Atrophin controls developmental signaling pathways via interactions with Trithorax-like
Figures
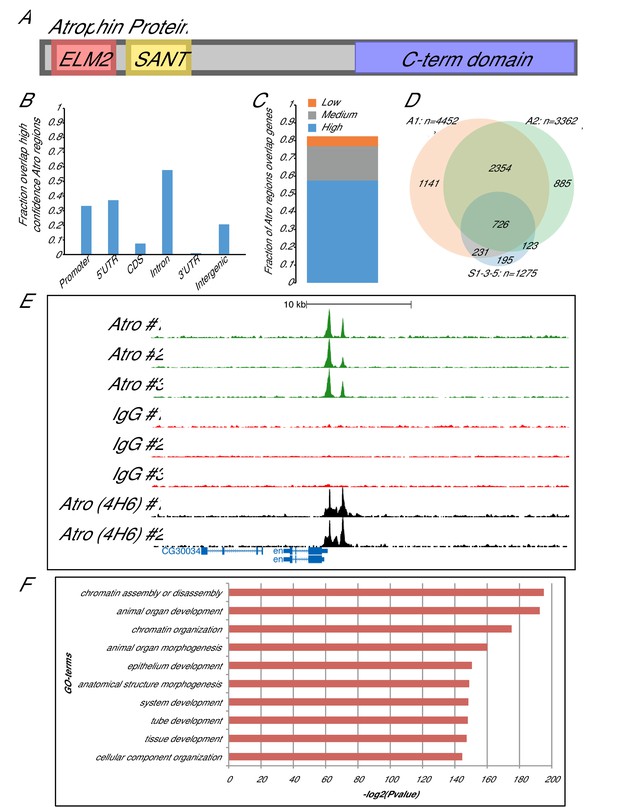
Atrophin ChIP-seq results.
(A) A schematic of Atro protein is shown. Atro’s N-terminal side has ELM2 and SANT domains to interact with HDAC1 and G9a, respectively. Atro’s C-terminal domain interacts with Tailless and Fat. (B) Fraction of the common Atro regions overlapping with various genomic features (genome release 5.57) (Attrill et al., 2016). (C) The fraction of common Atro regions overlapping genes (including 500 bp upstream of transcription start site) with high, medium or low expression in S2 cells (data from [Cherbas et al., 2011]). (D) Venn diagram showing overlap of the common regions from three biological replicates (S1-3-5) with two additional, independent biological replicates (A1 and A2) using a different Atro antibody (4H6). Only the peaks at the major chromosome arms (heterochromatin are excluded) are compared, and thus, only 1275 peaks are used in S1-3-5 for this analysis instead of 1377 peaks. (E) Example overlap of Atro ChIP-seq peaks at the engrailed (en) locus. Atro #1–3 are the triplicates Atro ChIP-seq data; IgG #1–3 are the corresponding IgG ChIP-seq controls for the Atro ChIP-seq data. Atro (4H6) #1–2 are the independent Atro ChIP replicates (shown in D). (F) Top 10 GO-term enrichment hits of the Atro ChIP-seq data. GO-term enrichments were done with PANTHER overrepresentation test with default parameters and Bonferroni correction (Mi et al., 2016).
-
Figure 1—source data 1
PANTHER GO-Term enrichment results (Mi et al., 2016) for Figure 1F.
- https://doi.org/10.7554/eLife.23084.004
-
Figure 1—source data 2
Overlap of ChIP data sets with the three classes defined in PCA analysis of Atro peaks (modENCODE (downloaded from http://intermine.modencode.org/) and CBP [Philip et al., 2015]) for Figure 1—figure supplement 1B.
- https://doi.org/10.7554/eLife.23084.005
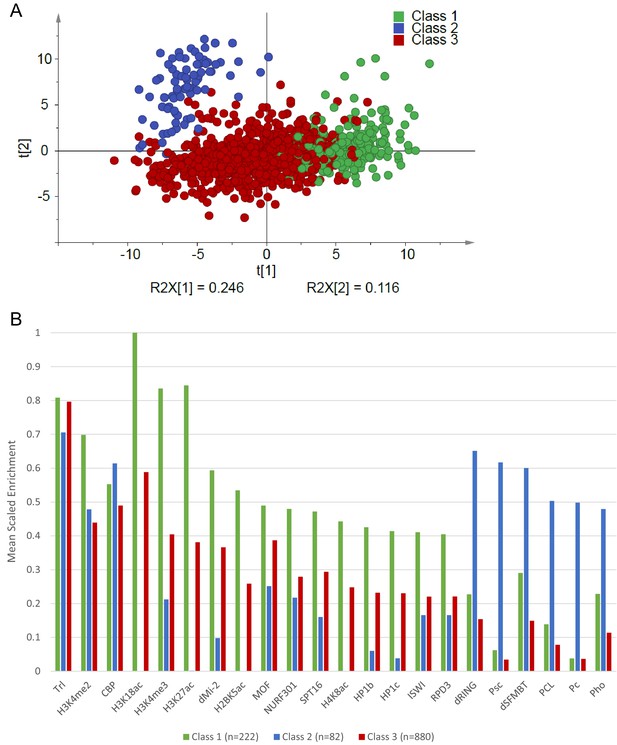
Principal component analysis of Atro peaks.
(A) Score plot showing the first two components from principal component analysis using enrichment of all factors mapped by modENCODE (S2 cells) within Atro-binding regions. The Atro-binding regions are colored according to the three classes defined by hierarchical clustering. R2X values represent the fraction of the total variation explained by each component. (B) The mean scaled enrichment values for each factor withing the three classes. All factors with enrichment values below 0.4 in all classes were excluded.
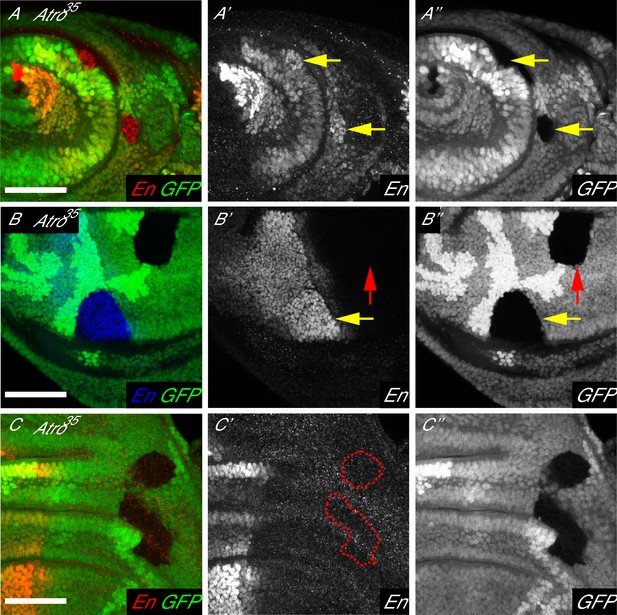
Atro is required for modulating En levels in larval imaginal discs.
(A) Antennal disc clones of Atro35 have increased En levels (arrows). (B) Atro35 wing disc clones in the posterior compartment have increased En levels (yellow arrow). But Atro35 clones cannot induce ectopic En expression in the anterior compartment (red arrow). (C) Atro35 clones cannot induce ectopic En expression (dotted lines mark clonal borders, wing disc is shown here). (A) has posterior to the right; (B–C) have posterior to the left, dorsal up. All scale bars are 50 μm.
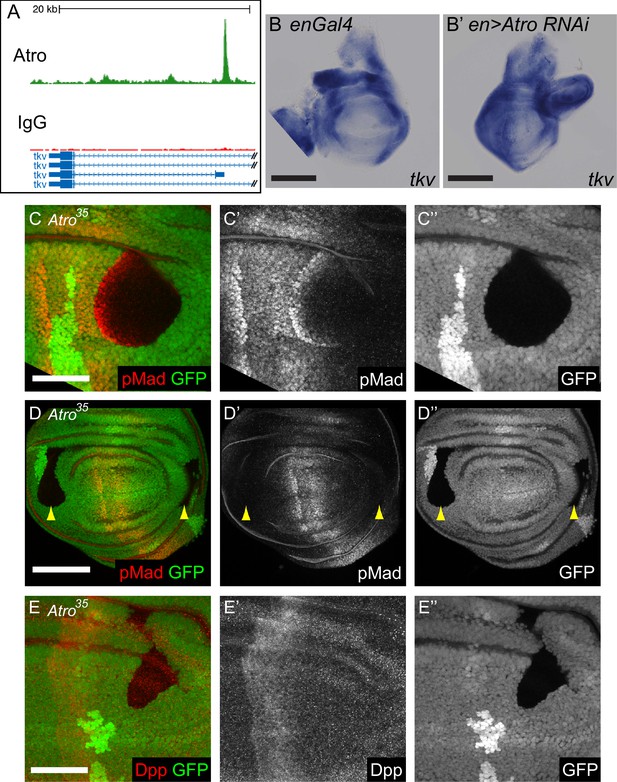
Atro regulates Dpp signaling.
(A) An Atro ChIP peak is found inside the tkv locus. This peak is directly upstream of the transcription start site of tkv isoform D. (B) In situ hybridization showing enGal4 control wing disc with normal tkv expression. (B’) shows in situ hybridization of Atro RNAi driven in the posterior half of the wing disc by enGal4. tkv expression is increased in the posterior half. (C) Atro35 wing disc clones that cross the endogenous pMad regions have increased pMad levels along the interior border of the clone that is closest to the middle of the disc (where the Dpp source is located). This indicates that Atro35 clones have increased Dpp signaling. (D) Atro35 wing disc clones cannot cause ectopic Mad phosphorylation (yellow arrow heads). (E) Atro35 wing disc clones cannot induce ectopic Dpp. All clones are marked by the absence of GFP; all figures have posterior to the left, dorsal up. Scale bars in A and C are 100 μm; in B and D are 50 μm.
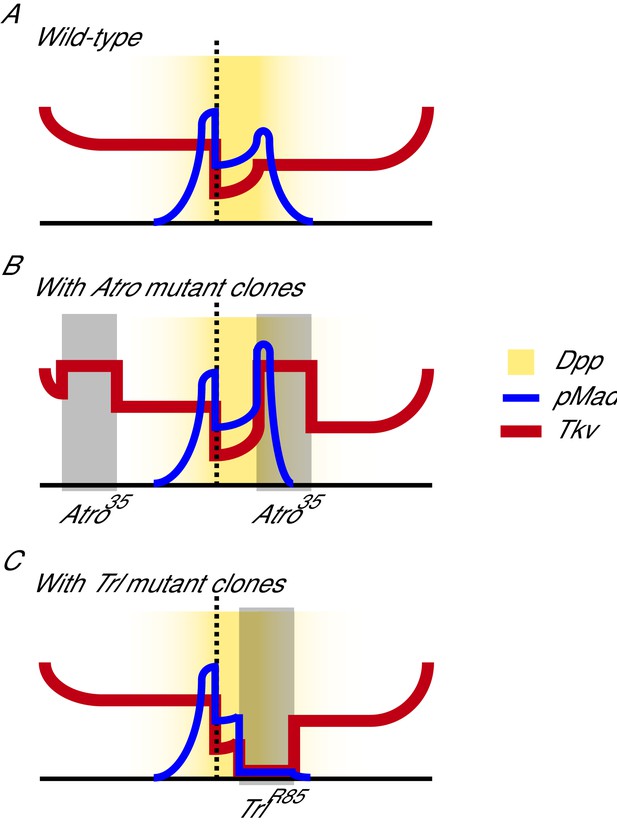
Model of how loss of Atro and Trl affect Dpp signaling in wing discs.
(A) Dpp signaling in the wild-type situation is shown (Tanimoto et al., 2000). (B) In Atro35 clones (dark rectangle), Tkv protein levels are increased. The increase of receptors causes pMad to increase near the interior clonal border closest to the Dpp source. Atro35 clones near the anterior/posterior edges of the wing also increase Tkv levels but does not affect pMad levels. (C) TrlR85 clones (dark rectangle) cause a loss of Tkv protein levels and thus pMad levels also decrease within the clones.
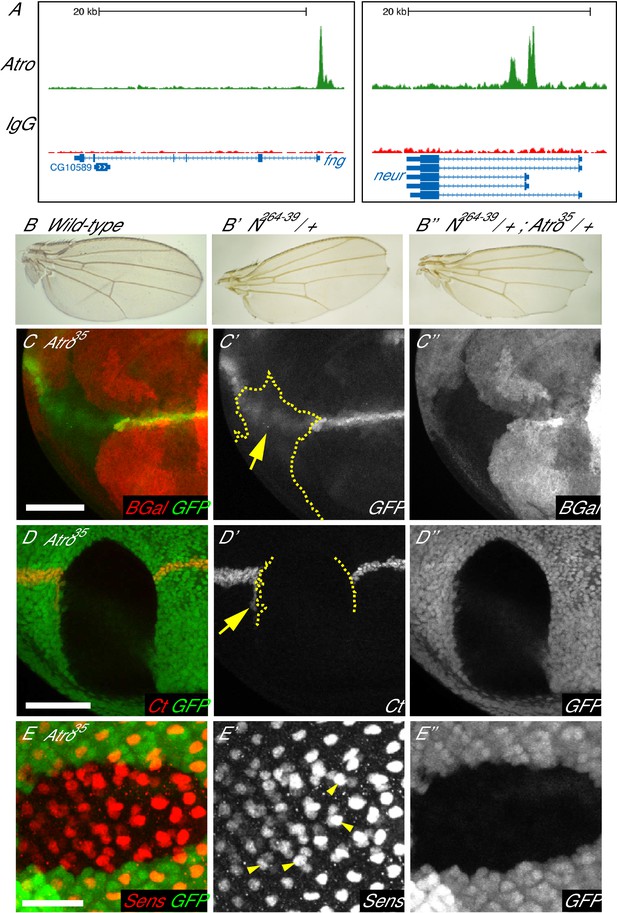
Loss of Atro leads to loss of Notch signaling phenotypes.
(A) Atro ChIP peaks are found in the fng and neur loci, indicating that Atro may regulate these genes during development. (B) Wild-type wings have no notches at the wing margin. N264-39 heterozygous wings have wing notches (B’). Wing notch phenotype is enhanced in transheterozygous N264-39, Atro35 wings (B’’). (C) Atro35 clones cause the Notch signaling reporter (GFP) to express in a diffused pattern (arrow). Dotted line marks clonal borders. (D) Large Atro35 clones cause an autonomous loss of wing margin marker Cut as well as ectopic Cut expression on the posterior side of the clone (arrow). Clonal borders are marked with dotted lines. (E) Atro35 clones have extra cells expressing the early R8 marker, Sens. Extra Sens—positive cells are found in clusters of two to three cells but one cell within each cluster has more Sens staining than the rest (arrowheads). Clones are marked by absence of ß-Gal (red) in C and clones are marked by the absence of GFP in D and E. B have posterior to the bottom and C to E have posterior to the left. C and D have dorsal side up. Scale bars in C and D are 50 μm; in E is 25 μm.
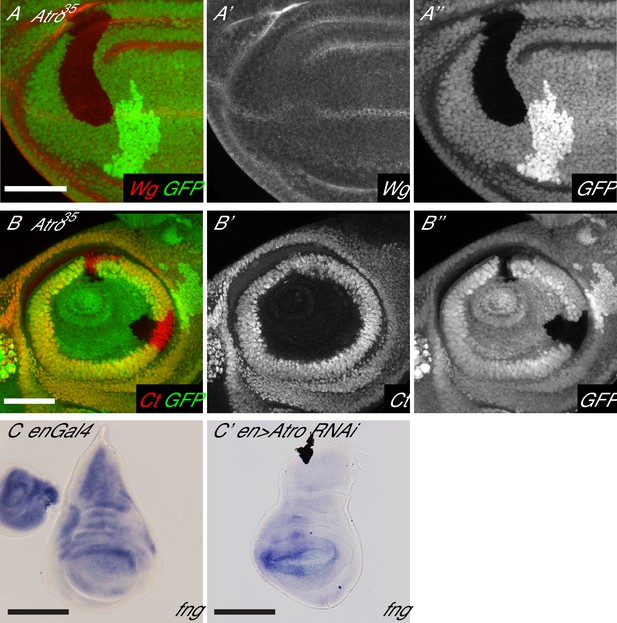
Loss of Atro causes loss of wing margin markers via Notch signaling.
(A) Atro35 clones have an autonomous loss of Wg expression. (B) Atro35 clones do not affect Cut expression in the antennal disc. (C) In situ hybridization of enGal4 control wing disc with normal fng expression. (C’) In situ hybridization of a wing disc with Atro RNAi driven in the posterior half by enGal4. fng expression is increased in the posterior half. A and C have the posterior side to the left, dorsal side up. B has posterior to the right. Scale bars are 50 μm in A and B; 100 μm in C.
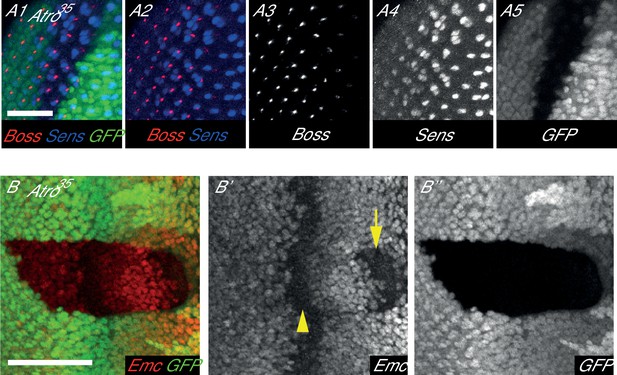
Atro35 clones have normal Boss expression but altered Emc levels.
(A) Atro35 clones have extra Sens-positive cells (blue) but normal amount of Boss staining (red). (B) Atro35 clones have reduced Emc levels (arrow) anterior to the morphogenetic furrow (arrowhead) but normal levels posterior to the furrow. All clones are marked by absence of GFP. All pictures have posterior on the left. Scale bar in A is 25 μm; in B is 50 μm.
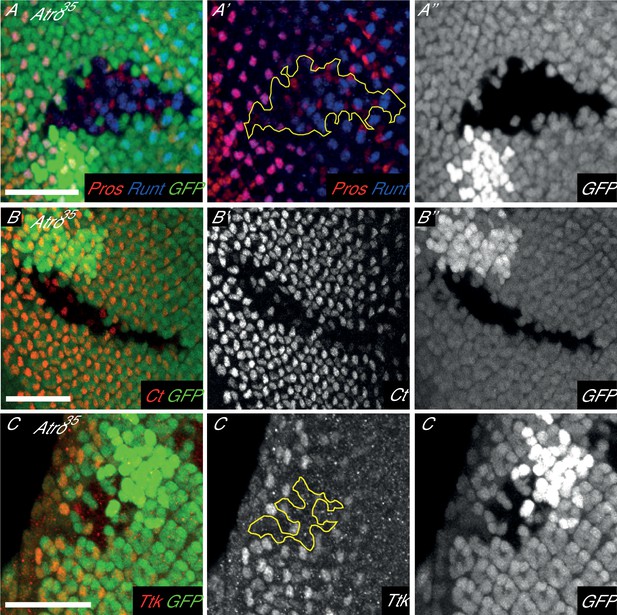
Loss of Atro have eye phenotypes similar to loss of Notch signaling.
(A) Atro35 clones have a loss of R7 cells. R7 cells are marked by Pros and Runt co-expression (purple). Yellow outline marks the clone boundary. (B) Atro35 clones have a loss of Cut expression but some Cut positive cells are still found inside the clone. (C) Atro35 clones have a loss of Tramtrack isoform 69 (Ttk69) expression. Clonal boundary is marked by yellow outline. Clones are marked by the absence of GFP. All pictures have posterior to the left. Scale bars are 25 μm.
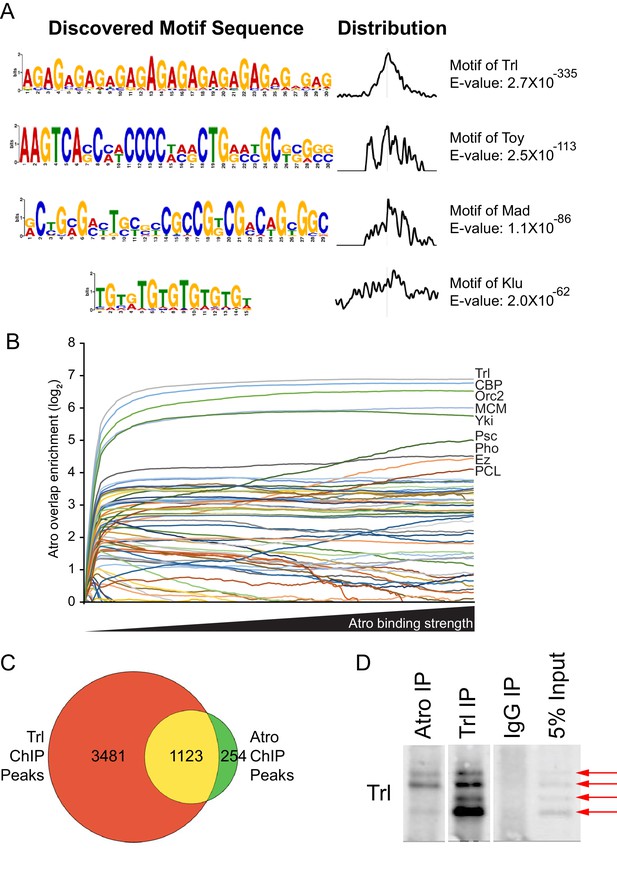
Atro and Trl bind to the same genomic locations.
(A) The top four hits from MEME-ChIP analysis using all Atro peaks. The top hit is a (GA) repeat, which is the binding motif of Trl. (B) Atro ChIP-seq data overlap with other factors in S2 cells. The y-axis represents the overlap over that expected by chance, where the fraction of the genome covered by each factor is the overlap expected by chance. Only positive values are shown. The Atro values are selected with increasing cut-off, so that fewer but stronger Atro-binding sites are shown along the x-axis. (C) shows the Venn diagram of the overlap of all Atro and Trl ChIP data. All 1377 Atro ChIP peaks were used and Trl ChIP peaks are from Fuda et al. (2015). Genomic coordinates from both data sets were used to construct this diagram.
-
Figure 5—source data 1
List of Atro peaks’ average –log(pvalue) and fold enrichment generated from MACS2 for Figure 5—figure supplement 2.
Atro peaks that intersect Trl were compared with peaks that do not intersect Trl.
- https://doi.org/10.7554/eLife.23084.015
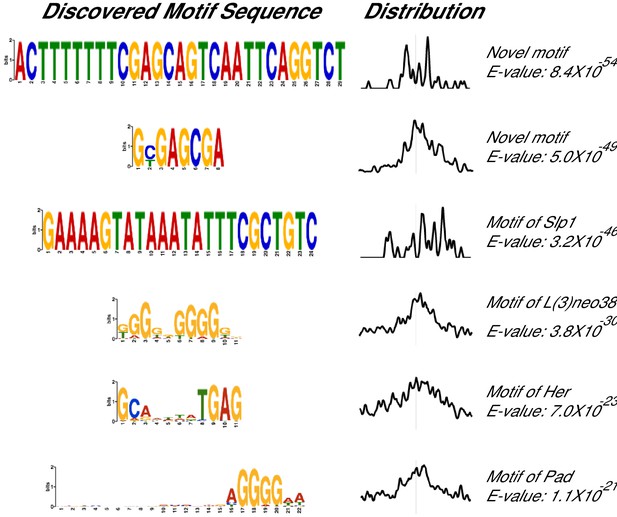
Additional MEME ChIP hits of Atro ChIP-seq.
These MEME ChIP results are the 5th-12th top hits of the MEME ChIP experiment.
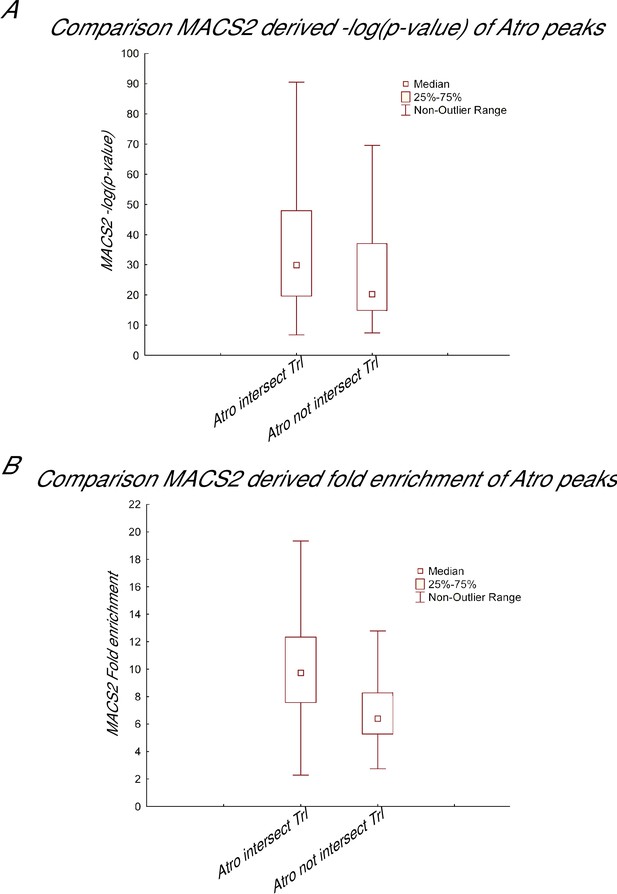
Comparison between Atro peaks that overlap Trl and those that do not.
(A) Box and whisker plot of Atro peaks based on MACS2 derived –log(pvalue), which is an indication of the significance of called peaks. (B) Box and whisker plot of Atro peaks based on MACS2-derived fold enrichment values for each called peaks. Both A and B shows Atro peaks that intersect with Trl peaks are more significant (higher –log(pvalue)) and have more fold enrichment than Atro peaks that do not intersect Trl (Wilcoxon test p-value<0.001 for both plots).
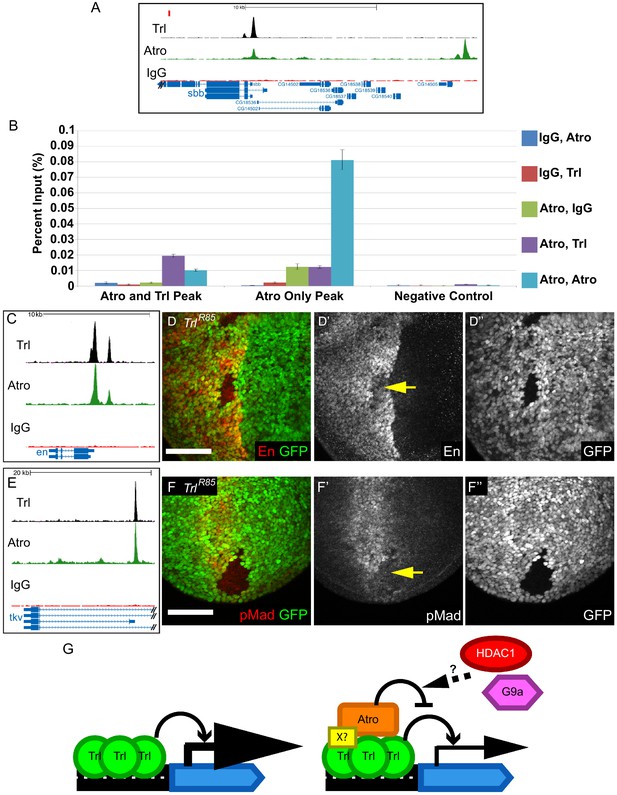
Trl and Atro bind to the same loci simultaneously and Trl is required for the expression of en and tkv.
(A) There is an Atro peak that overlaps with Trl peak within the sbb locus (left peak, Atro and Trl Peak in B). There is an Atro peak that does not overlap with Trl about 20 kb upstream of the sbb locus (right peak, Atro Only Peak in B). The red rectangle marks the region used as negative control in B. (B) ChIP-re-ChIP qPCR results. ChIP-re-ChIP samples are labeled as the sequence of antibody used (e.g. IgG, Atro means rabbit IgG ChIP followed by Atro re-ChIP). Negative controls for the ChIP-re-ChIP are any ChIPs with IgG. Atro, Trl ChIP-re-ChIP enriches the Atro and Trl Peak (purple bar) but it does not enrich the Atro only peak (same enrichment as Atro, IgG). Mean Ct value was used to calculate percent input and standard deviation of the Ct values was carried over in calculations and used as error bars. (C) Trl and Atro ChIP peaks coincide at the same loci upstream of en. (D) TrlR85 imaginal disc clones have decreased En levels (arrow, wing disc clone shown here). (E) Trl and Atro ChIP peaks coincide at the tkv locus. (F) TrlR85 wing disc clones have decreased pMad levels (arrow), possibly due to a decrease of tkv expression. All clones are marked by the absence of GFP; all figures have posterior to the left, dorsal up. Scale bars are 50 μm. (G) Model of how Atro and Trl function together to regulate expression of target genes. Trl is required to activate the transcription of its target genes. In the absence of Atro (Left), the target gene will express at a higher level than normal. Atro binds to the same site as Trl either directly or via some unknown cofactors (X?, Right). Atro modulates the expression of its target gene by counteracting Trl; potentially Atro is doing so by recruiting Histone deacetylase 1 (HDAC1) and G9a, a histone methyl transferase. Thus, target genes are expressed at the correct levels.
-
Figure 6—source data 1
Source data for qPCR %Input calculations for Figure 6B and Figure 6—figure supplement 1.
- https://doi.org/10.7554/eLife.23084.019
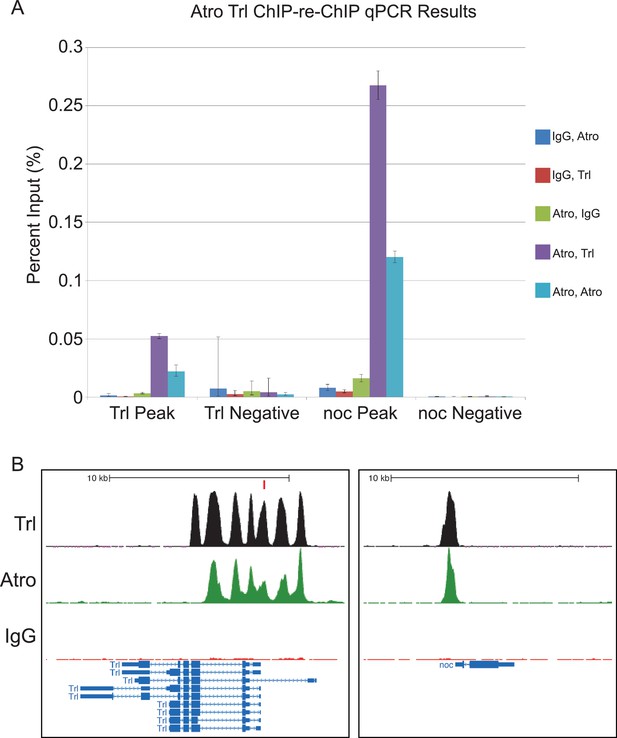
Additional ChIP-re-ChIP qPCR results of the Trl and no ocelli (noc) loci.
(A) Trl Negative and noc Negative are negative control loci for both genes. ChIP-re-ChIP samples are labeled in the sequence of antibody used (e.g. IgG, Atro means rabbit IgG ChIP followed by Atro re-ChIP). Negative controls for the ChIP-re-ChIP are any ChIPs with IgG. Atro and Trl ChIP-re-ChIP enriches the Trl and noc peaks (purple bar). Mean Ct values were used to calculate percent input and standard deviation was carried over in calculations to be used as error bars. (B) Atro (green) and Trl (black) ChIP peaks overlap at the same positions at the Trl and noc loci. The red rectangle in the Trl loci figure shows where the Trl primer set amplifies for the qPCR in A.
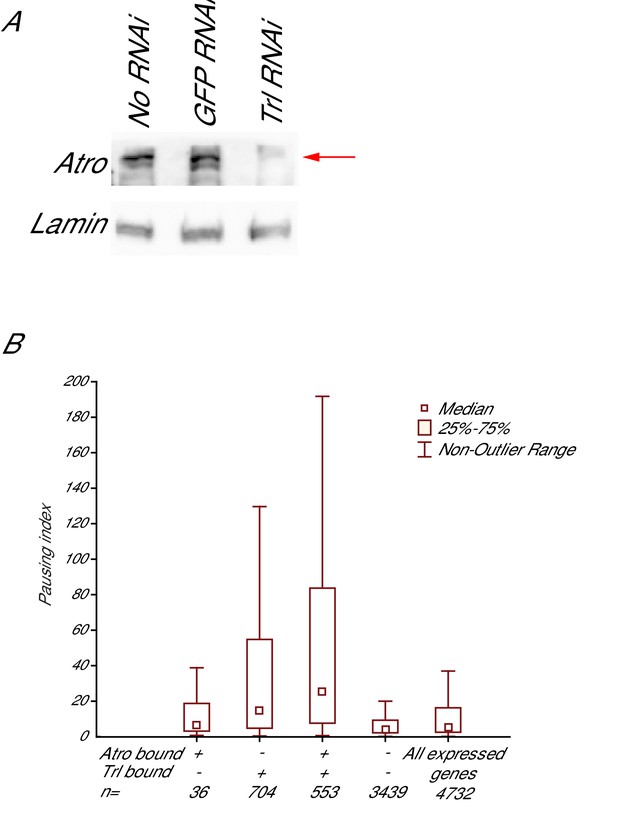
Trl knockdown decreases Atro protein levels, coimmunoprecipitation of Trl and Atro, and pausing indices of Trl and Atro bound genes.
(A) Trl knockdown by RNAi causes Atro protein levels to decrease (arrow), while no RNAi or GFP RNAi treatments do not affect Atro protein levels. Lamin was used as a loading control. (B) shows the coimmunoprecipitation of Atro and Trl. Each lane is labeled with the antibody used for each IP. Blot was stained with Trl antibody. The red arrows mark the Trl bands. Trl is coimmunoprecipitated with Atro but not with IgG control. (C) The pausing index (amount of Pol II in the promoter-proximal region versus the gene body calculated from PRO-seq data (Kwak et al., 2013) is higher on average for genes bound by Atro and Trl than for all other expressed genes in S2 cells.
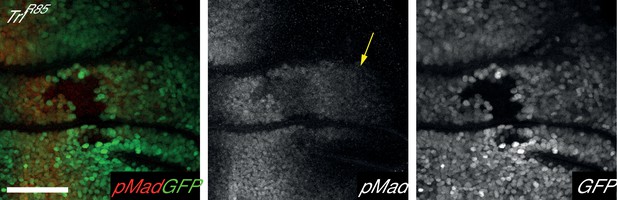
TrlR85 clones cause an autonomous decrease Mad phosphorylation but do not affect pMad levels outside clone (arrow).
Clones are marked by the absence of GFP. Posterior is to the left, dorsal upwards. Scale bar is 50 µm.
Additional files
-
Source code 1
Script used to calculate overlap between data sets as Atro binding increases.
Perl script used to count the frequency of overlap between various data sets in gff3-format to a reference gff3 file. Each data set is divided into a user defined number of bins by equally distributed cut-offs by value or by rank. Alternatively, the bins can be created so that all data points below each cut-off are merged into one bin. The frequency of overlap between the reference file data to the data points in each bin is reported.
- https://doi.org/10.7554/eLife.23084.023
-
Source code 2
Script used to calculate overlap between data sets as Atro binding increases.
Perl script used to count the frequency of overlap between various data sets in sgr-format to a reference gff3 file. Each data set is divided into a user defined number of bins by equally distributed cut-offs by value or by rank. Alternatively, the bins can be created so that all data points below each cut-off are merged into one bin. The frequency of overlap between the reference file data to the data points in each bin is reported.
- https://doi.org/10.7554/eLife.23084.024
-
Source code 3
Perl script used to calculate the mean enrichment value of data points in a sgr-file falling within each region in a reference gff3-file.
The user defines the number of data points within each region to include in the mean. The mean can be reported based on all values, the nth top values or the highest n consecutive values.
- https://doi.org/10.7554/eLife.23084.025
-
Supplementary file 1
Table showing the list of potential target genes of Atro as identified by proximity to Atro ChIP peaks.
- https://doi.org/10.7554/eLife.23084.026
-
Supplementary file 2
List of primers used for dsRNA, qPCR and in situ hybridization experiments.
- https://doi.org/10.7554/eLife.23084.027
-
Supplementary file 3
Sample size information for clonal analyses.
- https://doi.org/10.7554/eLife.23084.028