Transient protein-protein interactions perturb E. coli metabolome and cause gene dosage toxicity
Figures
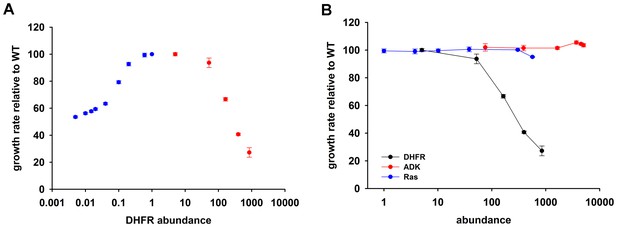
Over-expression of endogenous DHFR is detrimental to bacterial growth.
(A) The effect of variation in DHFR dosage on fitness of E. coli. Change in the intracellular DHFR abundance (in log scale) is plotted against growth rate. DHFR abundance and bacterial growth are normalized against the parameters observed for wild-type strain. In case of over-expression, the growth rates were normalized with values obtained with no inducer (arabinose) (also see Figure 1—source data 1). Controlled drop (over 100 fold) in DHFR abundance (blue circles) was induced by IPTG titration of the chromosomal folA gene of E. coli MG1655 strain at 30°C which was modified to contain LacI binding sites (see Materials and methods and [Bershtein et al., 2013]) and shows a Michaelis-Menten type dependence on fitness described in (Bershtein et al., 2013). A controlled increase (~850 fold) in DHFR abundance (red circles) was achieved by arabinose titration of E. coli BW27783 strain transformed with a plasmid at 37°C carrying the endogenous folA gene under the control pBAD promoter. DHFR over-expression shows a strong dose-dependent drop in fitness (Spearman r = −1, p=0.0167). The obtained abundance vs fitness function shows that the basal endogenous DHFR levels approach a physiological optimum with respect to E. coli growth rate. Overexpression of C-terminal His-tagged EcDHFR generated identical fitness drop (Figure 4—figure supplement 2). (B) Fitness as a function of protein abundance shown for EcDHFR, E. coli Adenylate Kinase (ADK) and a eukaryotic non-endogenous protein H-ras p21 (Ras). Only the expression of DHFR shows a dose-dependent toxicity and, therefore, it is not a generic effect of an over-expression burden. The intracellular DHFR, Ras, and ADK abundances were measured by Western Blot with custom raised antibodies (also see Materials and methods).
-
Figure 1—source data 1
Growth rate and abundance for over-expression (37°C) and down-regulation(30°C) of DHFR.
- https://doi.org/10.7554/eLife.20309.003
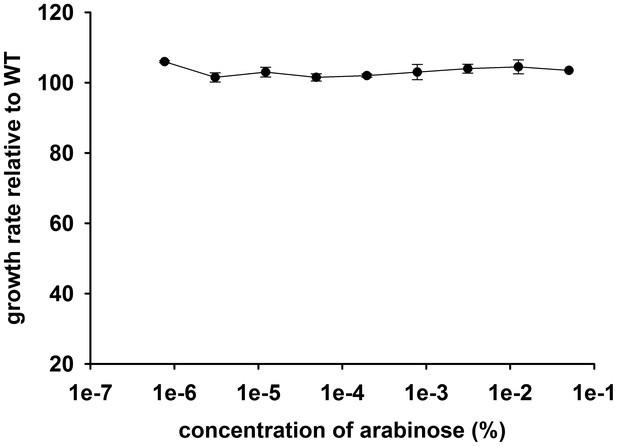
Growth rate (relative to untransformed cells) of E.coli cells transformed with an empty pBAD plasmid over a range of arabinose concentrations.
The empty plasmid does not show any toxicity.
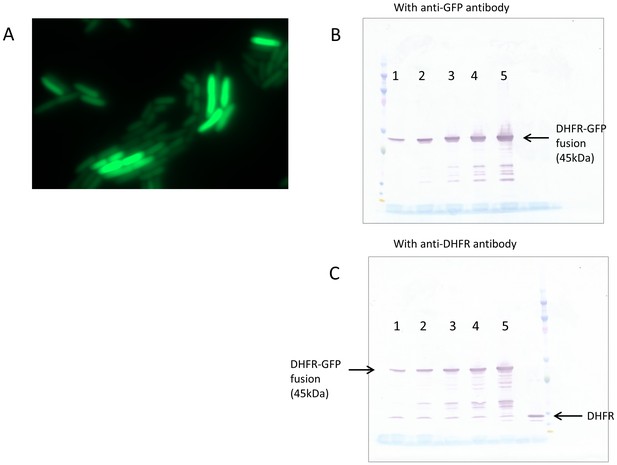
Over-expressed DHFR does not aggregate inside the cell.
(A) DHFR fused to monomeric superfolder GFP was expressed from pBAD plasmid, and fluorescence of the cells was detected using a Zeiss Cell Observer microscope. Intracellular abundance of the fusion protein in the image shown was ~350 fold over chromosomally expressed DHFR. The fluorescence was uniformly spread over the cells, ruling out any substantial aggregation. Over-expressed fusion protein is not cleaved inside the cell, as both (B) anti-GFP and (C) anti-DHFR antibodies showed very minor fraction of cleaved proteins. In panels (B) and (C), lanes 1 to 5 represent different expression levels of DHFR-GFP fusion protein.
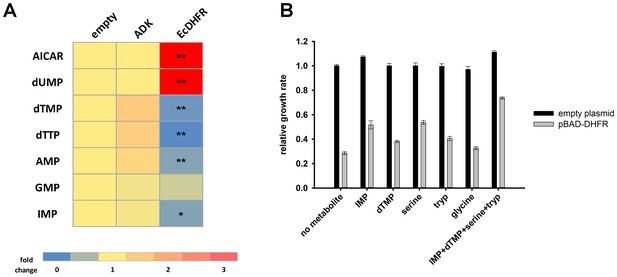
Overexpression of endogenous DHFR triggers a major metabolic shift.
(A) Heat-map of the intracellular levels of various metabolites detected in bacterial cells over-expressing endogenous ADK and DHFR proteins from a pBAD plasmid. While ADK over-expression does not alter the metabolite levels, DHFR over-expression results in a pronounced up-regulation of AICAR and dUMP levels, and down-regulation of purines and pyrimidine nucleotides (dTMP, dTTP, AMP, IMP) (See Materials and methods). In all experiments, sample size was three biological replicates. For statistical significance, levels in DHFR over-expression were compared to ADK over-expression, where * denotes p-value<0.05 and ** denotes p-value<0.001. (B) Metabolic complementation by addition of purine and pyrimidine nucleotides along with several amino acids, like serine and tryptophan, results in a partial rescue of fitness. 1 mM of each of the metabolites was added to supplemented M9 medium at 37°C. Growth rates under different conditions were normalized by the growth rate obtained with empty plasmid without addition of any metabolite, and was referred to as ‘relative growth rate’. For all experiments the arabinose concentration used was 0.05%, which corresponds to ~850 fold over-expression of DHFR and ~5000 fold increase of ADK.
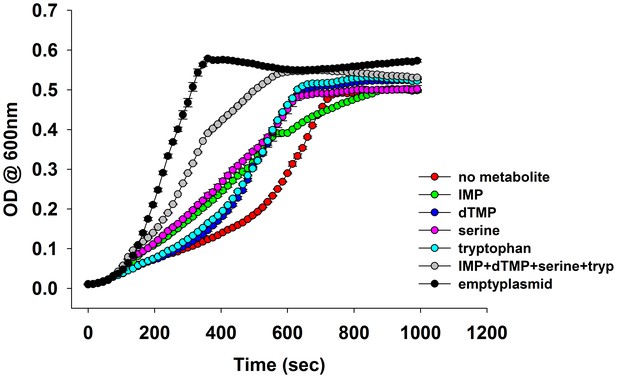
Overlay of growth curves of E.coli over-expressing DHFR and those in the presence of various metabolites.
Addition of 1 mM IMP, dTMP, serine and tryptophan to the medium showed a marked improvement in the growth. For all experiments the arabinose concentration used was 0.05%, which corresponds to ~850 fold over-expression of DHFR.
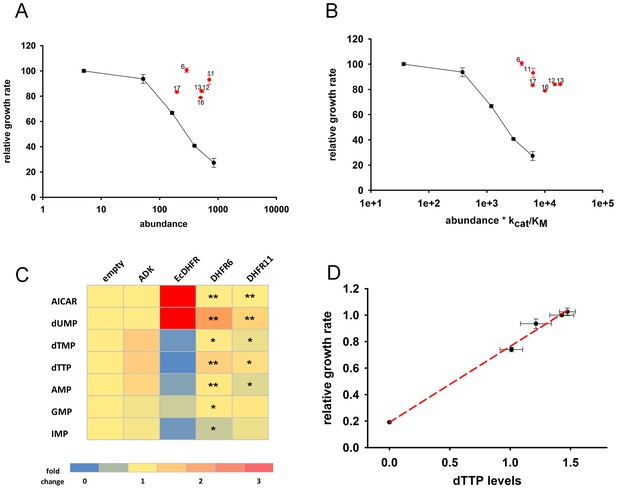
Orthologous DHFR proteins do not cause metabolic imbalance and dosage-related toxicity.
Fitness is shown as a function of (A) intracellular DHFR abundance and (B) (abundance * kcat/KM) for overexpression of E. coli DHFR as well as those from five mesophilic bacteria Listeria innocua (DHFR 6), Bordetella avium (DHFR 11), Leuconostoc mesenteroides (DHFR 12), Aeromonas hydrophila (DHFR 13), Clostridium cellulolyticum (DHFR 16) and Streptococcus dysgalactiae (DHFR 17) expressed from the same plasmid under pBAD-promoter using an inducer concentration of 0.05%. Fitness of cells over-expressing orthologous DHFRs was significantly different from those expressing E. coli DHFR at equivalent concentrations (in all cases, p-value<0.001) (also see Materials and methods) and only endogenous EcDHFR was highly toxic to E. coli. The intracellular abundance of orthologous DHFR was measured by Western Blot with anti-His antibodies (See Materials and methods). Overexpression of orthologous DHFR proteins lacking the His-tag produced identical results (Figure 4—figure supplement 2). (C) Heat-map of the intracellular levels of various metabolites detected in cells overexpressing endogenous ADK and E. coli DHFR as well as two orthologous DHFRs (6 and 11). An empty pBAD plasmid is used as a control. Metabolite levels in orthologous DHFR over-expression were compared to EcDHFR over-expression for statistical significance, where * denotes p-value<0.05 and ** denotes p-value<0.001. Hence when compared to E. coli DHFR, orthologous DHFRs do not cause any major perturbation in the intracellular purine and pyrimidine levels. In all experiments, sample size was three biological replicates. (D) Growth rate of E. coli over-expressing ADK, E. coli DHFR, and orthologous DHFRs 6, 11 and 16 in the presence of 0.05% arabinose is shown as a function of the intracellular dTTP levels. All data were normalized by growth rate of E. coli transformed with the empty plasmid. The data shows that fitness is tightly correlated to cellular metabolite levels (Pearson r = 0.99, p=0.0002).
-
Figure 4—source data 1
Raw and normalized data for metabolomics.
- https://doi.org/10.7554/eLife.20309.009
-
Figure 4—source data 2
Molecular properties of orthologous DHFR proteins.
- https://doi.org/10.7554/eLife.20309.010
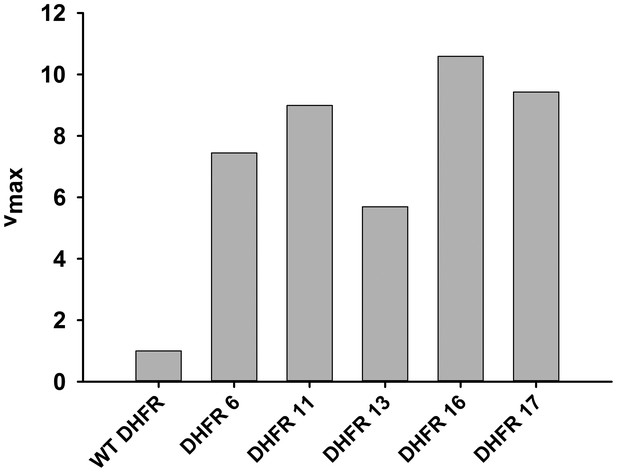
Activity (vmax) of over-expressed DHFR from whole cell lysate.
The initial rate of conversion of 50 µM Dihydrofolate (DHF) to THF was measured in the presence of 100 µM NADPH in 50 mM MES buffer for E. coli over-expressing different DHFRs. As expected from their in vitro activity parameters, over-expressed orthologous DHFR proteins were more active than E. coli DHFR even in the lysate.
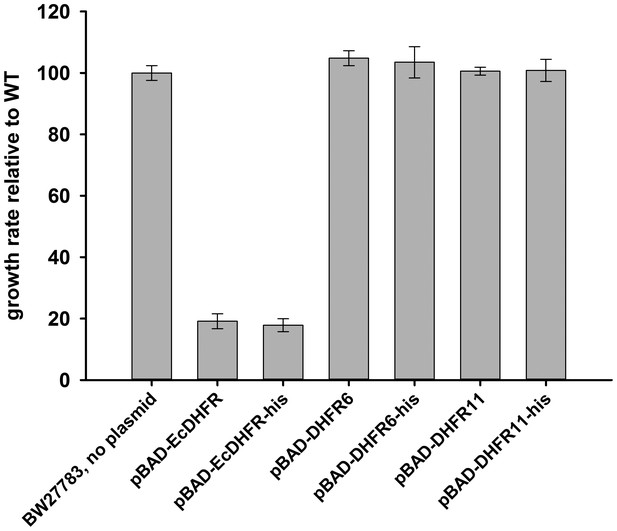
The hexa-histidine tag on DHFR is neutral to fitness.
E. coli DHFR, DHFR 6 and 11 were cloned in pBAD plasmid with and without a C-terminal his-tag and growth rate of BW27783 cells transformed with the plasmids were measured using an inducer concentration of 0.05%.
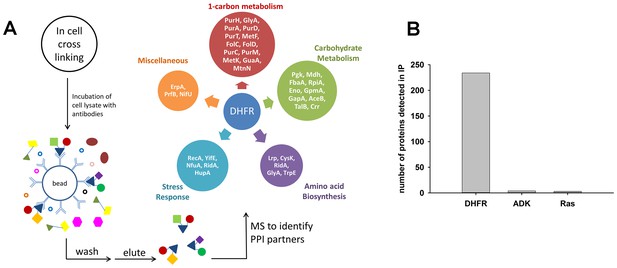
Detection of protein-protein interaction (PPI) partners of E.coli DHFR.
(A) Schematics of the co-immunoprecipitation protocol. Proteins were cross-linked inside the cell using a cell-permeable cross-linker DSP (dithiobis(succinimidyl propionate)), and following lysis, the lysate was captured on anti-DHFR/anti-ADK/anti-Ras/anti-His-tag antibodies to fish out the protein of interest and its PPI partners. The interacting partners were subsequently identified using LC-MS/MS analysis. The observed interactome of overexpressed DHFR could be classified broadly into carbohydrate metabolism and 1-carbon metabolism (purine biosynthesis and amino acid metabolism groups). (B) Over-expressed E. coli DHFR picked out 234 proteins in total, while ADK and Ras detected only 4 and 3 proteins respectively. In case of DHFR, IP datasets were background subtracted using lysate from untransformed WT E. coli cells and DHFR3 (a MG1655 strain where the chromosomal DHFR has been replaced with DHFR from an orthologous bacteria (Bershtein et al., 2015) (also see SI Materials and methods for data analysis). In case of IP for ADK and Ras over-expression, background correction was done with lysate with only untransformed WT E. coli cells. Three biological repeat IP experiments with over-expressed E. coli DHFR and polyclonal anti-DHFR antibody (Figure 5—source data 1) had statistically significant correlation among themselves (average r = 0.8, p<0.001).
-
Figure 5—source data 1
Consolidated IP data for all proteins.
- https://doi.org/10.7554/eLife.20309.014
-
Figure 5—source data 2
Consolidated list of binding partners of EcDHFR detected using anti-his and/or anti-EcDHFR antibodies.
- https://doi.org/10.7554/eLife.20309.015
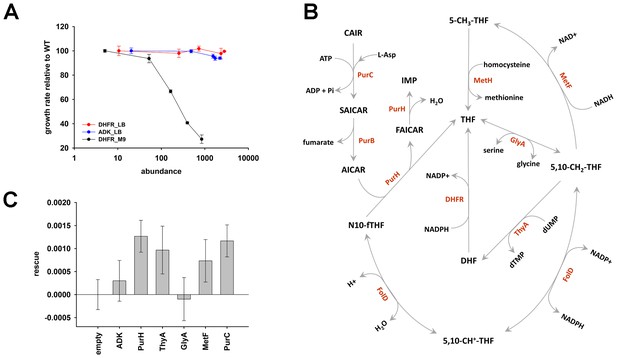
The role of purine biosynthesis and 1-carbon metabolism enzymes in DHFR over-expression toxicity.
(A) Unlike supplemented M9 medium, over-expression of DHFR is not toxic in rich medium (LB) in terms of growth rate (Spearman r = −0.3, p=0.7), (note, however, that saturation ODs at 600 nm is lower in LB in comparison to growth in M9; see Figure 6—figure supplement 1). This indicates the role of conditionally essential genes like purH, purC, metF, etc. in determining the over-expression toxicity of DHFR. All data were normalized by growth rate of E. coli transformed with the empty plasmid. (B) Schematics of the 1-carbon pathway metabolic pathway. GlyA, ThyA, MetF are important enzymes that function immediately downstream of DHFR and utilize THF or its derivative. (C) Partial rescue of fitness of DHFR over-expressing E. coli by a dual-expression system. DHFR was expressed from a IPTG-inducible plasmid while PurH, ThyA, GlyA, MetF and PurC along with a negative control protein ADK were expressed separately from an arabinose-inducible pBAD system. Rescue factor is defined as , where is the growth rate, and X is the corresponding protein.
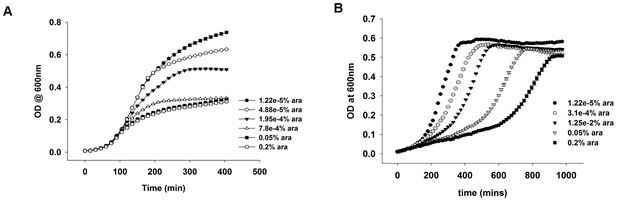
OD vs time curves for DHFR over-expression in (A) rich media (LB) and (B) in M9 medium supplemented with amino acids.
In LB, the growth rate is not affected due to over-expression, though the cells cannot grow to the same saturation ODs. In M9 however, the growth rate is severely affected, while the saturation ODs remain largely unchanged.
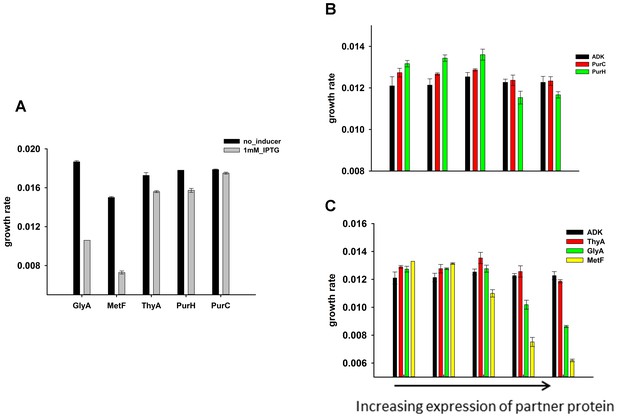
Effect of over-expression of purine biosynthesis and 1-carbon metabolism pathway proteins on growth rates of E.coli (A) alone and (B), (C) on the background of DHFR over-expression.
GlyA and metF are significantly toxic at the highest induction level, ThyA and PurH show moderate toxicity while PurC was found to be non-toxic. On the background of DHFR over-expression (basal expression from pTRC plasmid), expression of the partner proteins was beneficial at low expression levels, however eventually become toxic at higher inducer concentrations.
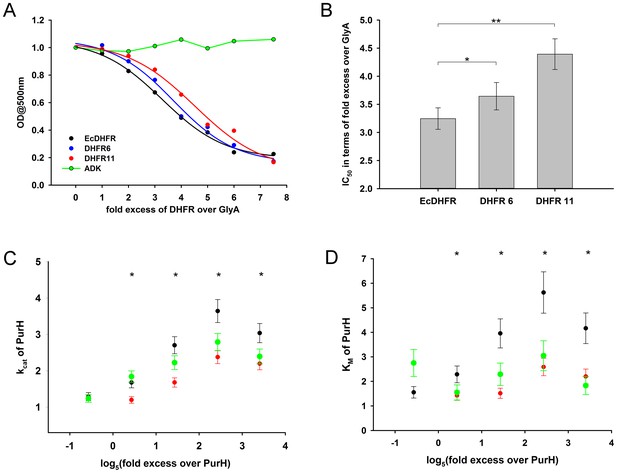
Compared to orthologous DHFRs, E.coli DHFR is a more potent inhibitor of GlyA and PurH.
(A) Formation of a ternary complex (GlyA-PLP+glycine + 5f-THF) was monitored at 500 nm as a function of increasing amounts of different DHFRs and the negative control protein ADK. 20 µM GlyA was pre-incubated with varying concentrations of DHFRs and ADK (zero to 150 µM) before 0.2M glycine and 200 µM 5f-THF were added to it. All data were normalized by those in the presence of an equal volume of buffer. Data were fit to a 4-parameter sigmoidal function to extract the fold excess of DHFR required to achieve 50% inhibition (IC50) as shown in panel (B). Though all DHFRs caused inhibition of GlyA compared to ADK, EcDHFR had a significantly lower IC50 than DHFR6 (* denotes p-value<0.05) and DHFR11 (** denotes p-value<0.001), explaining its higher potency in sequestering GlyA and, hence, toxicity. Catalytic rate kcat (C) and Michaelis coefficient KM (D) of PurH for 10Nf-THF measured in the presence of different DHFRs and ADK. All values were normalized relative to those of PurH measured in the absence of added protein. 250 nM of PurH was pre-incubated with varying concentrations of DHFRs or ADK (0.1 µM, 0.5 µM, 2.5 µM, 12.5 µM and 60 µM) and, subsequently, the initial rate of transfer of formyl group (AICAR transformylase activity) from 10N-formyl THF to AICAR was measured at 298 nm. For determination of kcat and KM at each concentration of a protein, the concentration of 10Nf-THF was varied from 20 µM to 1 mM, while AICAR concentration was fixed at saturation (500 µM). Each data point is an average of 3–5 independent measurements and the error bars represent standard deviation. For all proteins, kcat increased with increasing concentration of protein added, dropping off slightly at the highest concentration. The mechanism of this effect is not fully understood, but can be partially attributed to the generic crowding effect of proteins. However, only EcDHFR caused a concentration dependent increase in KM of PurH for 10Nf-THF, thereby explaining its selective toxicity upon over-expression. Statistical analyses were done to compare kcat and KM values of EcDHFR (black) and DHFR11(red), and in all cases indicated by *, the p-value was <0.05.
-
Figure 7—source data 1
Kinetic parameters for binding of purified purH and glyA proteins to surface immobilized DHFRs by surface plasmon resonance (Biacore).
- https://doi.org/10.7554/eLife.20309.020
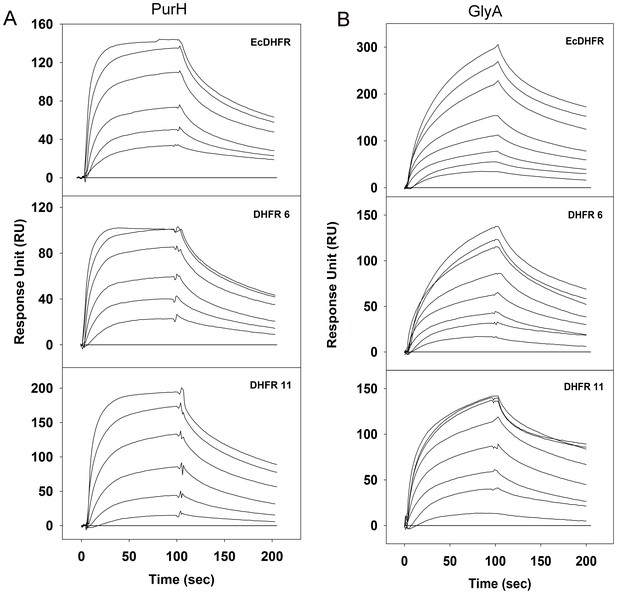
Binding of purified PurH and GlyA proteins with EcDHFR, DHFR 6 and 11 in vitro detected using surface plasmon resonance.
700–900 RUs of purified His-tagged DHFRs were immobilized on a CM5 chip and (A) PurH and (B) GlyA proteins were flown over the surface at 25°C. For panel (A), curves from bottom to top represent 1.5 µM, 3 µM, 6 µM, 12 µM, 24 µM and 48 µM of purified PurH. For panel (B), curves from bottom to top represent 1 µM, 2 µM, 4 µM, 8 µM, 16 µM, 32 µM, 48 µM and 64 µM of purified GlyA. His-tagged ADK protein was immobilized on the negative control channel and was subtracted from all binding data (baseline shown in all three plots).
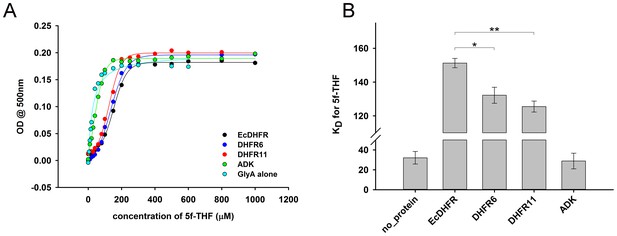
Determination of equilibrium dissociation constant (KD) of binding of 5f-THF to GlyA (A) Formation of a ternary complex (GlyA-PLP+glycine + 5f-THF) was monitored at 500 nm as a function of increasing amounts of 5f-THF in the presence of different DHFRs/ADK.
20 µM GlyA was pre-incubated with 60 µM of DHFRs/ADK and the concentration of 5f-THF was varied from 0 to 1 mM. (B) While addition of both EcDHFR as well as the orthologs increased the KD of 5f-THF binding to GlyA substantially, EcDHFR caused the largest increase, and therefore inhibited GlyA the most. Presence of ADK did not perturb the KD, indicating that the effect of DHFRs was highly specific. For significance tests, * indicates p-value<0.05, while ** indicates p-value<0.001.
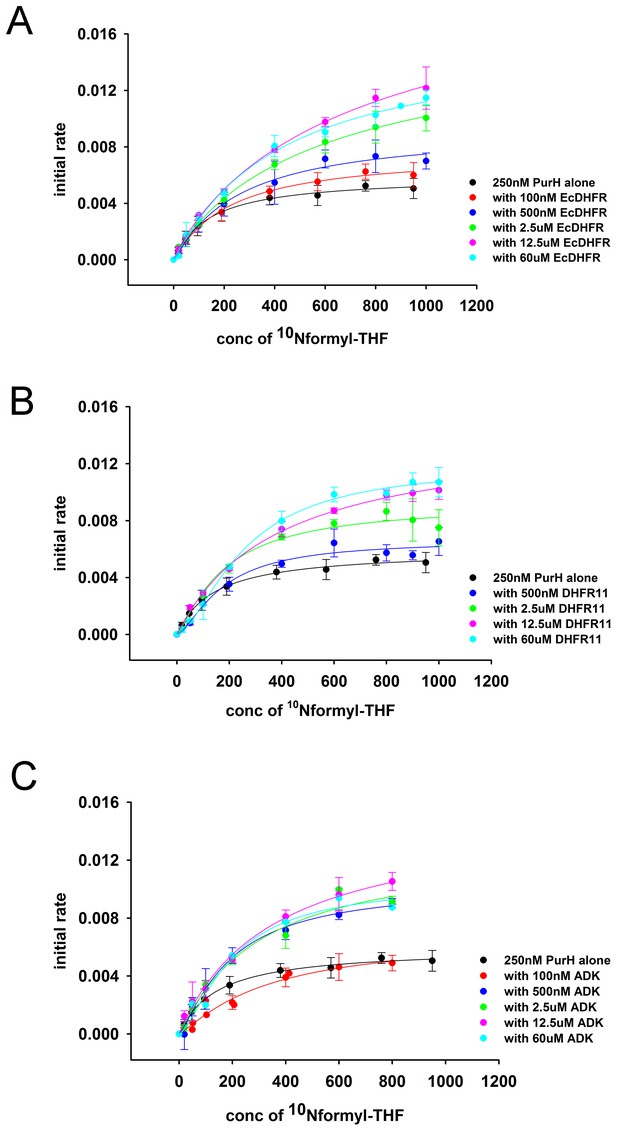
Overlay of initial rate (maximum rate) of PurH activity as a function of 10Nf-THF concentration at different concentrations of added protein (A) EcDHFR (B) DHFR 11 and (C) ADK.
For all proteins, there is steady increase in the initial rates with addition of extra protein.
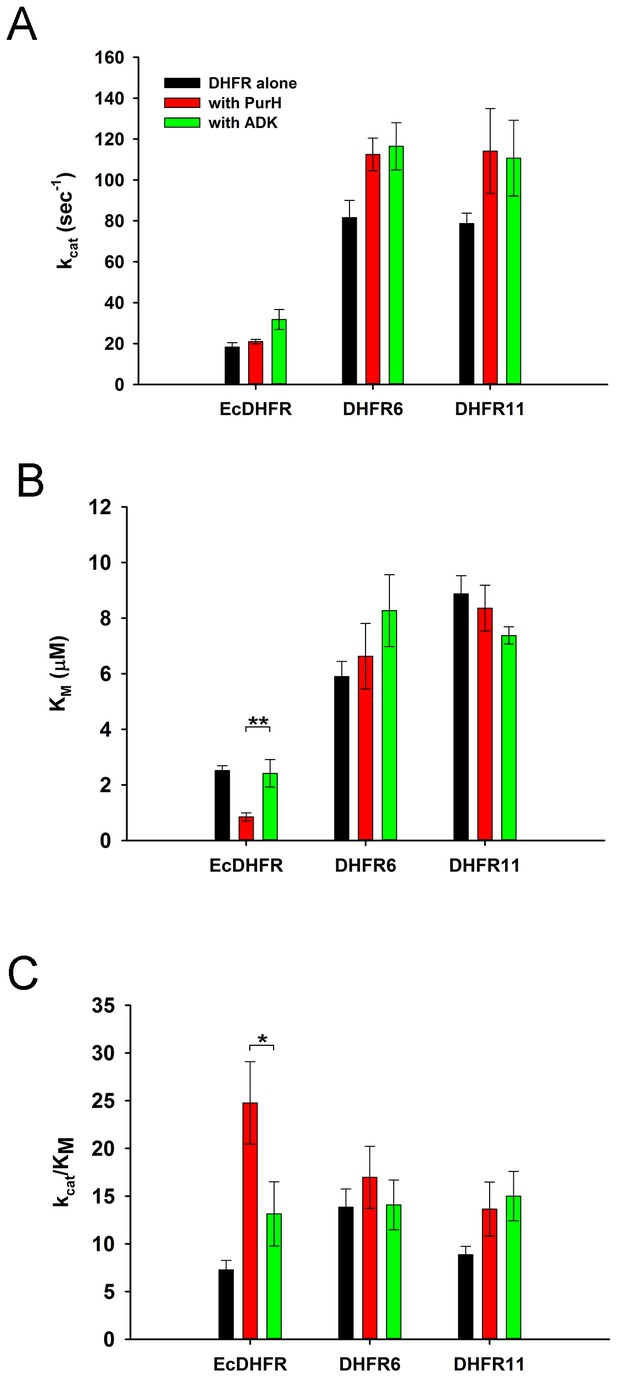
PurH has a selective beneficial effect on EcDHFR activity only (A) Catalytic rate (kcat), Michaelis coefficient (KM), and the catalytic efficiency (kcat/KM) of EcDHFR, DHFR6 and DHFR11 for dihydrofolic acid (DHF) in the presence of low concentrations of PurH.
10 nM DHFR were pre-incubated with 15 nM of PurH or ADK and, subsequently, the initial rate of conversion of NADPH to NADP+ was measured at 340 nm. For determination of kcat and KM, the concentration of DHF was varied from 0.1 µM to 16 μM for EcDHFR and from 1 μM to 64 μM for DHFR6 and 11, while NADPH concentration was fixed at saturation (150 µM). Each data point is an average of 3–5 independent measurements and the error bars represent standard deviation. Both PurH and ADK resulted in an increase in kcat of all the DHFRs, however PurH caused a significant drop in KM and a concomitant increase in kcat/KM for EcDHFR only. In all panels, * indicates p-value<0.05, while ** indicates p-value<0.001. Therefore, at physiological ratios of proteins, PurH is only beneficial for EcDHFR. This observation suggests an evolutionary functional relationship between PurH and EcDHFR at physiological concentrations. Orthologous DHFRs that have diverged during the course of evolution no longer have this benefit from E. coli PurH.