Substrate specificity of TOR complex 2 is determined by a ubiquitin-fold domain of the Sin1 subunit
Figures
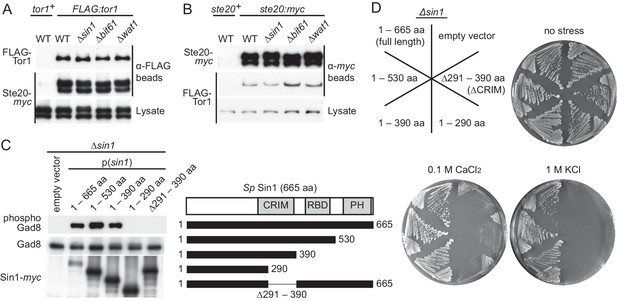
CRIM, but not the RBD and PH domain, is essential for Sin1 function.
(A, B) The RICTOR ortholog Ste20 associates with Tor1 in the absence of Sin1. (A) Co-purification of Ste20-myc with FLAG-Tor1 was tested by anti-FLAG (‘α-FLAG’) affinity beads in wild-type (CA7087), ∆sin1 (CA7143), ∆bit61 (CA7150) and ∆wat1 (CA7151) cell lysate. A ste20:myc strain expressing untagged Tor1 (CA6435) was used as a negative control (tor1+). (B) Co-purification of FLAG-Tor1 with Ste20-myc was tested by anti-myc (‘α-myc’) affinity beads as in (A). A FLAG:tor1 strain expressing untagged Ste20 (CA6530) was used as a negative control (ste20+). (C) The CRIM, but not the RBD and C-terminal PH domain, of Sin1 is required for TORC2 to phosphorylate Gad8. TORC2-dependent phosphorylation of Gad8-S546 and total Gad8 were detected by immunoblotting (Tatebe et al., 2010) in cell lysate from a ∆sin1 strain (CA5126) carrying plasmids to express full-length (1–665 aa) or various Sin1 fragments fused to the myc epitope. (D) Expression of the mutant Sin1 lacking the C-terminus rescues the ∆sin1 phenotype. Their stress sensitivity was evaluated by growth on solid YES medium containing either 1 M KCl or 0.1 M CaCl2 for 2 days at 30°C. CRIM, Conserved region in the middle; PH, Pleckstrin homology; RBD, Ras-binding domain.
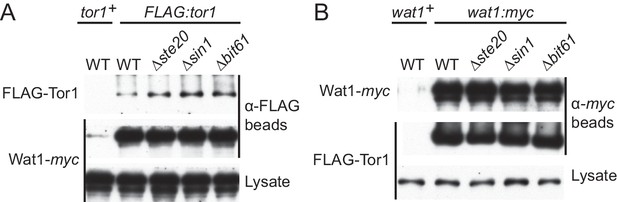
Sin1 is dispensable for the interaction between Tor1 and the LST8 ortholog Wat1.
(A) Five fission yeast strains (CA7183, CA7213, CA7286, CA7317, CA7329) were grown to mid log phase, and the cell lysate was subjected to immunoprecipitation with anti-FLAG-affinity gel, followed by immunoblotting with anti-myc and anti-FLAG antibodies. (B) Five fission yeast strains (CA6530, CA7213, CA7286, CA7317 and CA7329) were tested as in (A) with anti-myc-affinity gel.
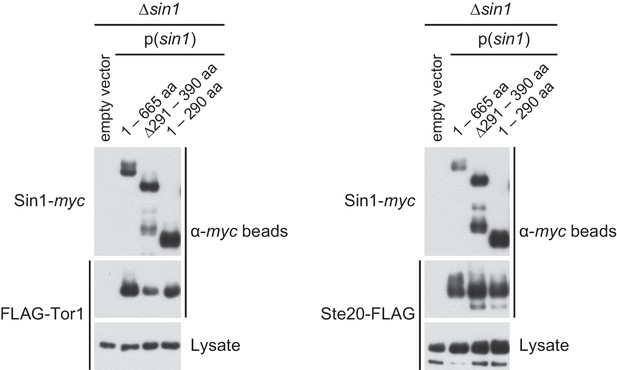
The CRIM domain is not required for Sin1 to bind the other TORC2 subunits.
∆sin1 strains expressing FLAG-tagged Tor1 (CA6870) or Ste20 (CA6275) were transformed with an empty vector, or a plasmid that expresses the myc epitope-tagged full-length Sin1 (1–665 aa) or Sin1 fragments lacking CRIM (∆291–390 aa, 1–290 aa; Figure 1C). Sin1-myc was precipitated with anti-myc-affinity beads and co-purification of FLAG-Tor1 and Ste20-FLAG was examined by anti-FLAG immunoblotting. CRIM, Conserved region in the middle.
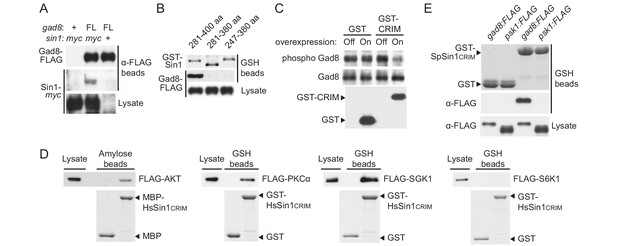
Sin1CRIM binds specifically to the TORC2 target AGC kinases.
(A) Sin1 interacts with the TORC2 substrate Gad8 in S. pombe. Co-purification of Sin1-myc with Gad8-FLAG (‘FL’) precipitated by anti-FLAG beads was tested in a sin1:myc gad8:FLAG strain (lane 2; CA6993). sin1:myc gad8+ (lane 1; CA6984) and gad8:FLAG sin1+ (lane 3; CA6281) strains were used as negative controls. (B) SpSin1CRIM can bind Gad8 in S. pombe. SpSin1CRIM fused to GST was expressed in a gad8:FLAG strain (CA6281), and proteins collected on glutathione (GSH)-beads and the cell lysate were analyzed by Coomassie blue staining and anti-FLAG immunoblotting. (C) Overexpressed SpSin1CRIM inhibits Gad8 phosphorylation by TORC2. GST or GST- SpSin1CRIM (247–400) were induced (‘On’) from the thiamine-repressible nmt1 promoter and the crude cell lysate was analyzed by immunoblotting. (D) Human Sin1CRIM binds mTORC2 substrates, but not the mTORC1 substrate S6K1. FLAG-tagged AKT, PKCα, Sgk1 and S6K1 were expressed in HEK-293T and the cell lysate was incubated with bacterially produced MBP- or GST-fused HsSin1CRIM, which were immobilized onto amylose- and GSH-beads, respectively. Proteins bound to the beads and the cell lysate were analyzed by anti-FLAG immunoblotting and Coomassie blue staining. (E) SpSin1CRIM does not interact with Psk1, a S. pombe TORC1 substrate. Bacterially produced GST-SpSin1CRIM bound to GSH-beads was incubated with cell lysate from gad8:FLAG (CA6281) and psk1:FLAG (CA8070) strains of S. pombe. Proteins bound to the GSH-beads and the cell lysate were analyzed by anti-FLAG immunoblotting and Coomassie blue staining.
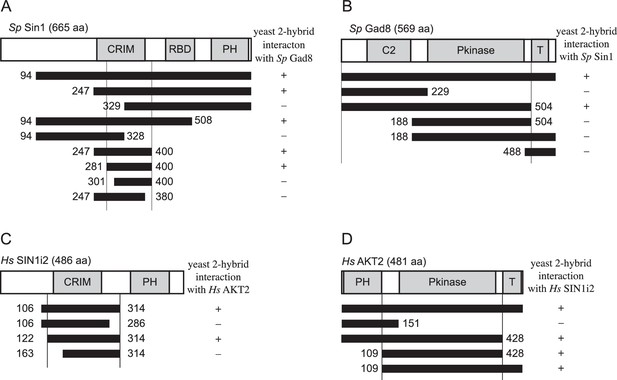
Interaction between fission yeast Sin1 – Gad8 and human Sin1 – AKT2 in yeast two-hybrid assays.
(A) Interaction between various fission yeast Sin1 fragments and Gad8 in yeast two-hybrid assays. Abbreviation: CRIM, Conserved Region In the Middle; RBD, Raf-like Ras-binding domain; PH, pleckstrin homology. CRIM spans the amino acid residues 253–383 of fission yeast Sin1 (Schroder et al., 2004); RBD and PH do the residues 438–514 and 560–658, respectively (Schroder et al., 2007). Interaction was assessed by histidine auxotrophy: ‘+’, interaction; '–', no interaction. (B) Interaction of Gad8 fragments with Sin1 in yeast two-hybrid assays. Abbreviations: C2, C2 domain; Pkinase, protein kinase domain; T, protein kinase C-terminal domain. Domain prediction is based on the motifs PF00069, PF00433, and PF00168 in the Pfam database. (C) Interaction between various human Sin1 central fragments and human AKT2 in yeast two-hybrid assays. CRIM spans the amino acid residues 137–267 of human Sin1i2 (Schroder et al., 2004), while PH does the amino acid residues 348–450 (Schroder et al., 2007). Note that the Sin1i2 isoform does not contain the intact RBD. (D) Interaction of human AKT2 fragments with human SIN1 in yeast two-hybrid assays. Abbreviations: PH, PH domain; Pkinase, protein kinase domain; T, protein kinase C terminal domain. Domain prediction is based on PF00069, PF00433, and PF00169 in the Pfam database.
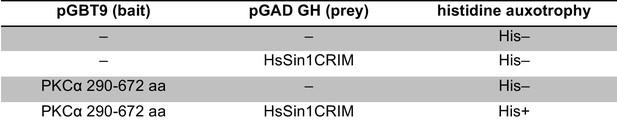
Yeast two-hybrid assay for interaction between HsSin1CRIM and PKCα.
Interaction was examined by HIS3 reporter gene expression in the histidine auxotrophic HF7c budding yeast strain.
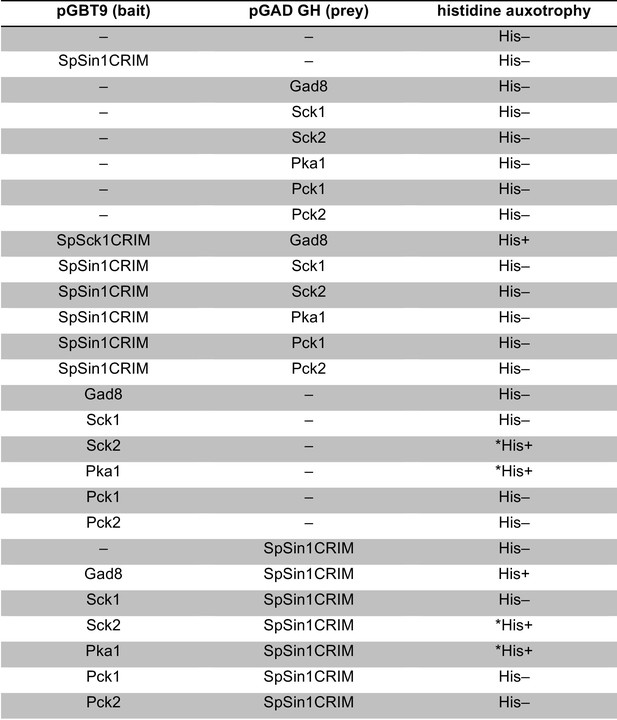
Yeast two-hybrid assay for interaction between SpSin1CRIM and fission yeast Sck1, Sck2, Psk1, Pck1, Pck2.
Interaction was examined by HIS3 reporter gene expression in the histidine auxotrophic HF7c budding yeast strain. The Sck2 and Pka1 baits exhibited auto-activation in HIS3 reporter gene expression independently of the SpSin1CRIM prey (labeled by asterisks).
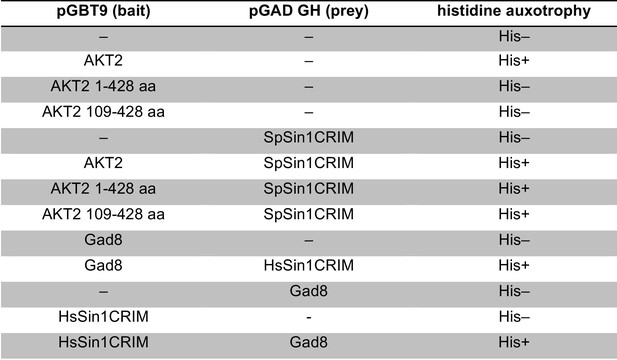
Yeast two-hybrid assay for cross-species interaction between Sin1, Gad8, and AKT2.
Interaction was examined by HIS3 reporter gene expression in the histidine auxotrophic HF7c budding yeast strain.
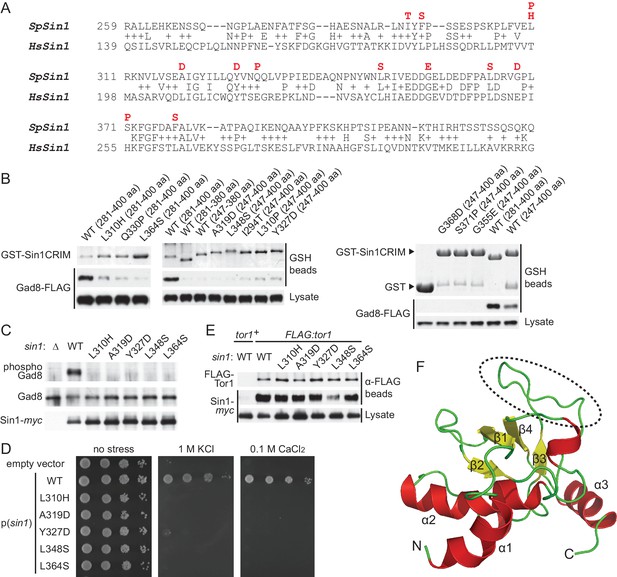
Sin1CRIM forms a ubiquitin-fold domain that binds TORC2 substrates.
(A) Yeast two-hybrid screens isolated SpSin1CRIM mutations that abrogate the interaction with Gad8 (red). Pairwise alignment of the fission yeast and human CRIM sequences was performed using the SSEARCH program (Pearson, 1991). (B) Defective interaction of the mutant SpSin1CRIM with Gad8. The Sin1CRIM (residues 247–400 or 281–400) fragments and those with the indicated mutations were expressed as GST-fusion in a gad8:FLAG strain of S. pombe (CA6281), and proteins collected on GSH-beads and the cell lysate were detected by Coomassie blue staining and anti-FLAG immunoblotting. (C) TORC2 with the Sin1CRIM mutations fails to phosphorylate Gad8-S546. A ∆sin1 strain (CA5126) was transformed with a plasmid to express myc-tagged wild-type or mutant Sin1 carrying one of the CRIM mutations, and the cell lysate was analyzed by immunoblotting. (D) Stress sensitivity of the Sin1 mutants in (C) was examined by growth on YES agar medium containing either 1 M KCl or 0.1 M CaCl2. (E) CRIM mutations do not compromise the incorporation of Sin1 into TORC2. A ∆sin1 FLAG:tor1 strain (CA6870) was transformed with the plasmids used in (C), and co-purification of the wild-type and mutant Sin1-myc with FLAG-Tor1 precipitated by anti-FLAG beads was tested. A ∆sin1 strain expressing untagged Tor1 (CA5126) was used as a negative control (tor1+). (F) A ribbon representation of the lowest energy structure of SpSin1CRIM (272–397). The protruding acidic loop region is marked by a dashed oval.
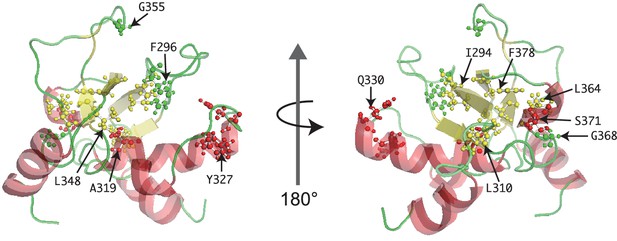
Mutated residues of SpSin1CRIM in the tertiary structure.
The Sin1 residues whose mutation abrogates the interaction with Gad8 (Figure 3A) are shown as ball-and-stick models in the NMR structure of the CRIM domain.
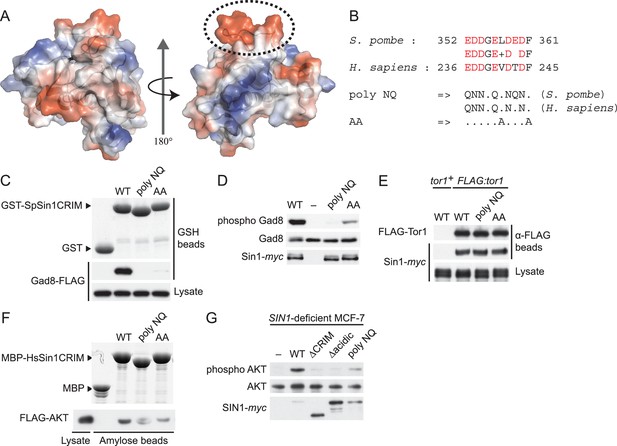
The protruding acidic loop of CRIM is essential for Sin1 to interact with TORC2 target kinases.
(A) The molecular surface of SpSin1CRIM colored according to the electrostatic potential ranging from positive (blue) to negative (red) charge. The protruding acidic loop is marked by a dashed oval. (B) Alignment of the fission yeast and human Sin1CRIM sequences that correspond to the acidic protrusion. The ‘poly NQ’ and ‘AA’ show the amino acid substitutions used in (C) ~ (G). (C) The acidic and hydrophobic residues in the protruding loop of SpSin1CRIM are essential for Gad8 binding in vitro. The recombinant wild-type and mutant GST-SpSin1CRIM on GSH-beads were incubated with cell lysate from a gad8:FLAG strain (CA6281). Proteins bound to the beads and the cell lysate were analyzed by anti-FLAG immunoblotting and Coomassie blue staining. (D) TORC2 with the mutations to the acidic loop of Sin1CRIM fails to phosphorylate Gad8. A ∆sin1 strain (CA5126) was transformed with a plasmid to express myc-tagged wild-type or mutant Sin1 carrying the poly-NQ and AA mutations, and the cell lysate was analyzed by immunoblotting. (E) The acidic loop mutations do not compromise the incorporation of Sin1 into TORC2. A ∆sin1 FLAG:tor1 strain (CA6870) was transformed with the plasmids used in (D), and co-purification of the wild-type and mutant Sin1-myc with FLAG-Tor1 precipitated by anti-FLAG beads was tested. A ∆sin1 strain expressing untagged Tor1 (CA5126) was used as a negative control (tor1+). (F) The acidic and hydrophobic residues in the conserved loop region of HsSin1CRIM are important to bind AKT. FLAG-tagged AKT was expressed in HEK-293T, and the cell lysate was incubated with the recombinant wild-type and mutant MBP-HsSin1CRIM on amylose-beads. Proteins bound to the beads and the cell lysate were analyzed as in (C). (G) The conserved acidic loop region of HsSin1CRIM is important for mTORC2-dependent phosphorylation of AKT-S473. The wild-type and mutant SIN1 lacking the entire CRIM (‘∆CRIM’) or acidic region ('∆acidic’) as well as the poly NQ SIN1mutant were expressed with the myc tag in the SIN1-deficient MCF-7 cells. Insulin-stimulated phosphorylation of AKT-S473 was analyzed by immunoblotting.
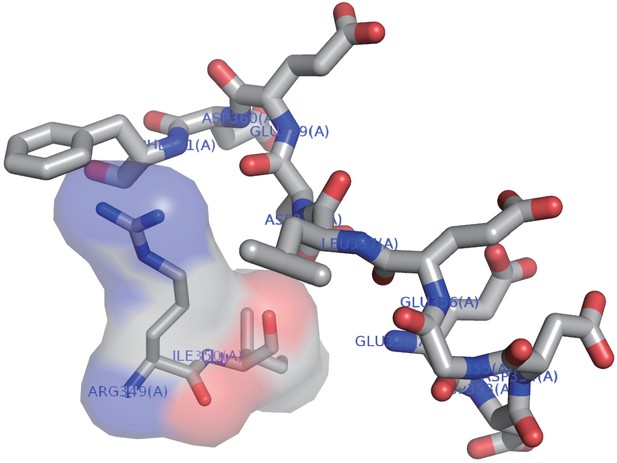
The acidic side chains of the conserved loop region do not interact with other residues of the CRIM domain.
The NMR structure of the acidic loop in SpSin1CRIM (residues 352–361; Figure 4B) is shown as a stick model. The side chains of the most residues within the acidic loop region face outward and do not interact with other residues of the CRIM domain. The Ligplot+ (Laskowski and Swindells, 2011) and WHAT IF (Vriend, 1990) programs identified neither hydrogen bond nor salt bridge between the loop region and the rest of the CRIM domain. Arg349 and Ile350 are predicted by LIGPLOT software to have hydrophobic interaction with the loop region and are shown by a surface model.
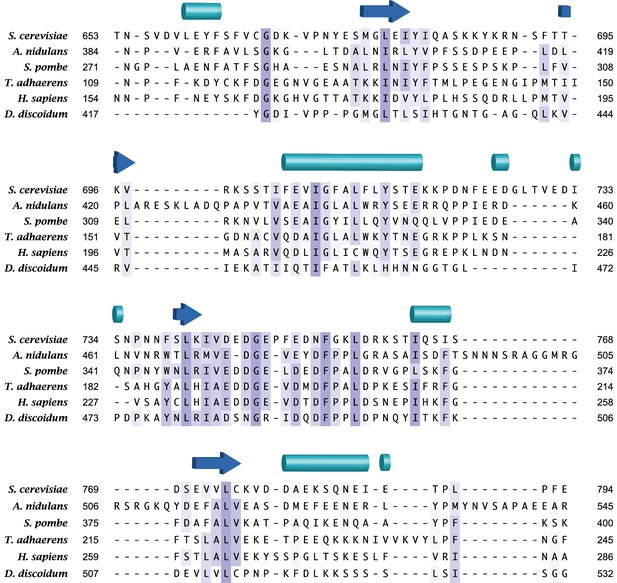
The acidic loop region is highly conserved among Sin1 orthologs.
Multiple alignment was performed by MSAprobWS (Liu et al., 2010) via Jalview (Waterhouse et al., 2009). Sequences included in the alignment are YOL078W (S. cerevisiae), ANID_06304 (A. nidulans), SPAPYUG7.02c (S. pombe), gi52453 (T. adhaerens), NP_077022.1 (H. sapiens) and XP_002649140.1 (D. discoidum). The secondary structure elements of SpSin1CRIM determined by DSSP (Kabsch and Sander, 1983; Touw et al., 2015) are shown above with cylinders and arrows as α-helices and β-sheets, respectively.
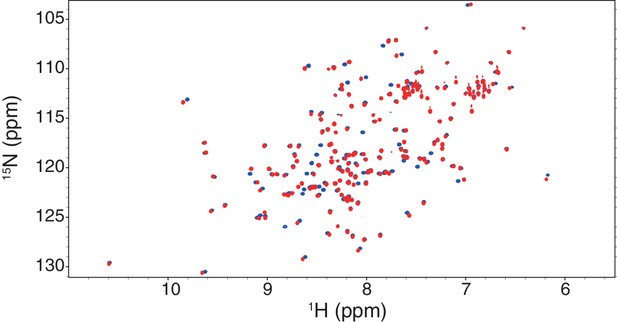
An overlay of 1H-15N HSQC spectra of the wild-type (blue) and ‘poly NQ’ mutant (red) of SpSin1CRIM.
The NMR spectrum of the ‘poly NQ’ mutant resembles that of the wild-type protein, suggesting that the mutation does not significantly disturb the overall folding of the CRIM domain.
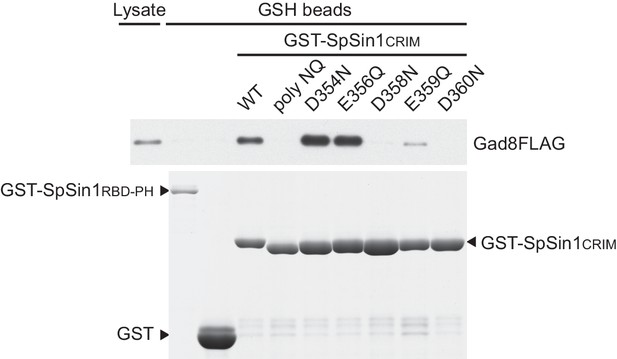
Point mutations to the acidic residues in the conserved SpSin1CRIM loop compromise the interaction with Gad8.
The recombinant wild-type and mutant GST-SpSin1CRIM on GSH-beads were incubated with cell lysate from a gad8:FLAG strain (CA6281). Proteins bound to the beads and the cell lysate were analyzed by anti-FLAG immunoblotting and Coomassie blue staining. Unfused GST and the Sin1 RBD and PH domains (residues 401–665) fused to GST were used as negative controls.
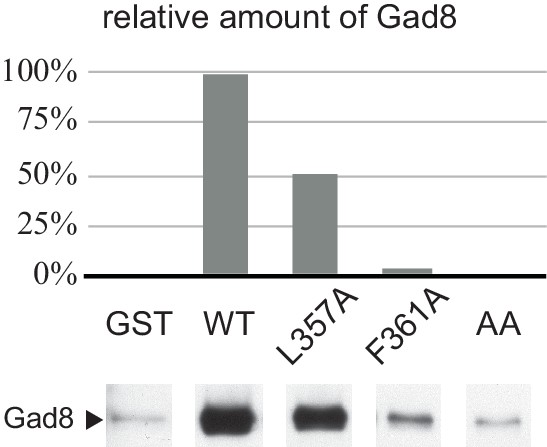
Alanine substitution of the conserved hydrophobic residues within the SpSin1CRIM loop reduces the Gad8 binding.
The recombinant wild-type and mutant GST-SpSin1CRIM on GSH-beads were incubated with the cell lysate from a gad8:FLAG (CA6281) strain. Gad8 bound to the beads were analyzed by anti-FLAG immunoblotting, followed by densitometric quantification.
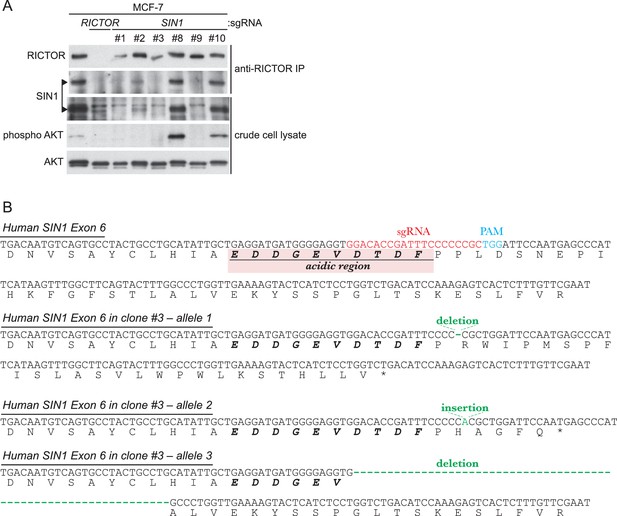
Inactivation of SIN1 by CRISPR/Cas9-mediated genome editing was confirmed by immunoblotting and genomic sequencing.
(A) Expression of the SIN1 protein is abolished in several clones of the MCF-7 human culture cells that are transfected with a SIN1-targeting CRISPR/Cas9 construct. In clones #1, 3, and 9, SIN1 (indicated by arrowheads) was not detected at all by immunoblotting in either crude cell lysate or anti-RICTOR immunoprecipitate. Accordingly, AKT phosphorylation at the hydrophobic motif was completely lost in those clones. Clone #3 was used in the subsequent experiment shown in Figure 4G. (B) Genomic sequencing of SIN1-deficient clone #3 detected a small insertion and small deletions in Exon 6 of human SIN1 that resulted in frameshift mutations or an internal deletion.
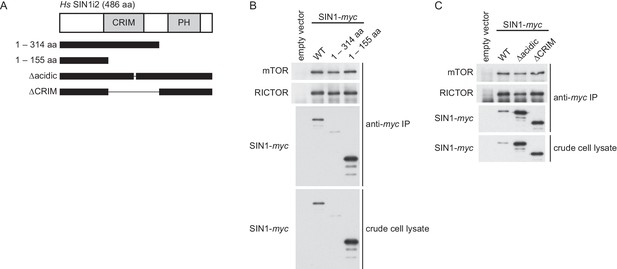
The CRIM domain of human Sin1 is not required for its interaction with mTOR and RICTOR to form mTORC2.
(A) The truncated SIN1 fragments and the SIN1 mutant proteins lacking the conserved acidic loop (∆236–245) or the CRIM domain (∆156–314). (B) The wild-type and mutant SIN1 proteins shown in (A) were expressed with the myc epitope tag in the SIN1-deficient MCF-7 cells. Co-purification of SIN1-myc with mTOR and Rictor was tested by anti-myc immunoprecipitation (IP).
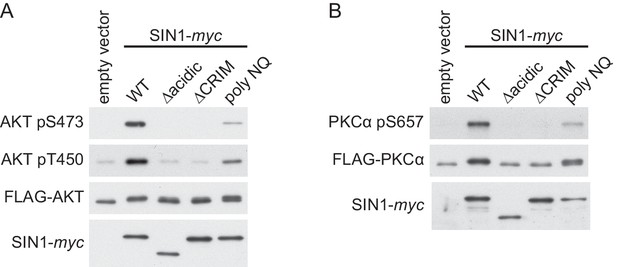
The human Sin1CRIM and its acidic loop are essential for phosphorylation of the mTORC2 targets.
(A) FLAG epitope-tagged AKT was co-expressed with the indicated SIN1 mutants (see Figure 4—figure supplement 7A) in the SIN1-deficient MCF-7 cells. AKT was collected onto anti-FLAG-affinity beads, and immunoblotting was carried out to detect phosphorylation of Ser-473 and Thr-450 in its hydrophobic and turn motifs, respectively. (B) FLAG-tagged PKCα was co-expressed with the indicated SIN1 mutants in the SIN1-deficient MCF-7 cells. PKCα was collected onto anti-FLAG-affinity beads and analyzed by immunoblotting. mTORC2-dependent phosphorylation of PKCα is known to increase its stability (Ikenoue et al., 2008).
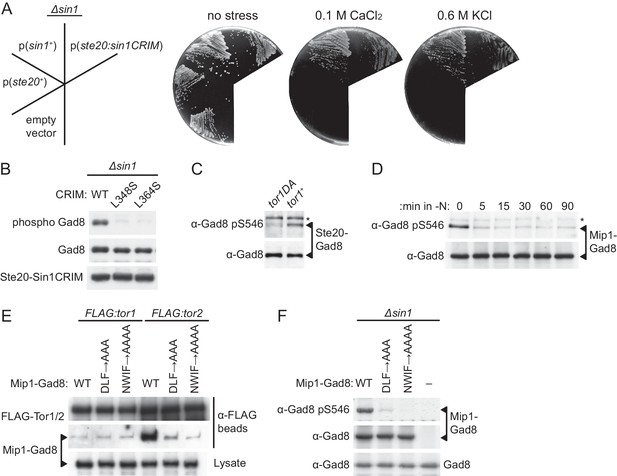
Substrate recruitment by Sin1CRIM determines the specificity of TORC2.
(A and B) SpSin1CRIM fused to the Ste20 subunit can substitute for the Sin1 function in TORC2. In (A), a ∆sin1 strain (CA5126) was transformed with an empty vector, or a plasmid to express the ste20+, sin1+ or ste20:sin1CRIM genes. Growth of the transformants was tested at 30°C on YES plates with and without 0.1 M CaCl2 or 0.6 M KCl. In (B), phosphorylation of Gad8-S546 was examined by immunoblotting in a ∆sin1 strain (CA5126) carrying a plasmid to express FLAG-tagged Ste20 fused to SpSin1CRIM with and without L348S/L364S mutations. (C) TORC2 without Sin1 can phosphorylate Gad8 fused to the Ste20 subunit. ∆sin1 strains with tor1+ (CA7471) and tor1-D2137A (CA7395) alleles were transformed with a plasmid to express Ste20 fused to Gad8. Expression and phosphorylation of the Ste20-Gad8 fusion were detected by antibodies against Gad8 and phosphorylated Gad8-S546, respectively. (D) Gad8 fused to the Mip1 subunit of TORC1 is phosphorylated when TORC1 is active. A ∆sin1 strain (CA5126) carrying a plasmid to express Gad8 fused to Mip1, the Raptor ortholog in fission yeast, was grown to mid-log phase, followed by nitrogen starvation (-N) to inactivate TORC1. Expression and phosphorylation of the Mip1-Gad8 fusion were analyzed as in (C). (E) The Mip1-Gad8 fusion is incorporated into TORC1. Mip1-Gad8 was expressed in FLAG:tor1 (CA6530) and FLAG:tor2 (CA6904) strains, from which TORC2 and TORC1 were collected onto anti-FLAG beads, respectively, and co-purification of Mip1-Gad8 was examined by anti-Gad8 immunoblotting. Alanine substitutions of Mip1 residues 316–318 (‘DLF’) or 366–369 (‘NWIF’) prevented the fusion protein from being incorporated into TORC1. (F) Phosphorylation of Gad8-S546 in the Mip1-Gad8 fusion protein incorporated into TORC1. A ∆sin1 strain (CA5126) was transformed with an empty vector (−) or plasmids to express Mip1-Gad8 with or without the mutations that abrogate its incorporation into TORC1 as shown in (E). Expression and phosphorylation of the Mip1-Gad8 proteins were analyzed as in (D). The endogenous Gad8 was monitored as a loading control (bottom).
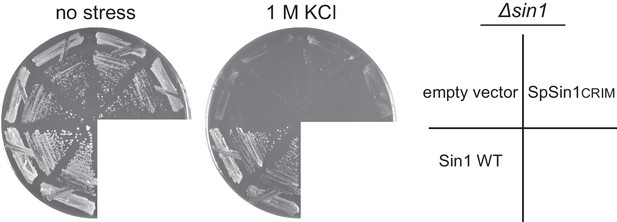
The CRIM domain alone is not sufficient to replace the Sin1 function in vivo.
A ∆sin1 strain (CA5126) was transformed with an empty vector, a plasmid to express the full-length Sin1 (‘Sin1 WT’), or that to express SpSin1CRIM. Growth was tested at 30°C with and without high osmolarity stress of 1 M KCl.
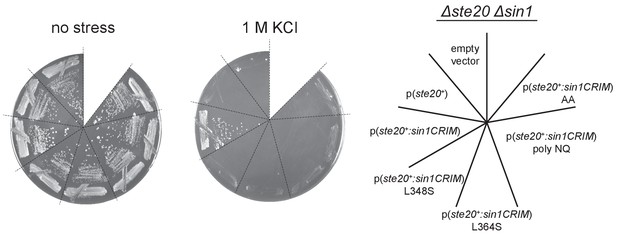
When fused to Ste20, SpSin1CRIM can replace the Sin1 function in a manner dependent on its ability to bind Gad8.
A ∆ste20 ∆sin1 (CA7471) strain was transformed with an empty vector, a plasmid expressing the ste20+ gene, or a plasmid that expresses either wild-type or mutant SpSin1CRIM fused to the Ste20 subunit. Transformants were incubated at 30°C in the absence (‘no stress’) or in the presence of 1 M KCl.
Tables
Structural statistics for SpSin1CRIM. RDC correlation coefficient was caluculated using the program PALES (Zweckstetter and Bax, 2000). Ramachandran analysis was performed using PROCHECK 3.5.4 (Laskowski et al., 1996). Deviations from ideal geometry was caluculated using PDB Validation Server.
NOE upper distance restraints | |
---|---|
Short-range (|i−j|<=1) | 636 |
Medium-range (1<|i−j|<5) | 132 |
Long-range (5<=|i−j|) | 199 |
Total | 967 |
PRE distance restraints | 748 |
Dihedral angle restraints | |
φ | 99 |
ψ | 110 |
χ1 | 12 |
Hydrogen-bond restraints | 0 |
RMS Deviations (272–397) (Å) | |
backbone | 0.89 ± 0.11 |
heavy | 1.36 ± 0.12 |
RDC correlation coefficient | 0.94 ± 0.02 |
Violations | |
NOE (>0.5 Å) | 0 |
PRE (>0.5 Å) | 0 |
Dihedral (>5°) | 0 |
Maximum violation | |
NOE (Å) | 0.49 |
PRE (Å) | 0.15 |
Dihedral (°) | 3.1 |
Ramachandran analysis (272–397) (%) | |
Most favored regions | 91.3 |
Additional allowed regions | 7.7 |
Generously allowed regions | 0.5 |
Disallowed regions | 0.6 |
Deviations from ideal geometry | |
Bond lingths (Å) | 0.011 |
Bond angles (°) | 0.14 |
S. pombe strains used in this study.
Strain ID | Genotype | Source, Reference |
---|---|---|
CA101 | h− leu1–32 | Lab stock |
CA4593 | h− leu1–32 ura4-D18 ∆tor1::ura4+ | |
CA4767 | h− leu1–32 ura4-D18 ∆tor1::ura4+ ∆sin1::kanMX6 | |
CA4855 | h− leu1–32 ura4-D18 ∆tor1::ura4+ ste20:5FLAG(kanR) | This study |
CA5021 | h− leu1–32 ∆ste20::kanMX6 | |
CA5126 | h− leu1–32 ∆sin1::kanMX6 | |
CA5142 | h− leu1–32 ura4-D18 ∆gad8::ura4+ | |
CA5827 | h- leu1–32 ura4-D18 ∆tor1::ura4+ ∆gad8::ura4+ | This study |
CA6275 | h− leu1–32 ste20:5FLAG(kanR) ∆sin1::kanMX6 | This study |
CA6281 | h− leu1–32 gad8:5FLAG(kanR) | |
CA6323 | h− leu1–32 tor1D2137A | This study |
CA6435 | h− leu1–32 ste20:13myc(kanR) | |
CA6530 | h− leu1–32 FLAG:tor1(hph) | |
CA6855 | h− leu1–32 FLAG:tor1(hph) bit61:13myc(kanMX6) | |
CA6859 | h− leu1–32 bit61:13myc(kanMX6) | |
CA6870 | h− leu1–32 FLAG:tor1(hph) ∆sin1::kanMX6 | This study |
CA6904 | h− leu1–32 FLAG:tor2(kanR) | |
CA6984 | h− leu1–32 sin1:13myc(kanMX6) | |
CA6993 | h− leu1–32 sin1:13myc(kanMX6) gad8:5FLAG(kanMX6) | |
CA7087 | h− leu1–32 FLAG:tor1(hph) ste20:13myc(kanMX6) | |
CA7092 | h− leu1–32 FLAG:tor1(hph) sin1:13myc(kanMX6) | |
CA7143 | h− leu1–32 FLAG:tor1(hph) ste20:13myc(kanMX6) ∆sin1::kanMX6 | This study |
CA7147 | h− leu1–32 FLAG:tor1(hph) sin1:13myc(kanMX6) ∆gad8::ura4+ | This study |
CA7150 | h− leu1–32 FLAG:tor1(hph) sin1:13myc(kanMX6) ∆bit61::ura4+ | This study |
CA7151 | h− leu1–32 FLAG:tor1(hph) ste20:13myc(kanMX6) ∆wat1::kanMX6 | This study |
CA7155 | h− leu1–32 FLAG:tor1(hph) ste20:13myc(kanMX6) ∆bit61::ura4+ | This study |
CA7172 | h− leu1–32 FLAG:tor1(hph) ∆sin1::kanMX6 bit61:13myc(kanMX6) | This study |
CA7183 | h− leu1–32 wat1:13myc(kanMX6) | This study |
CA7189 | h− leu1–32 ura4-D18 FLAG:tor1(hph) ste20:13myc(kanMX6) ∆gad8::ura4+ | This study |
CA7200 | h− leu1–32 FLAG:tor1(hph) sin1:13myc(kanMX6) ∆wat1::kanMX6 | This study |
CA7213 | h− leu1–32 FLAG:tor1(hph) wat1:13myc(kanMX6) | |
CA7217 | h− leu1–32 FLAG:tor1(hph) ∆ste20::kanMX6 bit61:13myc(kanMX6) | This study |
CA7222 | h+ leu1–32 ura4-D18 his7–366 FLAG:tor1(hph) ∆ste20::kanMX6 sin1:13myc(kanMX6) | This study |
CA7286 | h− leu1–32 FLAG:tor1(hph) ∆sin1::kanMX6 wat1:13myc(kanMX6) | This study |
CA7307 | h− leu1–32 ura4-D18 FLAG:tor1(hph) bit61:13myc(kanMX6) ∆gad8::ura4+ | This study |
CA7317 | h− leu1–32 ura4-D18 FLAG:tor1(hph) wat1:13myc(kanMX6) ∆bit61::ura4+ | This study |
CA7318 | h− leu1–32 ura4-D18 FLAG:tor1(hph) wat1:13myc(kanMX6) ∆gad8::ura4+ | This study |
CA7319 | h− leu1–32 FLAG:tor1(hph) ∆wat1::kanMX6 bit61:13myc(kanMX6) | This study |
CA7329 | h− leu1–32 ura4-D18 FLAG:tor1(hph) ∆ste20::kanMX6 wat1:13myc(kanMX6) | This study |
CA7395 | h− leu1–32 (hph)FLAG:tor1D2137A(kanMX6) ∆sin1::kanMX6 | This study |
CA7471 | h− leu1–32 ∆ste20::kanMX6 ∆sin1::kanMX6 | This study |
CA8070 | h− leu1–32 psk1:5FLAG(kanMX6) | This study |