An electrostatic selection mechanism controls sequential kinase signaling downstream of the T cell receptor
Figures
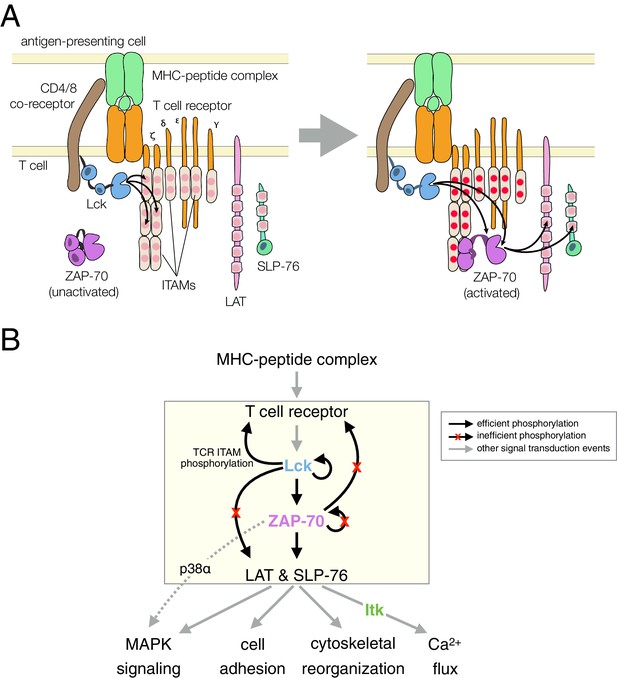
T cell receptor-proximal signaling.
(A) Initiating events in T cell receptor signaling (based on Au-Yeung et al., 2009). (B) Ordered kinase activation and substrate phosphorylation by Lck and ZAP-70 leads to downstream signaling. Abbreviations: TCR, T cell receptor; MHC, major histocompatibility complex; Lck, lymphocyte-specific kinase; ZAP-70, ζ-chain associated protein of 70 kilodaltons; Itk, interleukin-2-inducible T cell kinase; ITAMs, immunoreceptor tyrosine-based activation motifs; LAT, linker for the activation of T cells; SLP-76, SH2-containing leukocyte protein of 76 kilodaltons; and MAPK, mitogen-activated protein kinase.
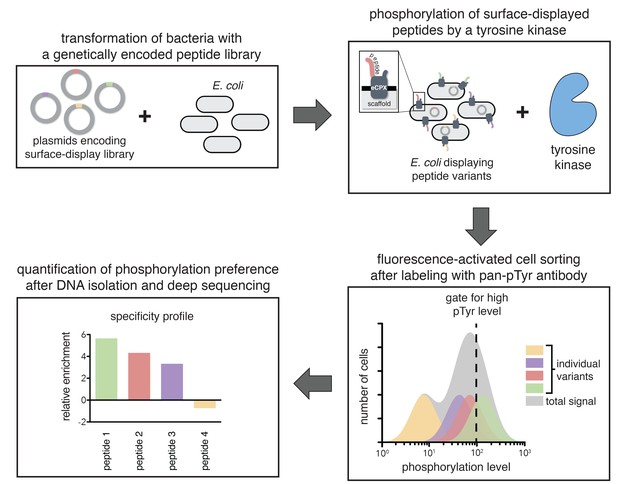
A high-throughput assay for tyrosine kinase specificity.
Top left panel: E. coli cells are transformed with plasmids encoding a library of peptide variants fused to the bacterial surface-display scaffold, eCPX (Rice and Daugherty, 2008). Top right panel: Expression of the peptide-scaffold fusions is induced to permit surface-display of the peptides, then the peptides on the extracellular surface of the cells are phosphorylated by the addition of a tyrosine kinase to the cell suspension (Henriques et al., 2013). Bottom right panel: Phosphorylated cells are labeled with a fluorescent pan-phosphotyrosine antibody, and cells with a high fluorescence signal are isolated by fluorescence-activated cell sorting. Bottom left panel: DNA from the sorted cells and an unsorted control population is isolated and sequenced by Illumina deep sequencing to determine the enrichment of the DNA sequence encoding each variant in the library after selecting for a high phosphorylation level.
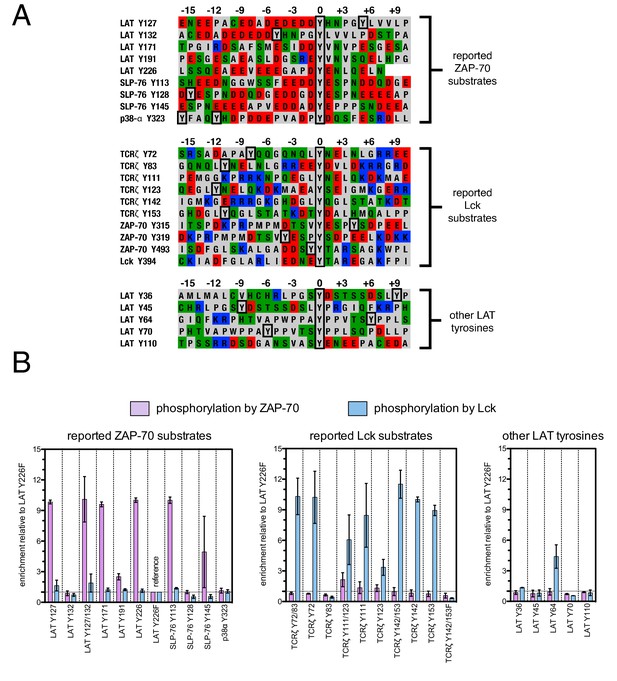
Phosphorylation by ZAP-70 or Lck of a variety of peptides based on LAT, SLP-76, p38α, and TCRζ.
(A) Sequences surrounding the key tyrosine residues analyzed in this study. The focal tyrosine in each sequence is denoted as residue 0, and other proximal tyrosines are highlighted by a black box. (B) Enrichment of peptides from a library of peptide sequences based on LAT, SLP-76, p38α, and TCRζ after phosphorylation by ZAP-70 or Lck, followed by selection. Error bars represent standard deviations in enrichment values from two screens. The exact peptide sequences for each member of the library analyzed in panel B, including the locations of several tyrosine-to-phenylalanine mutants, are given in Figure 3—figure supplement 1.
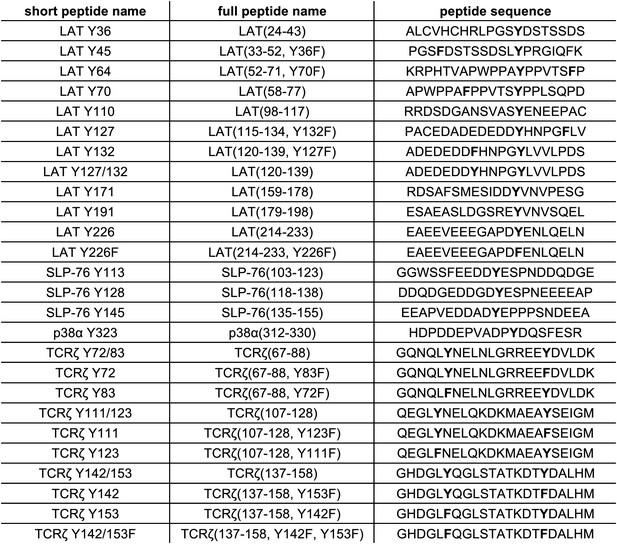
List of peptide sequences in the library containing segments of LAT, SLP-76, p38α, and TCRζ.
Tyrosine residues and tyrosine-to-phenylalanine mutations are shown in bold.
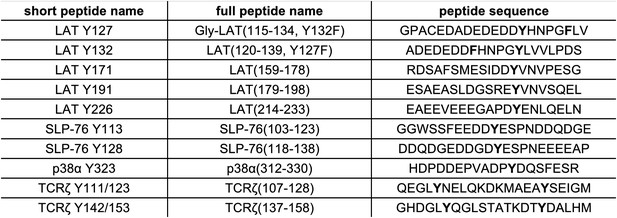
List of purified peptides used for in vitro kinetic assays.
https://doi.org/10.7554/eLife.20105.007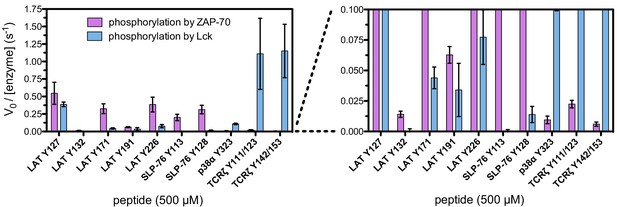
In vitro phosphorylation kinetics of purified peptides by the ZAP-70 and Lck kinase domains.
All peptides were used at a concentration of 500 μM, and both kinases were used at a concentration of 1 μM.
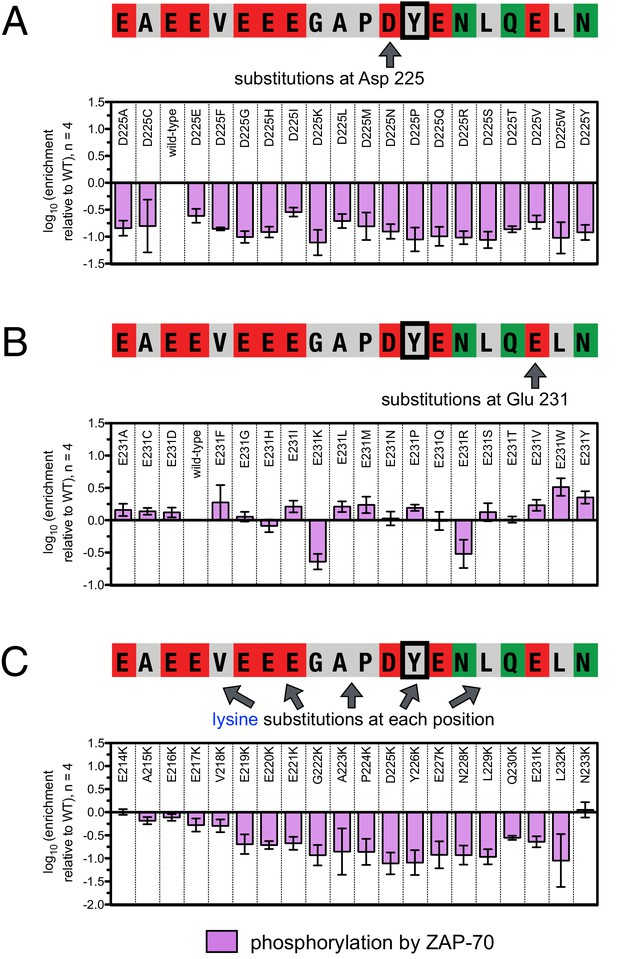
Effect of single amino acid substitutions on the phosphorylation of LAT Tyr 226 by ZAP-70.
(A) Impact of all amino acid substitutions at LAT Asp 225 on the phosphorylation of Tyr 226 by ZAP-70. (B) Impact of all amino acid substitutions at LAT Glu 231 on the phosphorylation of Tyr 226 by ZAP-70. (C) Impact of lysine substitutions at all 20 positions (LAT residues 214–233) on the phosphorylation of Tyr 226 by ZAP-70. Error bars represent standard deviations from an average of four measurements. The enrichment values of each variant were normalized to the enrichment value of the wild-type sequence and presented on a logarithmic scale. Thus, a value of 0 indicates that the substitution did not impact phosphorylation relative to the wild-type sequence, whereas positive and negative values mean that the substitution enhanced or diminished phosphorylation, respectively.
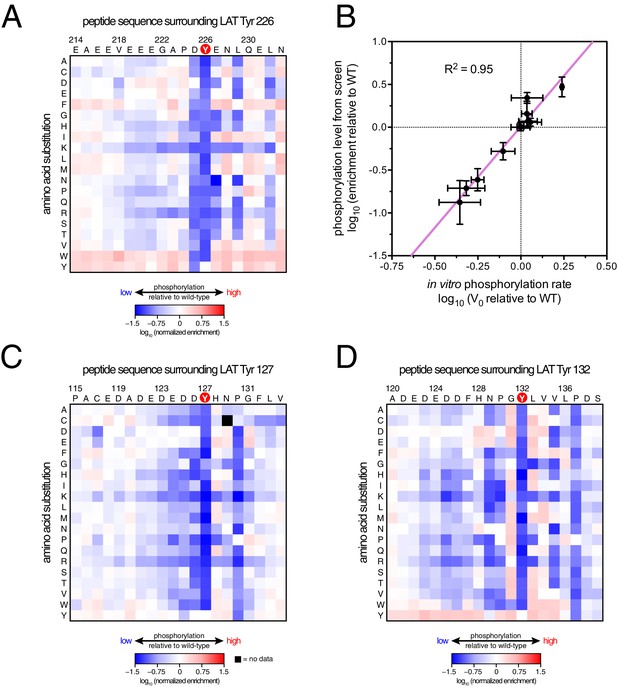
ZAP-70 phosphorylation of LAT point mutant libraries.
(A) Average enrichment values from four independent screens for phosphorylation of the LAT Tyr 226 library by ZAP-70. (B) Correlation between the rates of phosphorylation of 11 purified LAT Tyr 226 variants and their enrichment values from the screen shown in panel A. Horizontal error bars represent the standard deviations from three kinetic measurements with purified peptides, and vertical error bars represent the standard deviations from four screens. (C) Average enrichment values from three independent screens for phosphorylation by ZAP-70 of the LAT Tyr 127 library in a Y132F background. (D) Average enrichment values from three independent screens for phosphorylation by ZAP-70 of the LAT Tyr 132 library in a Y127F background. All enrichment values are log10-transformed and normalized relative to the parent peptide sequence in that screen, which has a value of 0.
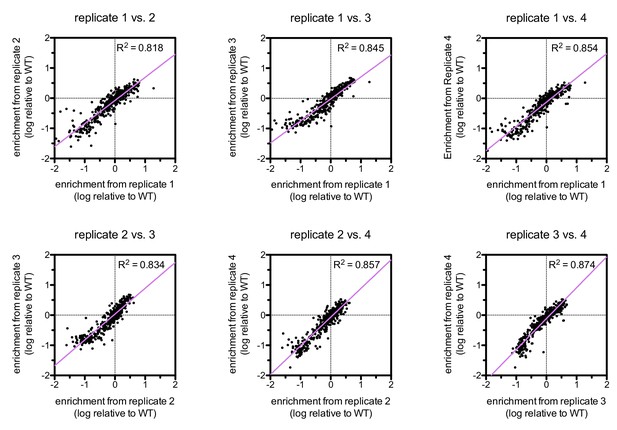
Correlations between enrichments from four replicates for ZAP-70 phosphorylation of the point mutant library spanning LAT Tyr 226.
https://doi.org/10.7554/eLife.20105.011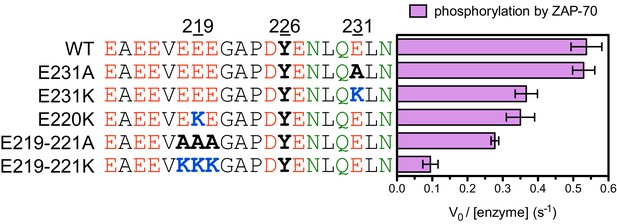
Phosphorylation by the ZAP-70 kinase domain of peptides spanning LAT Tyr 226 with various charge-altering substitutions.
All peptides were used at a concentration of 500 μM, and ZAP-70 was used at a concentration of 1 μM.
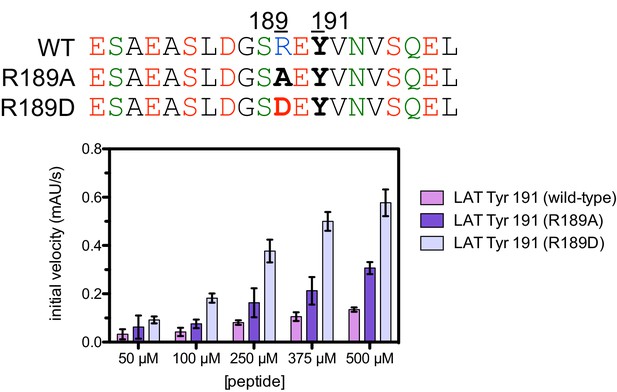
Phosphorylation by the ZAP-70 kinase domain of peptides spanning LAT Tyr 191 with the R189A or R189D substitutions.
ZAP-70 was used at a concentration of 1 μM.
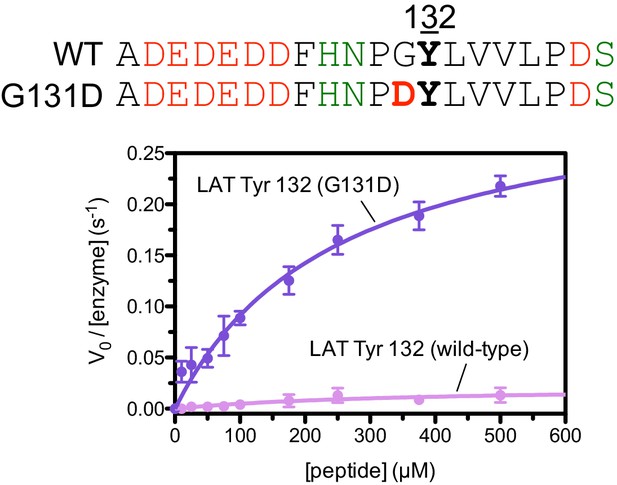
Phosphorylation by the ZAP-70 kinase domain of peptides spanning LAT Tyr 132 with or without the G131D substitution.
ZAP-70 was used at a concentration of 1 μM.
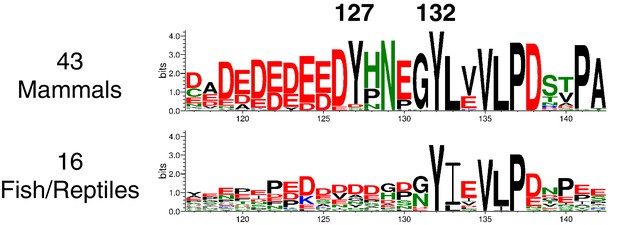
Sequence logos based on an alignment of vertebrate LAT segments surrounding Tyr 132.
Note that Tyr 127 is not conserved outside of mammals, and that the PLCγ SH2-binding motif (Y[I/L]XV) is conserved.
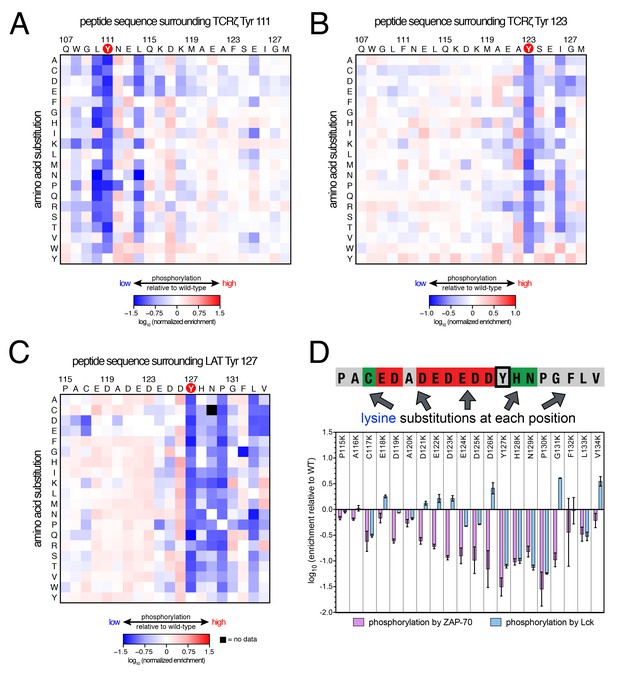
Lck phosphorylation of TCRζ ITAM and LAT point mutant libraries.
(A) Average enrichment values from two independent screens for phosphorylation by Lck of the TCRζ Tyr 111 library in a Y123F background. (B) Average enrichment values from two independent screens for phosphorylation by Lck of the TCRζ Tyr 123 library in a Y111F background. (C) Average enrichment values from two independent screens for phosphorylation by Lck of the LAT Tyr 127 library in a Y132F background. (D) Comparison of the effect of lysine mutations on LAT Tyr 127 phosphorylation by ZAP-70 and Lck. Error bars represent standard deviations in enrichment values from three and two screens with ZAP-70 and Lck, respectively. All enrichment values are log10-transformed and normalized relative to the parent peptide sequence in that screen, which has a value of 0.
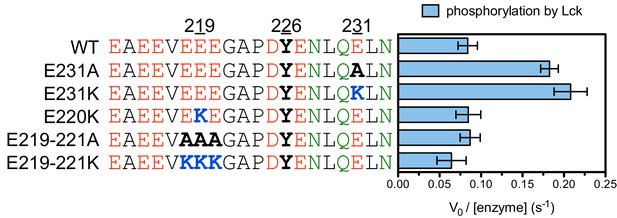
Phosphorylation by the Lck kinase domain of peptides spanning LAT Tyr 226 with various charge-altering substitutions.
All peptides were used at a concentration of 500 μM, and Lck was used at a concentration of 1 μM.
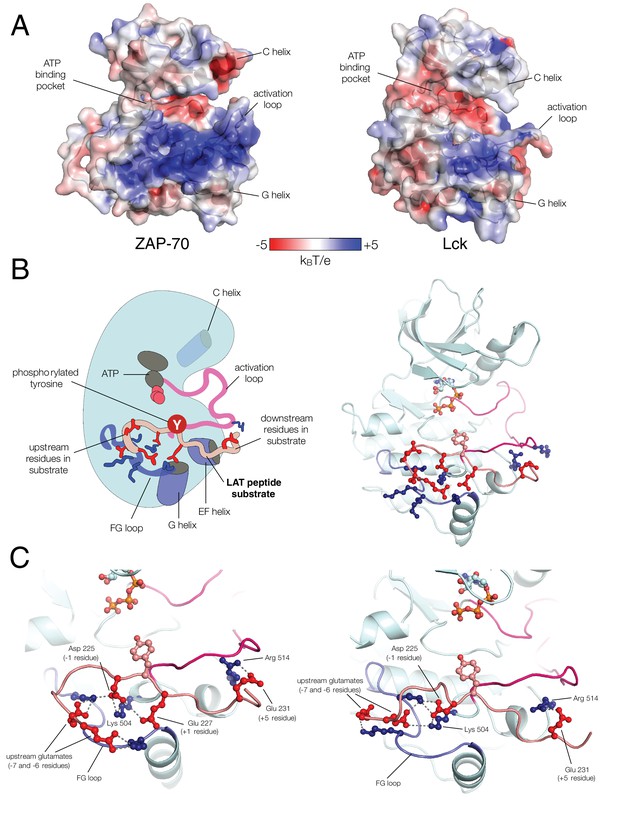
Electrostatic features of the ZAP-70 and Lck kinase domains, and peptide binding modes of ZAP-70.
(A) The electrostatic surface potential, calculated using APBS (Baker et al. 2001) and displayed using PyMOL (Schrödinger 2015), of the ZAP-70 kinase domain (PDB code 1U59) and the Lck kinase domain (PDB code 1QPJ). (B) Schematic representation (left) and an instantaneous structure from a molecular dynamics simulation (right) of the ZAP-70 kinase domain bound to a peptide containing LAT Tyr 226. (C) Two different instantaneous structures from molecular dynamics simulations of ZAP-70 bound to the same peptide, highlighting key kinase-substrate interactions.
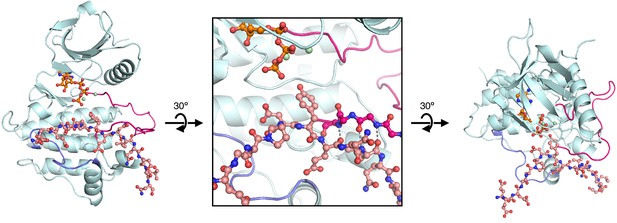
Starting structure used for molecular dynamics simulations of ZAP-70 bound to a peptide spanning LAT Tyr 226.
https://doi.org/10.7554/eLife.20105.019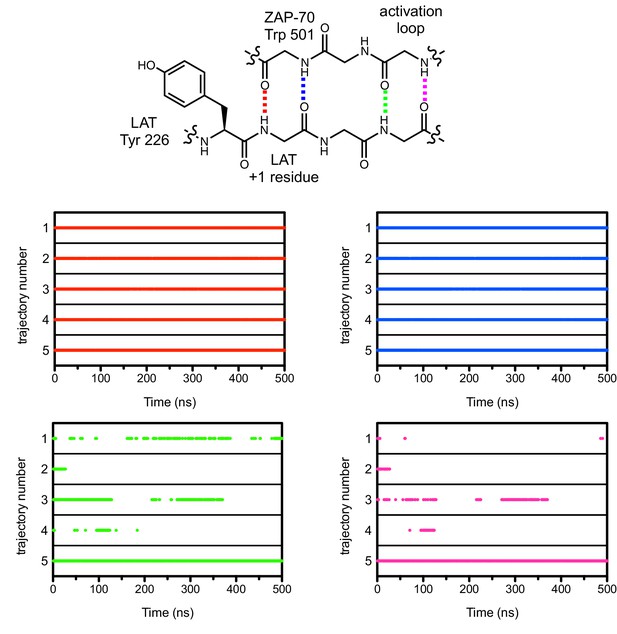
Hydrogen bonds between the ZAP-70 activation loop and the peptide +1 and +3 positions during five molecular dynamics trajectories.
The presence of a hydrogen bond in an instantaneous structure is noted in the graphs with a dot when the hydrogen bond distance was less than 2.5 Å. Side chains in the chemical structure schematic are omitted for clarity.
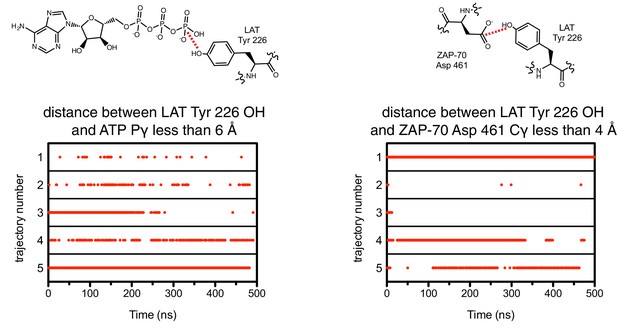
The close proximity between the LAT phospho-acceptor tyrosine and ATP (left) or the catalytic aspartate in ZAP-70 (right) during five molecular dynamics trajectories.
A red dot indicates that, in that particular instantaneous structure, the two atoms connected by a dotted red line in the schematic were less than the distance cutoff noted above each graph.
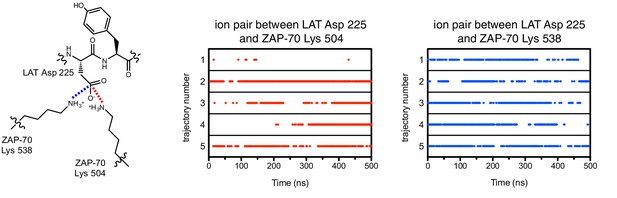
Ion pairs between LAT Asp 225 (−1 position) and lysine residues 504 and 538 on ZAP-70 during five molecular dynamics trajectories.
Red dots indicate that the distance between Asp 225 Cγ and Lys 504 Nε was less than 5 Å in that particular instantaneous structure, and blue dots correspond to the same measurement for Asp 225 and Lys 538.
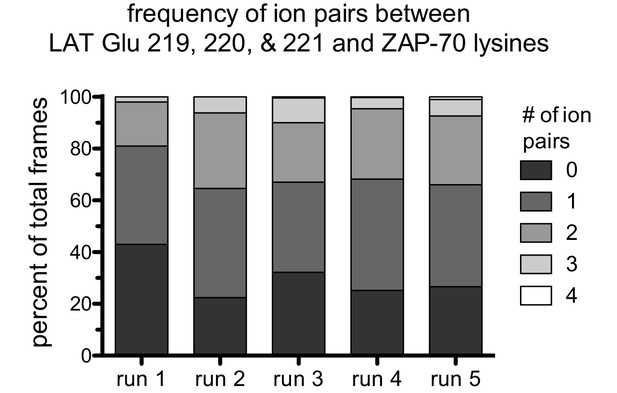
The frequency of ion pairs observed during instantaneous structures sampled every nanosecond from each trajectory.
Ion pairs were defined as existing when the distance between a LAT Glu Cδ atom was less than 4.5 Å from a ZAP-70 Lys Nε atom.
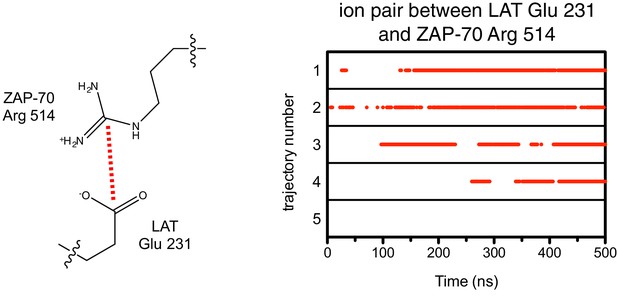
Ion pairs between LAT Glu 231 (+5 position) and Arg 514 on ZAP-70 during five molecular dynamics trajectories.
Red dots indicate that the distance between Glu 231 Cδ and Arg 514 Cζ was less than 5 Å in that particular instantaneous structure.
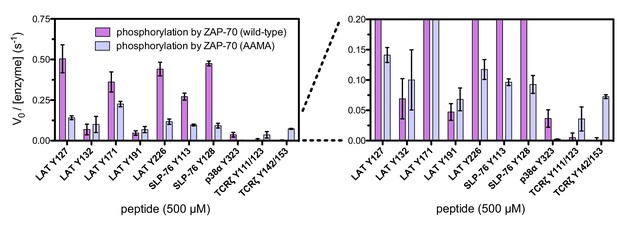
In vitro phosphorylation kinetics of purified peptides by the wild-type ZAP-70 kinase domain and an FG loop mutant containing the K541A, K542A, and K544A substitutions.
All peptides were used at a concentration of 500 μM, and both kinases were used at a concentration of 1 μM.
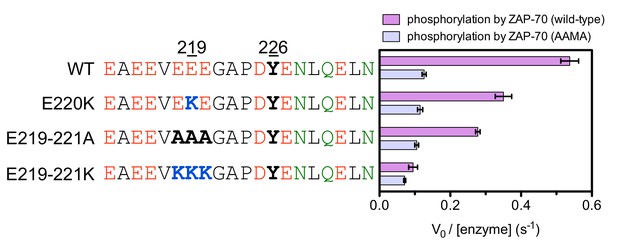
In vitro phosphorylation kinetics of purified peptides surrounding LAT Tyr 226 with various upstream charge-altering mutations by the wild-type ZAP-70 kinase domain and an FG loop mutant containing the K541A, K542A, and K544A substitutions.
All peptides were used at a concentration of 500 μM, and both kinases were used at a concentration of 1 μM.
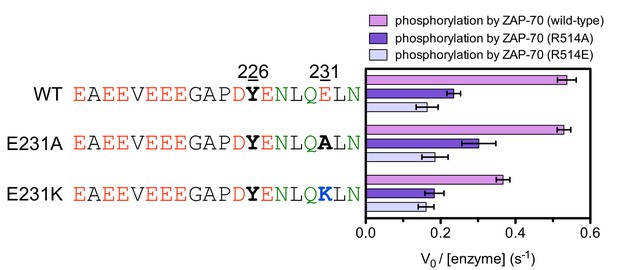
In vitro phosphorylation kinetics of purified peptides surrounding LAT Tyr 226 with the E231A or E231K mutations by the wild-type ZAP-70 kinase domain and mutants containing the R514A or R514E substitutions.
All peptides were used at a concentration of 500 μM, and all three kinases were used at a concentration of 1 μM.
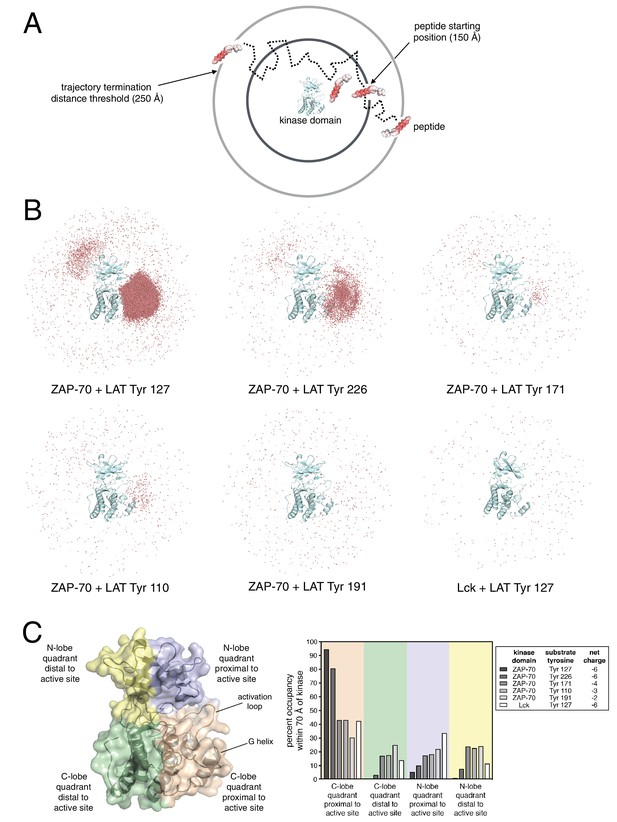
Brownian dynamics simulations of LAT peptide association with ZAP-70 and Lck kinase domains.
(A) Schematic representation of Brownian dynamics trajectories. For each kinase-peptide pair, 10,000 trajectories were initiated by randomly placing the peptide on the surface of a sphere of radius 150 Å, centered on the center of mass of the kinase domain. Trajectories were terminated when the peptide moved more than 250 Å from the center of mass of the protein. (B) Distribution of various LAT Tyr Cα atoms (shown as light red dots) within 70 Å of the ZAP-70 or Lck kinase domain center of mass. For visualization purposes, a random sampling of 0.1% of all peptide positions within the 70 Å sphere in all 10,000 trajectories is displayed. The density of LAT Tyr Cα atoms in each panel reflects the amount of time that peptide spent in the vicinity of the kinase domain. (C) Frequency of substrate tyrosine Cα atoms within four quadrants encompassing the ZAP-70 or Lck kinase domain. Only peptide positions within 70 Å of the center of mass of the kinase domain, as shown in panel B, were considered for this analysis. Quadrants are defined based on atoms in the N-lobe or C-lobe of the kinase, proximal or distal to the active site, as shown in the left panel.
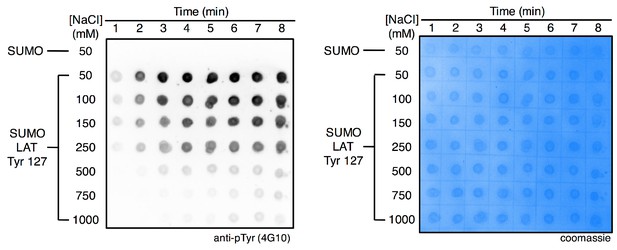
Ionic strength-dependent phosphorylation of LAT Tyr 127 by ZAP-70.
The substrate peptide was fused to SUMO to facilitate blotting, and isolated SUMO was also analyzed as a negative control for phosphorylation (top row). Substrate concentrations were 175 μM, and the ZAP-70 kinase domain was used at a concentration of 500 nM.
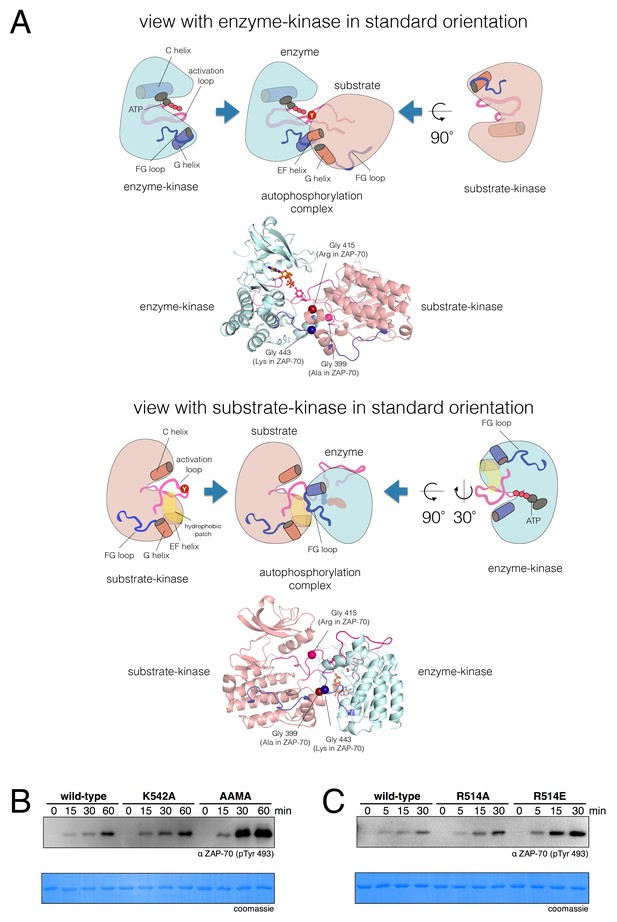
A structural model for activation loop phosphorylation of Lck.
(A) Schematic cartoons and structural renderings of a model for Lck activation loop trans-autophosphorylation. This model is based on a crystal structure of IGF1-R (PDB code 3LVP) (Nemecek et al., 2010), in which one kinase domain molecule in the asymmetric unit, deemed the 'substrate-kinase', presents its activation loop tyrosine into the active site of a second kinase domain molecule in the asymmetric unit, deemed the 'enzyme-kinase'. (B) Western blot analysis of in vitro activation loop autophosphorylation reactions with the wild-type ZAP-70 kinase domain and FG loop mutants bearing the K542A substitution alone or simultaneous K541A, K542A, and K544A substitutions (the 'KKMK' to 'AAMA' mutant). (C) Western blot analysis of in vitro activation loop autophosphorylation reactions with the wild-type ZAP-70 kinase domain and mutants in which Arg 514 was substituted with alanine or glutamate. Kinase domains were used at a concentration of 5 μM in the reactions shown in panels B and C.
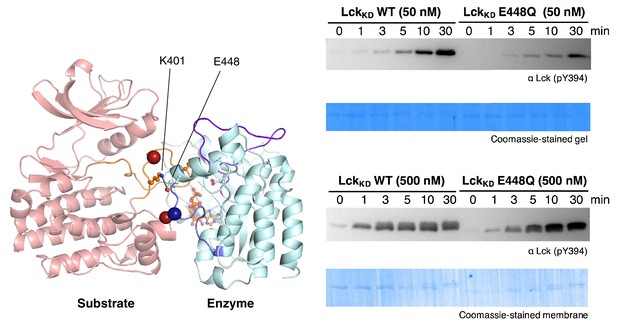
Activation loop autophosphorylation kinetics for the wild-type Lck kinase domain (residues 229–509) and a mutant with the E448Q substitution.
https://doi.org/10.7554/eLife.20105.031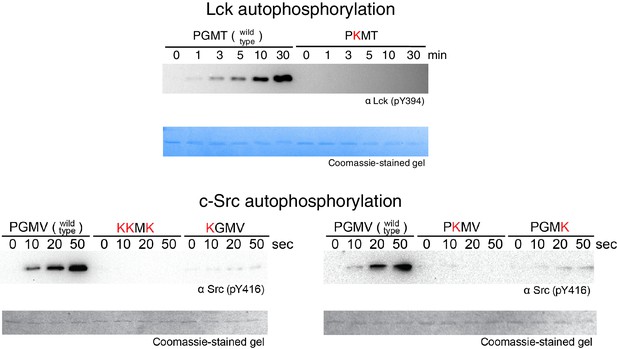
Activation loop autophosphorylation kinetics for the Lck and c-Src kinase domains containing substitutions in the FG loop.
The kinase domains were used at a concentration of 100 nM.
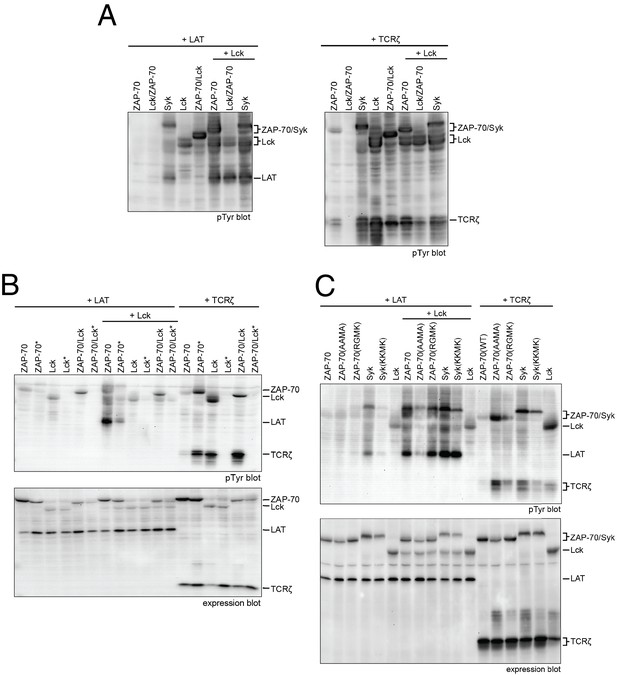
Lck, ZAP-70, and Syk activation and specificity in a model cell line.
(A) Co-expression of wild-type full-length kinases and chimeras of Lck and ZAP-70 with LAT or TCRζ. Two chimeric kinases were used: Lck/ZAP-70 refers to a construct with the Lck SH3-SH2 module fused to the ZAP-70 kinase domain. ZAP-70/Lck refers to the ZAP-70 tandem SH2 module fused to the Lck kinase domain. (B) Comparison of F-G loop mutations of ZAP-70 and Lck. ZAP-70* refers to the KKMK-to-AAMA mutant, and Lck* refers to the PGMT-to-KKMK mutant. (C) Comparison of F-G loop mutations in ZAP-70 and Syk. All experiments were carried out in human embryonic kidney (HEK) 293T cells as previously described (Brdicka et al., 2005), and interpretation of the results is also based on the analysis in Brdicka et al. 2005.
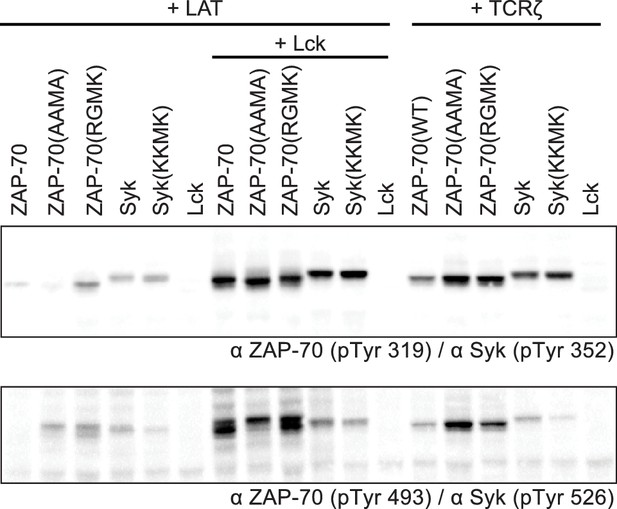
Western blot analysis ZAP-70 and Syk phosphorylation in HEK293 cells with site-specific antibodies.
The top blot shows phosphorylation of a tyrosine in the SH2-kinase linker of ZAP-70 or Syk (Tyr 319 or Tyr 352, respectively). The bottom blot shows phosphorylation of a tyrosine in the kinase domain activation loop of ZAP-70 or Syk (Tyr 493 or Tyr 526, respectively).
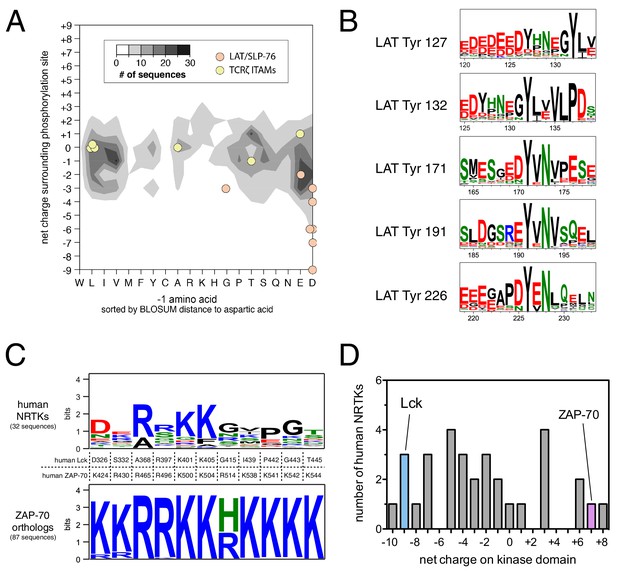
Unique sequence features of ZAP-70 and its substrates.
(A) Analysis of net charge and −1 residue distributions for 1100 tyrosine kinase substrates from the PhosphoSite Plus database (Hornbeck et al., 2015). Analyzed sequences span seven residues on either side of the phospho-acceptor tyrosine. Data are represented as a contour plot, which depicts the prevalence of phosphosites in the database with particular combinations of −1 residue identity and net charge. Darker regions of the contour plot indicate that more sequences have that particular combination of properties. (B) Sequence logos showing the conservation around five LAT tyrosines in 59 species from fish to mammals. (C) Conservation of lysine and arginine residues important for ZAP-70 substrate recognition. The top panel displays a sequence logo showing a lack of conservation at many of these positions in human non-receptor tyrosine kinases (NRTKs). The bottom panel displays high conservation at these positions between ZAP-70 orthologs from fish to mammals. (D) Distribution of net charge for the kinase domains of all 32 human non-receptor tyrosine kinases.
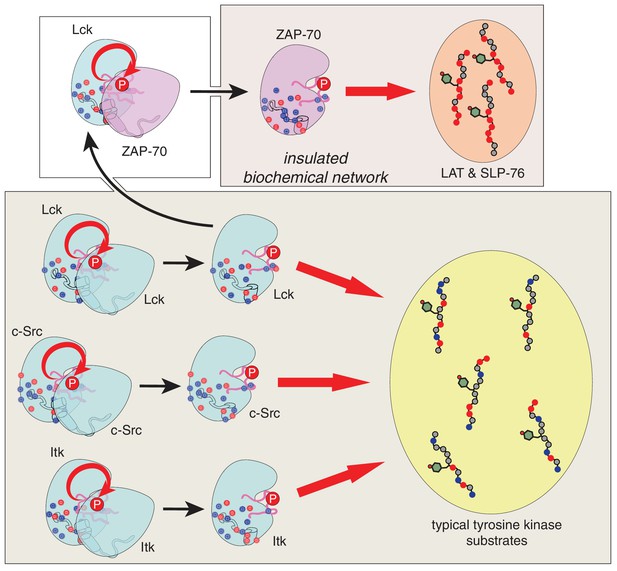
Schematic depiction of the biochemical insulation of ZAP-70, LAT, and SLP-76.
Many tyrosine kinases, such as Lck, c-Src, and Itk, can activate themselves through trans-autophosphorylation of their activation loops (bottom panel, left side). These kinases can efficiently phosphorylate typical tyrosine kinase substrates, which have a near-neutral net charge (bottom panel, right side). ZAP-70 activation requires phosphorylation of its activation loop by Lck (top left panel). Once activated, ZAP-70 exclusively phosphorylates LAT and SLP-76, but not typical tyrosine kinase substrates, and LAT and SLP-76 cannot be phosphorylated by kinases like Lck, c-Src, and Itk (top right panel). Thus, ZAP-70 and its substrates are insulated from other proteins in the T cell receptor signaling pathway.
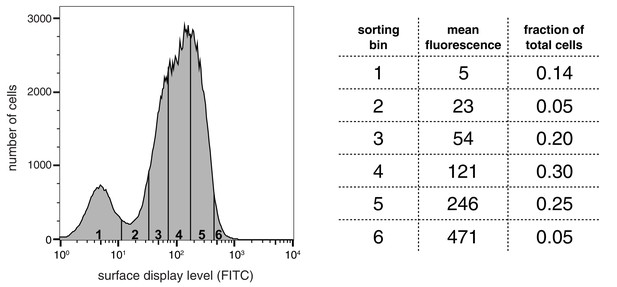
Distribution of surface-display levels and sorting bins for a library of peptides based on segments of LAT, SLP-76, p38α, and TCRζ.
https://doi.org/10.7554/eLife.20105.039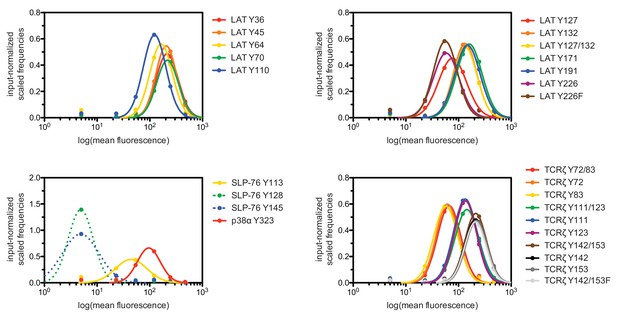
Surface-display distributions for individual peptides in LAT, SLP-76, p38α, and TCRζ library.
Note that fits were approximated for the SLP-76 Tyr 128 and Tyr 145 peptides, as most of the cells bearing this peptide were in the lowest fluorescence bin.
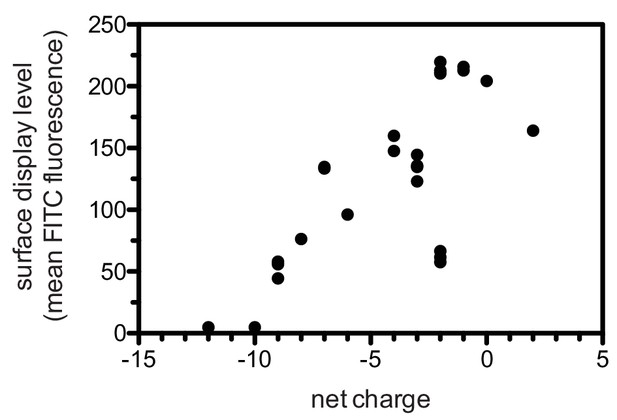
Correlation between surface-display level and net charge of the displayed peptide.
https://doi.org/10.7554/eLife.20105.041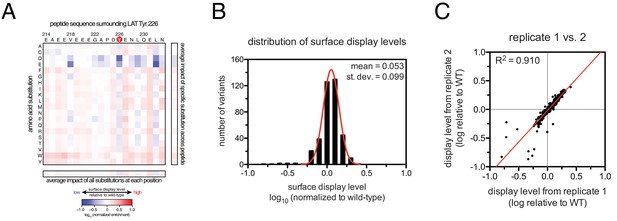
Analysis of surface-display levels for point mutants in the LAT Tyr 226 library.
(A) Heat map depicting the variation in display levels for every member of the library (average values from three independent measurements). (B) Histogram of the distribution of display levels (average values from three independent measurements). (C) Correlation between calculated surface-display levels from two independent replicates.
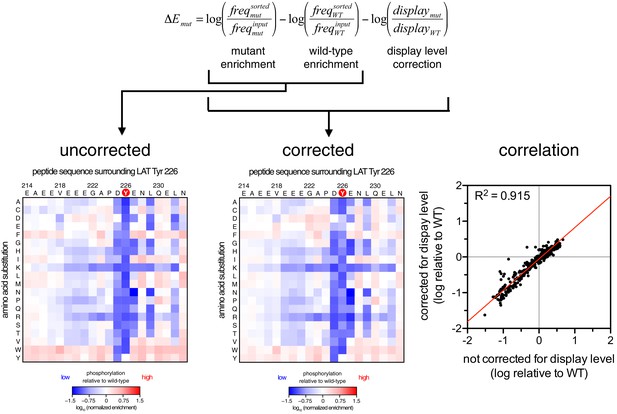
Correction for surface-display level in the dataset for ZAP-70 phosphorylation of the LAT Tyr 226 library.
The uncorrected phosphorylation matrix is shown on the left, the phosphorylation matrix corrected for surface display level is shown in the middle, and the correlation between the uncorrected and corrected datasets is shown on the right.
Additional files
-
Supplementary file 1
Trajectory file for a representative molecular dynamics simulation of the ZAP-70 kinase domain bound to a LAT segment surrounding Tyr 226.
This PDB file contains 500 instantaneous structures sampled every 1 ns from a 500 ns trajectory. The human ZAP-70 kinase domain (residues 328 to 602), ATP, and two Mg2+ ions are defined as chain A. Human LAT residues 219 to 233 are defined as chain B. Numbering in the PDB file corresponds to the conventional numbering of the human protein sequences. Water molecules were removed from the coordinate file for clarity and to constrain the file size. These coordinates correspond to 'run 2' of the five simulations described in Figure 7—figure supplements 2–6.
- https://doi.org/10.7554/eLife.20105.037
-
Supplementary file 2
Script to render MD trajectory in PyMOL.
Upon loading Supplementary file 1 into PyMOL (Schrödinger, 2015), running this script will render the molecules and highlight key residues similar to the images shown in Figure 7.
- https://doi.org/10.7554/eLife.20105.038