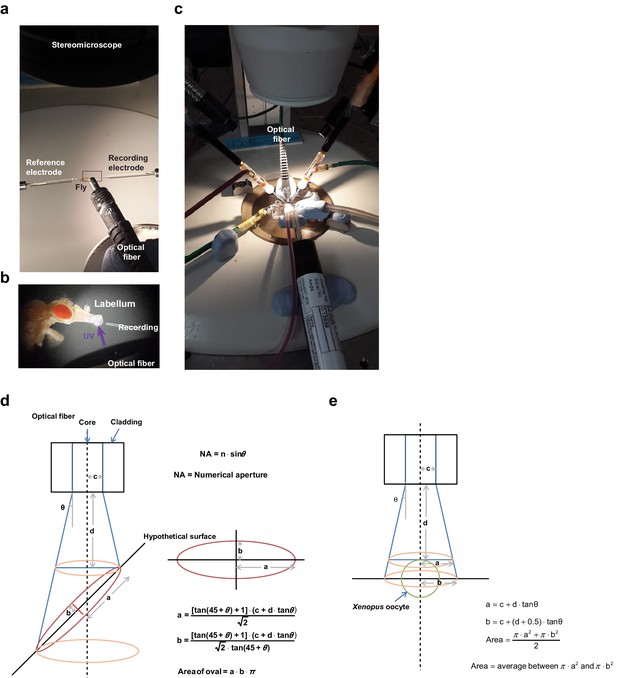
Setups for electrophysiological recordings of UV-evoked responses in in vivo taste neurons and Xenopus oocytes and estimation of light irradiance at the illuminated tissue.
(a) Extracellular tip recording configured with the UV-emitting optical fiber cable. (b) Magnified image of the inset in (a). (c) Two-electrode voltage clamping setup with the UV-emitting optical fiber cable. (d) An oval shape of the illumination surface resulting from the 45° angle between the light beam and the surface of the tissue was postulated to simplify estimation of the surface area of taste bristles contacting the light. NA values and inner diameters (2c) of the fiber core were given by the manufacturers (n = 1 for air in conversion of NA to the angle theta). Distance to the surface (d) was measured microscopically using a fine ruler. (e) Different considerations were used to calculate light intensity for TEVC, as the configuration between the fiber and oocytes is simple and invariant.
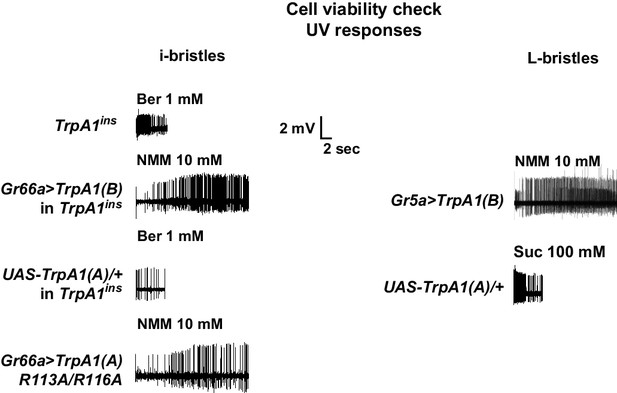
Cell viability check for non-responders in extracellular recording experiments.
The non-responding bristle sensilla in the experiments on UV responsiveness were stimulated by known tastants activating the corresponding cells. For i- and L-bristles, 1 mM berberine and 100 mM sucrose were used, respectively. In particular, non-responder cells expressing TRPA1 channels were tested with electrophilic NMM, which activates TRPA1s.
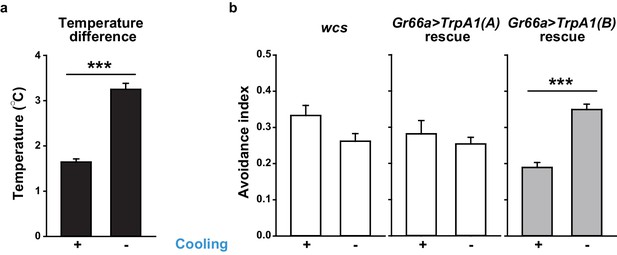
Temperature changes in Café assays that measure UV-dependent feeding avoidance.
(a) Temperature increases inside of vials with (n = 21) or without (n = 8) active air-cooling. (b) UV-induced feeding avoidance was temperature-dependent only for those expressing highly temperature-sensitive TRPA1(B) (n = 4–9). ***p<0.001, Student’s t-test or Mann-Whitney U test.
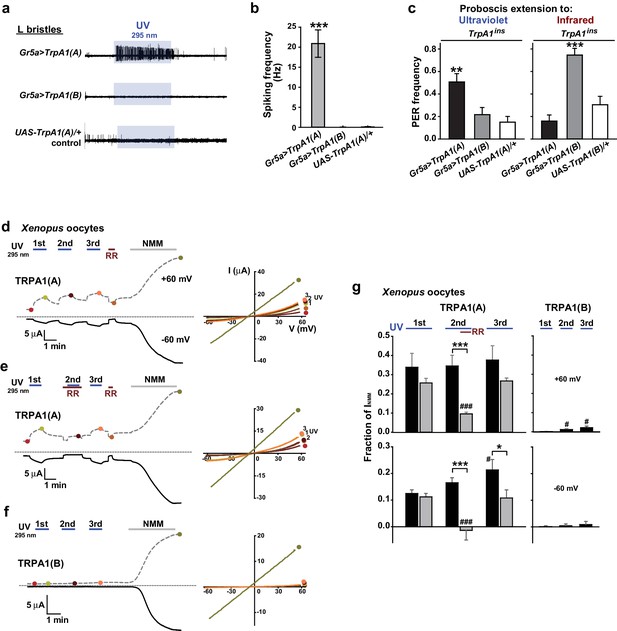
TRPA1(A) expression is sufficient for UV responsiveness.
(a) UV- and isoform-dependent action potentials in sweet-sensing neurons exogenously expressing TRPA1 isoforms. (b) Summary of a (n = 4–5). (c) Proboscis extension reflex (PER) to UV (n = 24–25) and IR (n = 22–24) in TrpA1ins flies ectopically rescued in sweet taste neurons. (d-f) Typical UV-evoked currents in Xenopus oocytes expressing the indicated isoforms. RR: 0.2 mM ruthenium red. NMM: 0.1 mM. Right, Current-voltage (IV) relationships at the indicated points in the Left panels. (g) Summary of d–f. UV responses normalized to NMM currents at +60 and −60 mV, respectively (n = 4–5). #: p<0.05, ###: p<0.001, ANOVA Repeated Measures test compared to the first response (n). *p<0.05, **p<0.01, ***p<0.001, Tukey’s, Student’s t- or Mann-Whitney U tests.
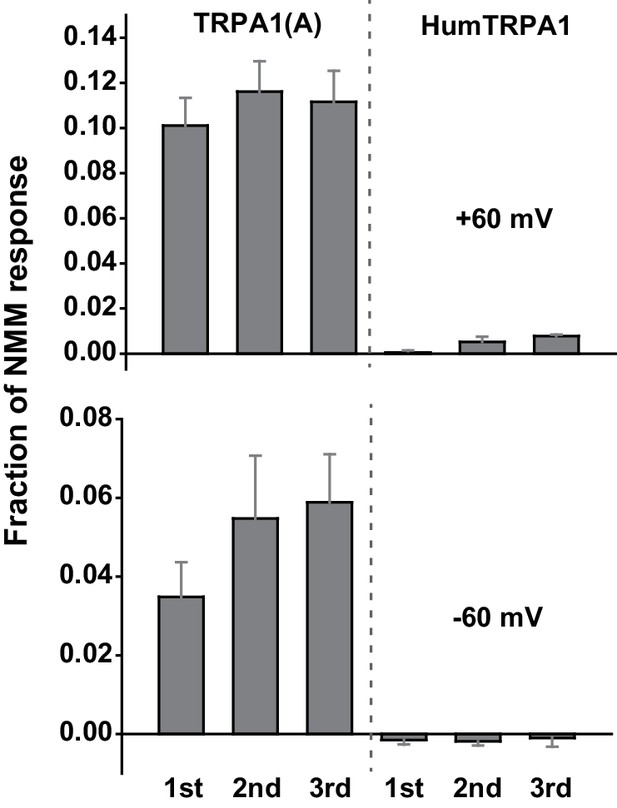
Human TRPA1 (humTRPA1) is not activated by the same UV intensity as Drosophila TRPA1(A).
Three serial 295 nm UV pulses at 5.2 mW/cm2 were administered to oocytes expressing the indicated TRPA1s. The UV-elicited current amplitudes at +60 or −60 mV were normalized to the 0.1 mM NMM-evoked current and averaged (n = 4–8).
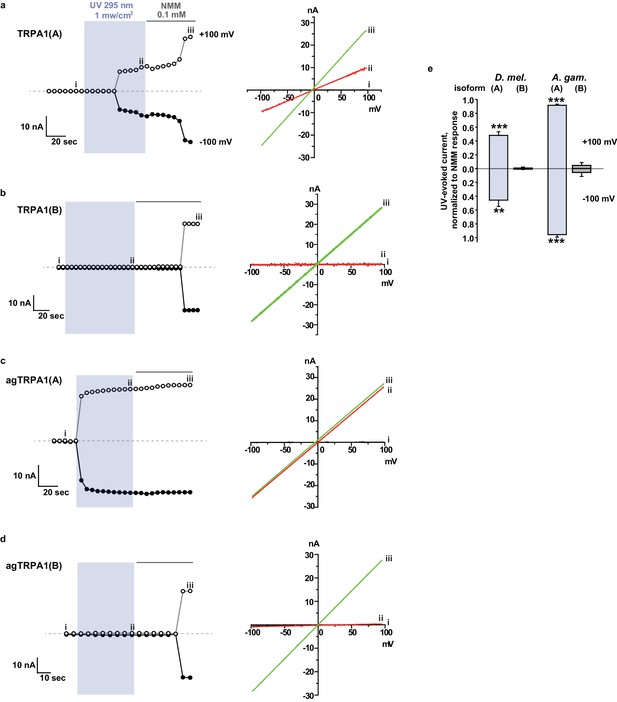
TRPA1(A)s from flies and mosquitoes do not need the cytosol of Xenopus oocytes for UV responsiveness.
(a–d) Inside-out macropatches excised from Xenopus oocytes were subjected to electrophysiological recording under 295 nm UV illumination at the indicated intensity. Left: currents at -100 and +100 mV. Right: IV curves at the marked recorded points. (e) Current amplitudes were averaged and presented as bar graphs (mean and SEM, n = 3–4). **p<0.01, ***p<0.001, ANOVA Tukey’s test.
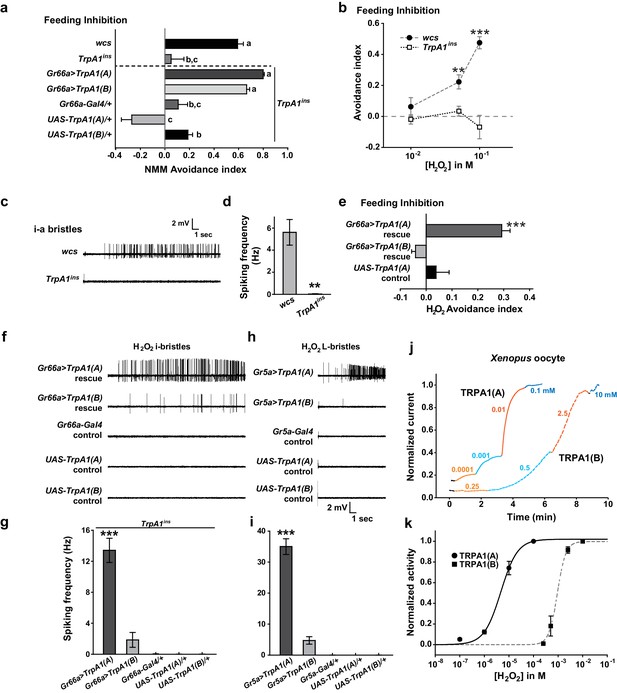
Responses of Drosophila TRPA1 to nucleophilicity-bearing H2O2are isoform-dependent.
(a) Electrophilic NMM-induced feeding avoidance is not isoform-dependent (n = 3–4). Letters indicate statistically distinct groups (p<0.01, Tukey's test). (b–d) Feeding deterrence (b) and neuronal responses (c and d) to H2O2 are abolished in TrpA1ins (b: n = 3–11, d: n = 8–14). (e–i) Isoform-dependent rescue of H2O2 feeding avoidance (e, n = 4) and neuronal activation (f–i, n = 6–29) in TrpA1ins. j and k, Typical H2O2 current recordings normalized to the maximum H2O2 response (j) and H2O2 dose-dependence (k, n = 4–11) of TRPA1 isoforms in oocytes. Alternating colors represent increasing concentrations of H2O2 as indicated. **p<0.01, ***p<0.001, Tukey’s or Student’s t-tests.
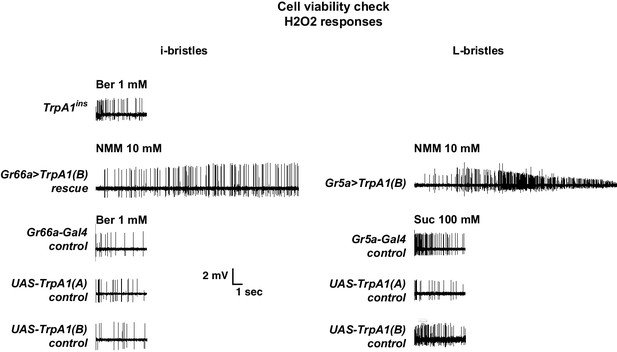
Cell viability check for non-responders in extracellular recording experiments.
The non-responding bristle sensilla in the experiments examining H2O2 responsiveness were stimulated by other tastants activating the corresponding cells. For i- and L-bristles, 1 mM berberine, and 100 mM sucrose were used, respectively. Non-responding cells expressing TRPA1 channels were tested with electrophilic NMM, which activates all TRPA1 channels.
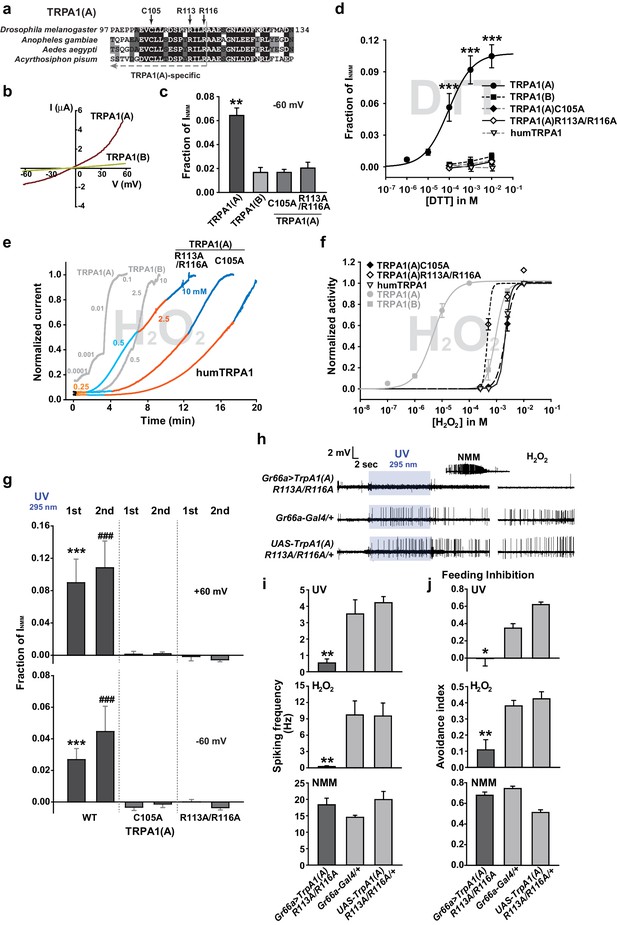
The sensitivity to nucleophilic dithiothreitol correlates with the UV and H2O2responses of TRPA1(A).
(a) Sequence alignment of TRPA1(A) N-terminal regions from indicated insects. Conserved residues that were substituted with alanine are indicated by arrows. (b) Representative current-voltage relationships in oocytes expressing TRPA1s. (c) Averaged basal activity of the indicated TRPA1 isoforms, normalized to NMM current (n = 7–37). (d) DTT dose dependence of TRPA1s, normalized to NMM current (n = 4–10). (e) Typical H2O2 responses of mutant TRPA1(A)s and human TRPA1 (humTRPA1) in comparison with WT TRPA1(A) and TRPA1(B). (f) H2O2 dose dependence of TRPA1s in e (n = 4–6). (g) UV-evoked currents at +60 and −60 mV from WT and mutant TRPA1(A)s normalized to NMM current (n = 4). *** or ###: comparison among first or second responses, respectively. (h) Typical extracellular recordings for UV or H2O2-induced action potentials from i-bristles expressing TrpA1(A)R113A/R116A through Gr66a-Gal4. Inset: NMM response from the UV-non-responder presented underneath. (i) Summary of h (n = 6–12). Response to the electrophile NMM was unimpaired despite severe attenuation of UV and H2O2 responses upon expression of TrpA1(A)R113A/R116A. (j) Similarly to neuronal responses, feeding deterrence to UV and H2O2 was repressed by expression of TrpA1(A)R113A/R116A (n = 4–8). **p<0.01, *** or ###p<0.001, Tukey’s test.
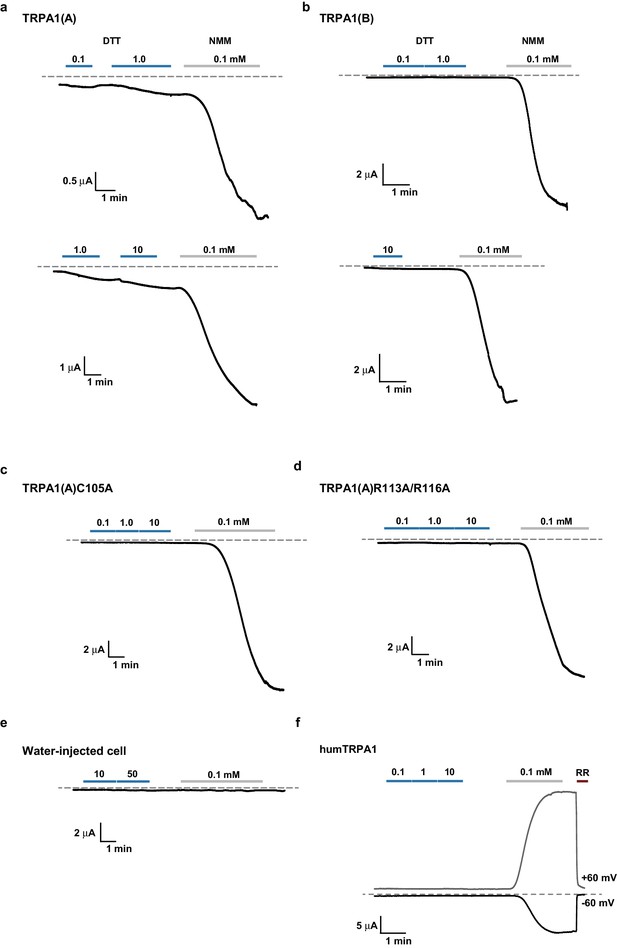
Representative TRPA1 currents evoked by DTT in Xenopus oocytes.
DTT-induced currents were compared with responses to 0.1 mM NMM for TRPA1s as indicated. (a–e), Voltage-clamped at −60 mV. (f) A 300-msec voltage ramp between -60 and +60 mV was applied every second. Currents at either -60 or +60 mV were plotted as a function of time. RR: ruthenium red at 50 μM.
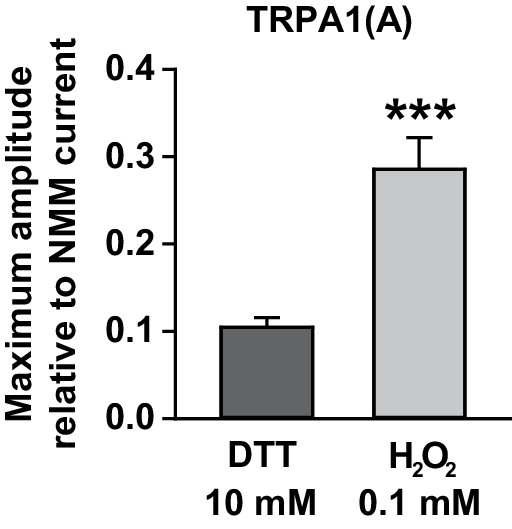
Maximal current amplitudes of TRPA1(A) by H2O2 were intermediate between those of DTT and NMM.
TRPA1(A) currents in Xenopus oocytes that were evoked by either 10 mM DTT (n = 5) or 0.1 mM H2O2 (n = 10) were normalized by currents induced following 0.1 mM NMM application. The indicated concentrations for the chemicals yielded maximal current responses at −60 mV, which were used for estimation of the maximum current amplitudes. ***p<0.001. Mann-Whitney U test.

Expression of TrpA1(A)C105A in bitter-tasting neurons failed to exhibit robust NMM-induced action potentials.
Spiking frequencies of bitter neurons expressing either TrpA1(A)C105A (n = 6) or TrpA1(A)R113A/R116A (n = 7) in TrpA1-deficient mutant animals were plotted. ***p<0.001, Student’s t-test.
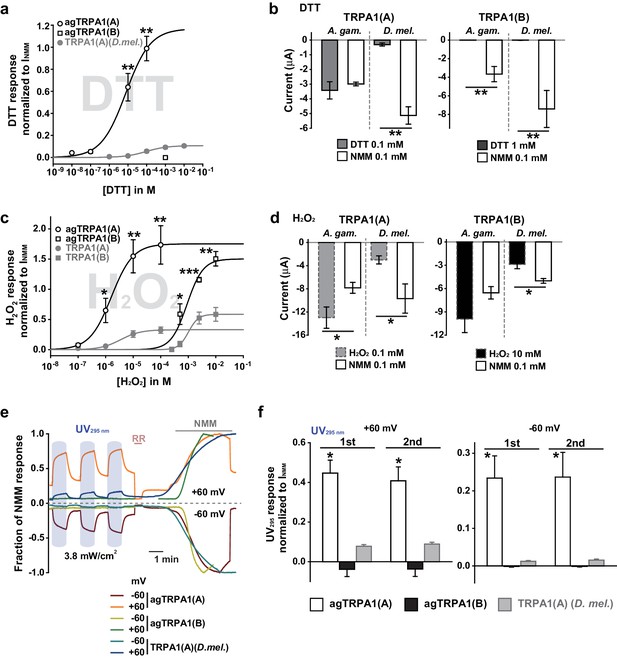
Concomitant natural variations in DTT, UV and H2O2 responsiveness between Anopheles gambiae and Drosophila melanogaster TRPA1(A)s.
(a and b) In Xenopus oocytes, agTRPA1s show isoform dependence to DTT as do their Drosophila counterparts, with larger response amplitudes (n = 5–6). Dose-dependency to DTT (a) and averaged peak current amplitudes evoked by DTT and NMM (b) are presented for the channels, as indicated. (c and d) The robust DTT receptor, agTRPA1(A), exhibits enhanced H2O2 responses compared to Drosophila TRPA1(A) (n = 4–5). Dose-dependency to H2O2 (c) and averaged peak current amplitude (d) are compared between mosquito and fly TRPA1 isoforms. (e and f) agTRPA1(A) responds more robustly to UV light than Drosophila TRPA1(A), while agTRPA1(B) does not. A typical UV-evoked current response of agTRPA1(A) is superimposed on the responses of agTRPA1(B) and Drosophila TRPA1(A) following normalization to the NMM response (e). Normalized UV-elicited current amplitudes averaged for the indicated channels (f, n = 4–12). *p<0.05, **p<0.01, ***p<0.001, Tukey’s and Mann-Whitney U or Student’s t-tests.
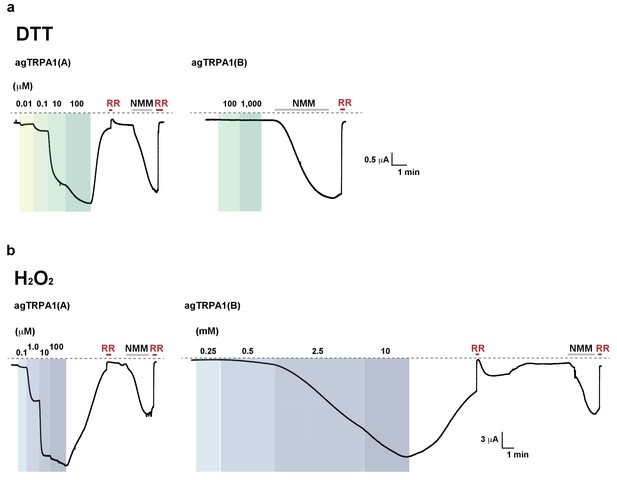
Typical DTT (a) and H2O2 (b) responses of agTRPA1(A) and agTRPA1(B) heterologously expressed in Xenopus oocytes.
RR: 50 μM ruthenium red. NMM: 0.1 mM N-methyl maleimide. DTT and H2O2 concentrations are indicated in the figure. Voltage clamped at −60 mV. The dashed line represents the baseline.
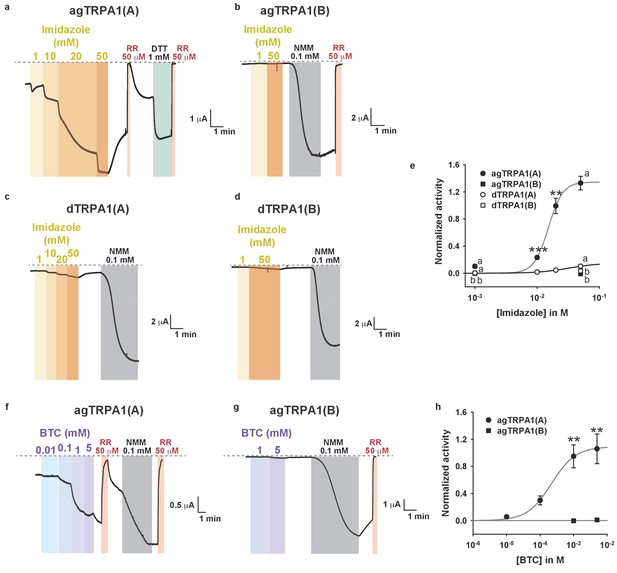
Nucleophiles other than DTT preferentially activate TRPA1(A) over TRPA1(B).
(a–d) Representative current traces from Xenopus oocytes expressing the indicated TRPA1 isoforms upon application of increasing imidazole concentrations (voltage-clamped at −60 mV). Imidazole is the nucleophilic side chain of histidine, four of which form coordinate bonds with oxygen-binding Fe2+ in the heme. (e) Imidazole dose dependences of the indicated TRPA1 channels (n = 3–5). (f–g) Typical current recordings from cells expressing agTRPA1 isoforms in response to benzyl thiocyanate (BTC). BTC is a naturally occurring plant nucleophile, while its isosteric compound benzyl isothiocyanate (BITC) activates TRPA1s because of its electrophilicity (Hinman et al., 2006). (h) BTC dose dependence of agTRPA1s (n = 5–12). The agTRPA1(A) isoform is much more sensitive to the nucleophile BTC than is agTRPA1(B). Letters indicate statistically distinct groups. ANOVA Dunn’s test for comparison between three or more groups. **p<0.01, ***p<0.001. Student’s t- or Mann-Whitney U tests between two groups.
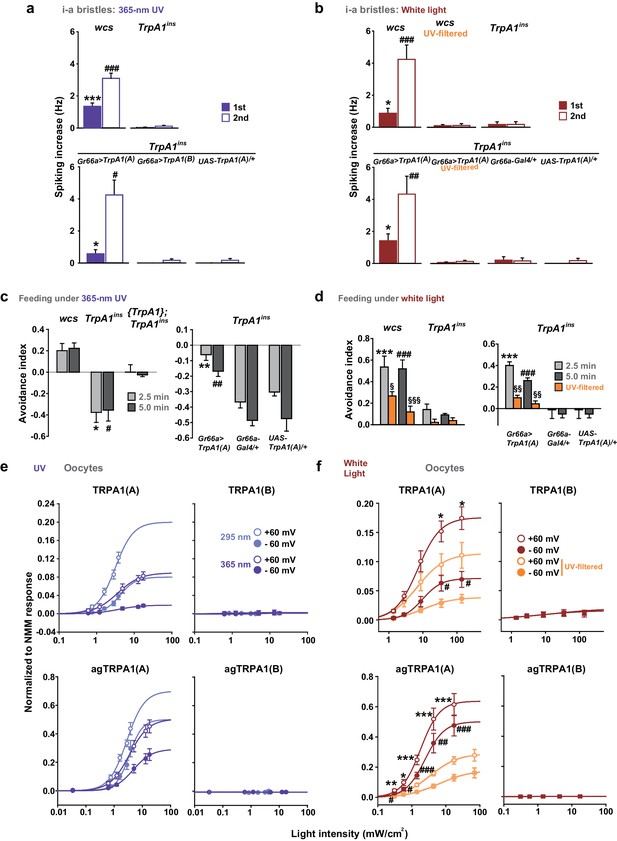
White light activates TRPA1(A) and deters feeding at natural intensities.
Monochromatic UVA at 365 nm (a, c and e) and polychromatic white light (b, d and f) suppress feeding through TRPA1(A). (a and b) Illumination with 365 nm UVA light excites bitter-tasting neurons of i-a bristles at 42.1 mW/cm2, and the response is dependent on TrpA1 (n = 5–8) (a). Polychromatic white light from a Xenon arc lamp stimulates TrpA1-dependent bitter-sensing neurons at 93.4 mW/cm2, which is similar to natural solar intensity (n = 5–9) (b). Neuronal activation by white light requires UV, as it was abolished upon filtering out UV with the thin titanium dioxide-coated glass. * and #p<0.05, *** and ###p<0.001, Student’s t- or Tukey’s test for the first and second illuminations, respectively. (c and d) UVA (c, n = 4–7) or white light illumination (d, n = 4–7) hinders feeding depending on TrpA1(A). UV blocking from white light significantly reduces feeding avoidance (orange bars). * and #p<0.05, ** and ##p<0.01, *** and ###p<0.001, Tukey test for 2.5 and 5 min, respectively. §, §§ and §§§: p<0.05, 0.01 and 0.001, respectively, Student’s t-tests between illuminations with and without the UV filter. (e and f) UV (e, n = 4–8) and white light (f, n = 4–8) intensity dependences of fly (upper) and mosquito (lower) TRPA1 isoforms heterologously expressed in oocytes. UVB at 295 nm (pale purple) produced higher responses than UVA at 365 nm (dark purple) (e). Blocking the UV component of white light significantly reduces the current elicited by white light illumination (f). The half maximal efficacy intensities of UV and white light are given in the text and Supplement file 1. Student’s t-test between illuminations with and without UV filter. * and #, ** and ##, and *** and ###p<0.05, 0.01, and 0.001, respectively (*: +60 and #: −60 mV).
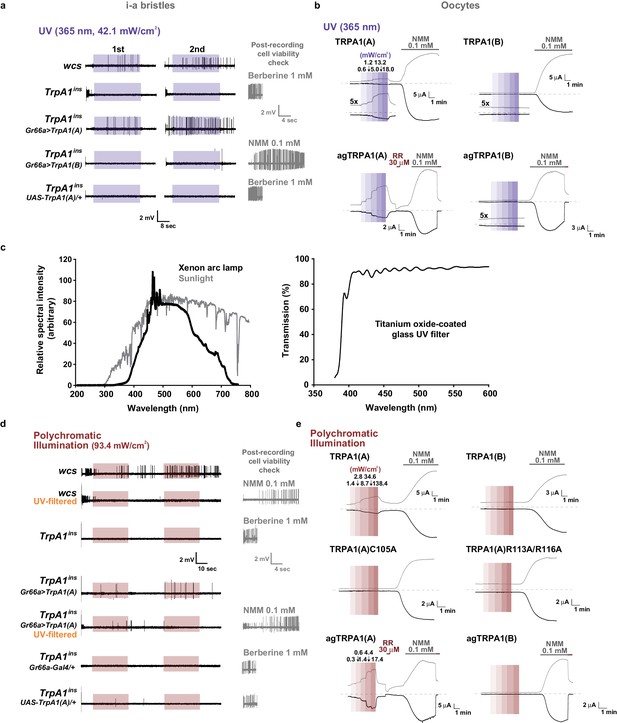
TRPA1(A)-dependent neuronal and heterologous responses to UVA and white light.
(a) Representative sensillum recording results following application of 365 nm UVA light. The right panels confirm the viability of non-responders. (b) Typical TEVC recording results with the indicated TRPA1 isoforms from flies (upper) and mosquitoes (lower). Insets marked with ‘5x’ show current traces magnified five times for clear presentation, while scale bars are drawn for 1x traces. (c) Left, relative spectral intensities of the Xenon arc lamp (black, DG4, Sutter instruments, NJ, USA) or sunlight on the ground (grey). Right, light transmission through the UV filter used in Figure 6. ~90% of light within the visible spectrum can pass through the filter. (d) Representative sensillum recordings with white light illumination from the indicated genotypes. The right panels confirm the viability of non-responders. (e) Typical TEVC recordings with the indicated TRPA1 isoforms from flies (upper and middle) and mosquitoes (lower). TRPA1(A) mutants that lack nucleophile sensitivity did not respond to white light (middle). Colored boxes indicate the time windows of light illumination.
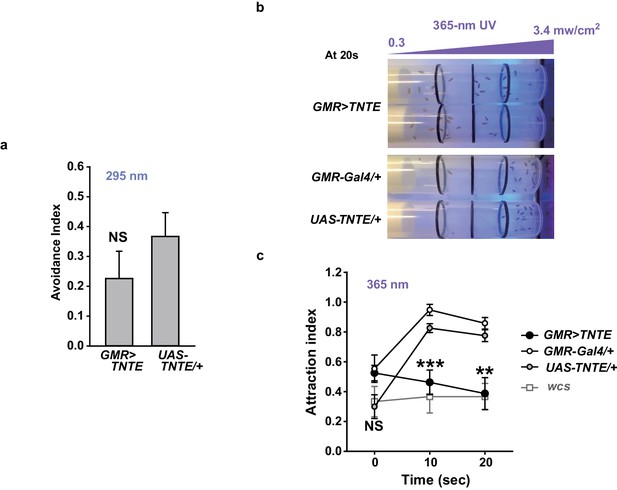
Photoreceptors are important for UVA attraction but not for UVB-dependent feeding suppression.
(a) UVB-illuminated Café assay results with animals in which chemical synaptic transmission of photoreceptors is silenced by the tetanus toxin light chain. NS: not significant. (b) Typical results showing UVA attraction of the indicated genotypes. The purple ramp illustrates the putative UVA gradient between the measured intensities at two internal extremes of the vial. Twenty seconds after illumination control flies mostly resided at the side of the UVA source, while flies with silenced neurons did not. (c) Summary of experiments in (b) and experiments with wcs (grey). ***p<0.001, Tukey’s test.
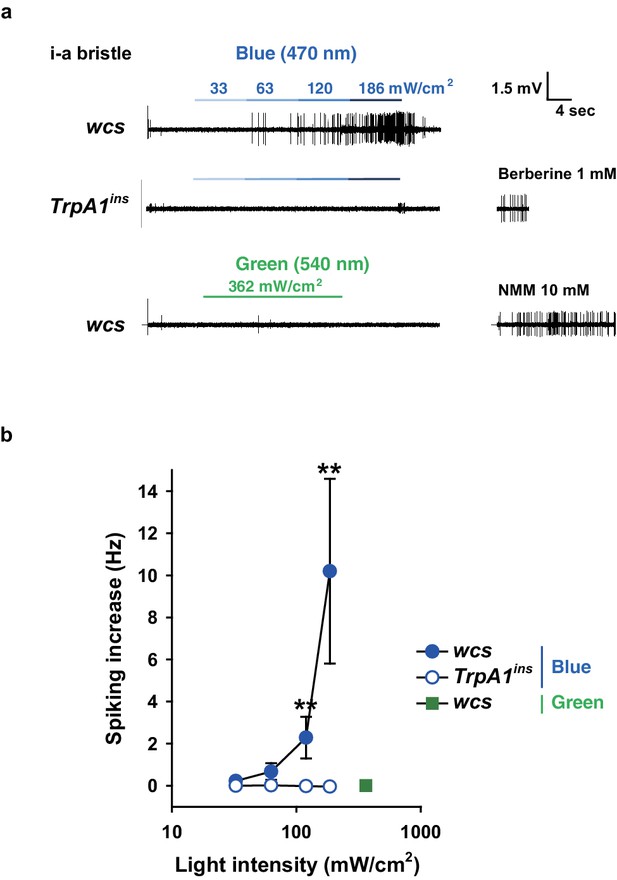
Blue but not green light is capable of activating taste neurons, which depends on TrpA1.
(a) Increasing intensities of blue light at 470 nm were administered as indicated to i-a bristles of WT and TrpA1ins. Green light did not elicit action potentials at a much higher intensity (bottom). (b) Averaged data (n = 5–8). **p<0.01, ***p<0.001, Mann-Whitney test between WT and TrpA1ins.
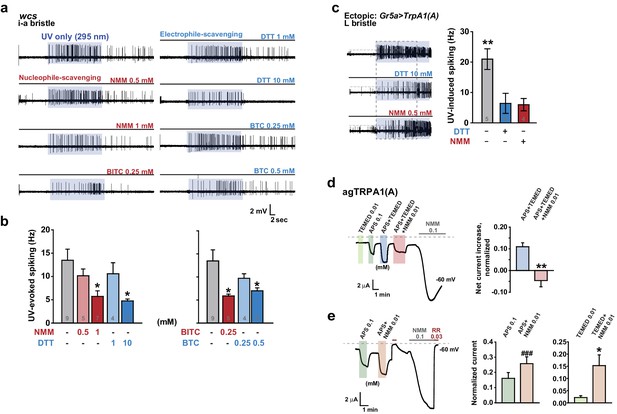
Nucleophilicity is required for UV or free radical-evoked TRPA1(A) activation.
(a and b) Nucleophile-scavenging electrophiles or electrophile-scavenging nucleophiles suppress UV responses in i-a bristles. Electrophiles are in red and nucleophiles are in blue. Typical results are presented in (a), with mean and SEM values provided in (b) as bar graphs. *p<0.05, ANOVA Dunn’s test. The numbers of conducted experiments are given at the bottom of each bar. (c) Ectopically expressed TRPA1(A) in sugar-sensing cells of L-bristles shows a reduced UV response in the presence of DTT or NMM. The dashed box indicates the data set that is presented in the right panel as bar graphs. **p<0.01, Tukey’s test. (d) Chemically generated free radicals activate agTRPA1(A) in oocytes when ammonium persulfate (APS) and tetramethylethylenediamine (TEMED) were incubated for >30 min (see also Figure 7—figure supplement 1). Activation is abrogated by incubation with the nucleophile scavenger NMM. Left: a representative recording. Right: the averaged net effects of the APS/TEMED mixture on agTRPA1(A) activation with or without NMM. **p<0.01, Student’s t-test (n = 4 and 6 and 6). (e) NMM does not act as an antagonist of heterologous agTRPA1(A) with either APS or TEMED alone. Left: a typical result with APS. Middle and Right: summary of APS (n = 5) and TEMED (n = 4–5) experiments, respectively. ###: p<0.001, paired t-test. *p<0.05, Student’s t-test.
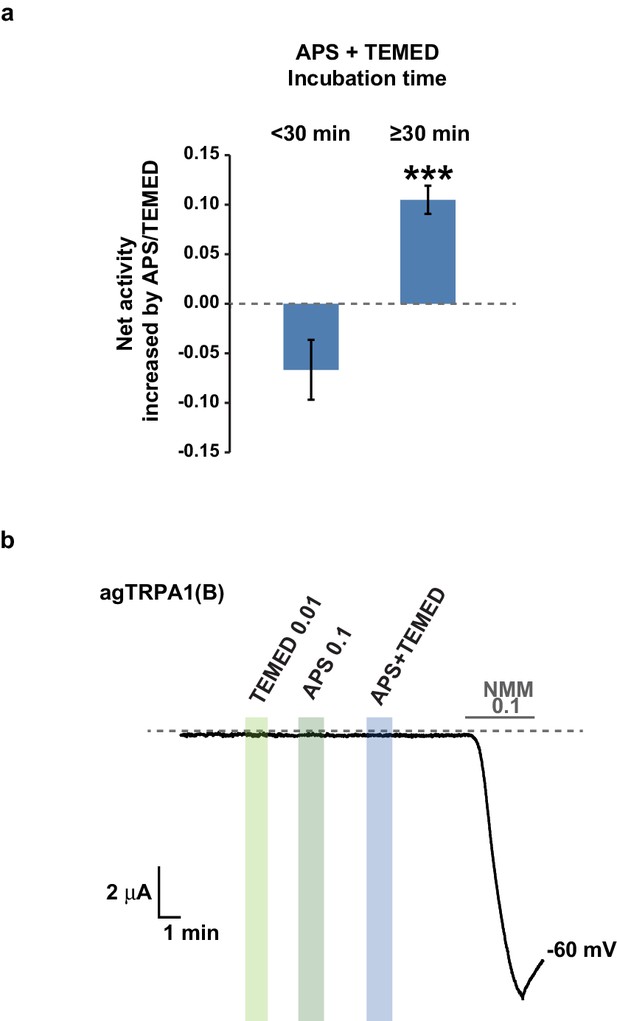
TRPA1(A)-specific activation by the APS+TEMED mixture is time-dependent.
(a) The net current increases resulting from the APS and TEMED mixture (0.1 and 0.01 mM, respectively) are binned into two groups based on incubation time as indicated and compared for their efficacy in activating agTRPA1(A) expressed in frog oocytes (n = 6 and 10 and 10). ***p<0.001, Student’s t-test. (b) agTRPA1(B) did not respond to the indicated chemicals, while it generated large NMM current in oocytes.
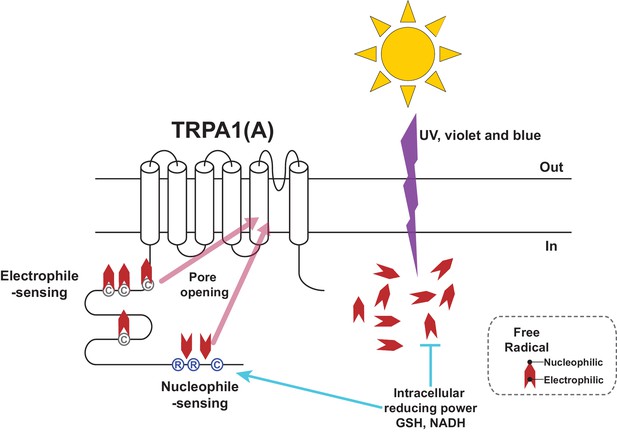
Schematic illustration of the TRPA1(A) activation mechanism in response to solar UV irradiation.
Solar irradiation inflict both electrophilicity and nucleophilicity in the cytosol. The two opposing characteristics of radicals were sensed by two distinct domains of TRPA1(A). Electrophilicity is often neutralized by the cytosolic reducing power, but nucleophilicity is not interfered with. C and R in the circles respectively represent cysteine and arginine critical for each activation pathway. Chevrons depict amphiphilicity of free radicals with two opposing ends.
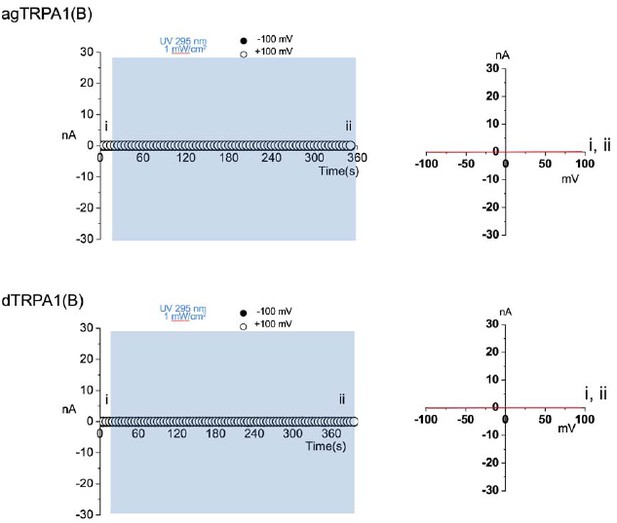
Membrane macropatches from cells expressing TRPA1(B) isoforms fail to show current responses to prolonged UV illumination.
https://doi.org/10.7554/eLife.18425.027Additional files
-
Supplementary file 1
Response profile of TRPA1s to light and chemical agonists in Xenopus oocytes.
- https://doi.org/10.7554/eLife.18425.026