Copy-number and gene dependency analysis reveals partial copy loss of wild-type SF3B1 as a novel cancer vulnerability
Figures
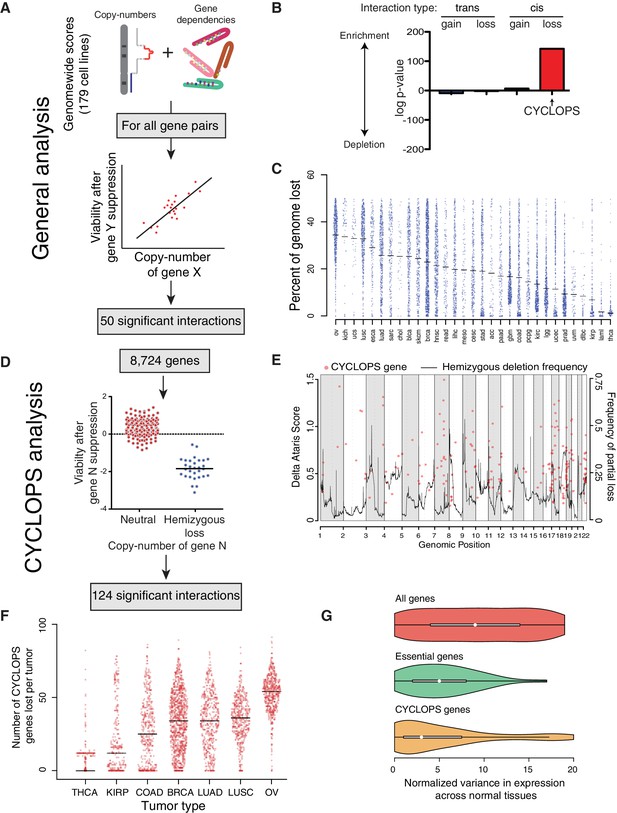
Analysis of copy-number-associated gene dependencies.
(A) Schematic describing the general analysis of copy-number-associated gene dependencies. (B) Significance of enrichment for positive copy-number:gene dependency associations across trans and cis association classes, relative to the expected share assuming all possible interactions exhibited equal likelihood of positive association. (C) Percent of genome lost across 31 TCGA cancer types. Cancer types are indicated by TCGA abbreviations (see https://tcga-data.nci.nih.gov/datareports/codeTablesReport.htm, ‘Disease Study’ table). (D) Schematic describing the approach to identify CYCLOPS genes. (E) Relative strength (delta ATARiS score) of CYCLOPS genes (orange circles; left axis) and frequency of hemizygous deletion (solid black line; right axis) against genomic position (x-axis). (F) The number of CYCLOPS genes lost per tumor for various tumor types as in (C). Horizontal black lines represent medians per tumor type. (G) Distribution of variances in gene expression for different gene classes, normalized to expression level (see Materials and methods), in normal tissues. Whiskers represent min/max values and boxes represent upper and lower quartile ranges. Width of plots represents relative sample density.
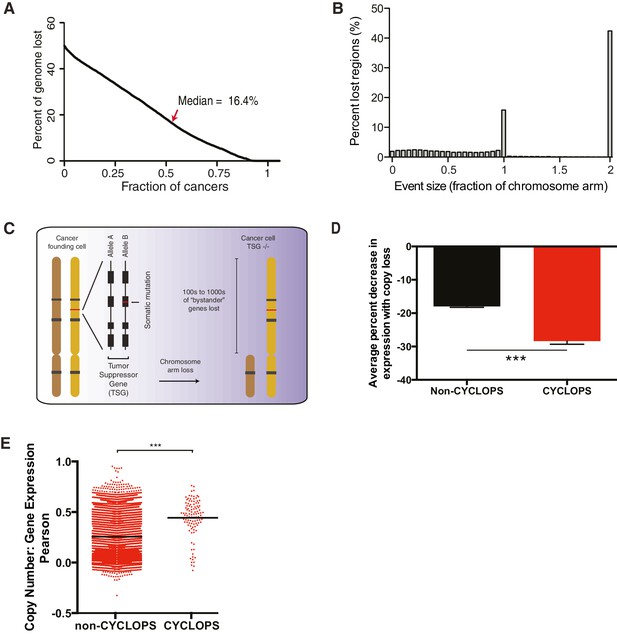
Analysis of copy-number-associated gene dependencies.
(A) Percent of genome affected by copy-loss across 10,570 cancers. (B) The fraction of the genome undergoing copy-loss as a function of the size of the copy-loss event. Fractions of a chromosome arm >1 reflect events affecting both chromosome arms; ‘2’ represents the whole chromosome. (C) Schematic for the loss of a tumor suppressor gene with focal inactivation of one allele (red line) and corresponding chromosome arm-level loss of the remaining allele. (D) Average percent decrease in gene expression upon copy-loss (y-axis) for non-CYCLOPS genes vs CYCLOPS genes across 16,867 genes and 1011 cancer cell lines. (E) Pearson correlation coefficients (y-axis) for associations between copy-number and gene expression across 16,867 genes and 1011 cancer cell lines. Red dots represent individual genes, black bars represent mean Pearson correlation coefficients for each group. For all panels: ***p<0.0001, error bars represent ± SEM.
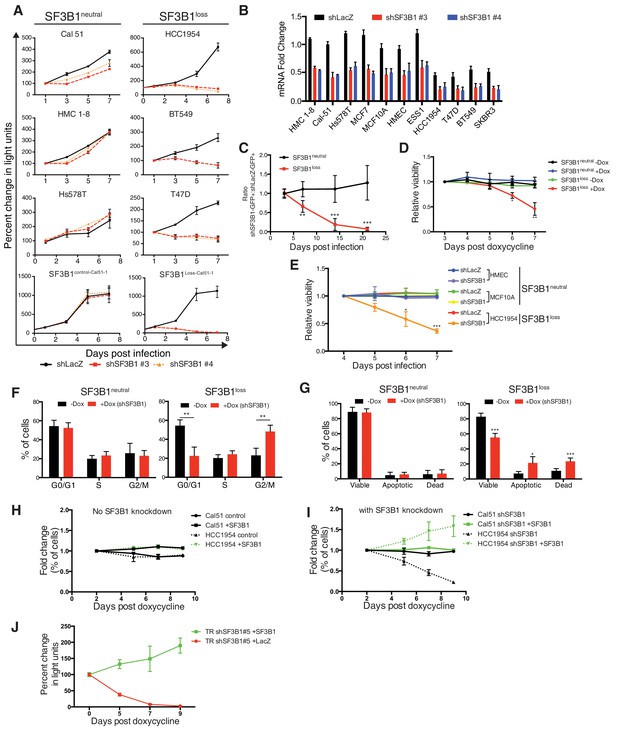
Characterization of SF3B1 as a CYCLOPS gene.
(A) Growth of breast cancer cell lines expressing shLacZ (black) or shSF3B1 (red and orange), measured as changes in CellTiterGlo luminescence relative to one day post-infection. (B) Quantitative RT-PCR of SF3B1 expression from the indicated cell lines expressing shLacZ or shSF3B1 shRNAs normalized to the diploid SF3B1neutral cell line Cal51. (C) Ratio of cells expressing shSF3B1-GFP relative to uninfected controls, normalized to the ratio of cells expressing shLacZ-GFP relative to uninfected controls. Data represent averages from SF3B1neutral (n = 7) and SF3B1loss (n = 6) cell lines, using shSF3B1 #4 in Figure 2—figure supplement 2E. (D) Viability of cells expressing TR-shSF3B1#3 and TRshSF3B1#5, relative to viability three days post doxycycline administration. (E) Viability of cells expressing shLacZ or the average of shSF3B1#3 and shSF3B1#4, measured asthe fraction of propidium iodide negative cells, relative to the viability of these cells four days post infection. (F) Cell cycle distribution four days after SF3B1 suppression averaged from TR shSF3B1#3 and #5. (G) Fraction of apoptotic cells five days after SF3B1 suppression averaged from TR shSF3B1#3 and #5, as determined by AnnexinV/PI flow cytometry. (H) Change in ratio of cells expressing SF3B1-GFP relative to uninfected cells. (I) Ratio of cells expressing SF3B1-GFP to uninfected cells, in the context of endogenous SF3B1 suppression with TR-shSF3B1 #5. (J) Growth of LacZ and SF3B1 expressing SF3B1loss cells upon SF3B1 suppression (TR-shSF3B1#5), measured as changes in CellTiter-Glo luminescence. For all panels, *p<0.05 **p<0.01 ***p<0.001, and error bars represent ± SD from at least three (panels A–G) or two (H–J) replicates.
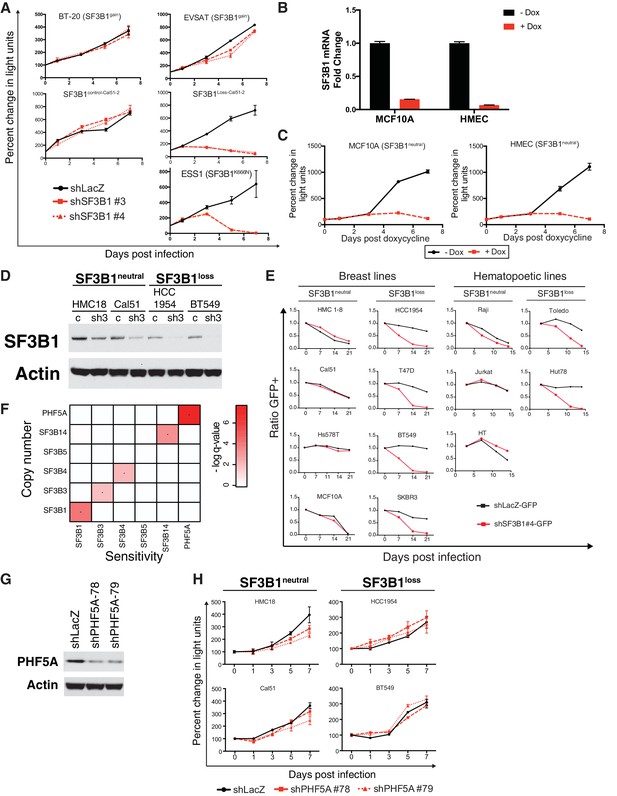
Characterization of SF3B1 as a CYCLOPS gene.
(A) Growth of SF3B1gain, SF3B1control-Cal51-2 and SF3B1Loss-Cal51-2, and SF3B1K666N mutant cells (measured as change in light units; Figure 2A) after infection with shRNAs targeting LacZ or SF3B1. (B) Quantitative RT-PCR of SF3B1 expression without (black) or with (red) doxycycline-induced shSF3B1 expression using TR-shSF3B1 #5 demonstrating greater than 85% knockdown. (C) Growth of non-transformed SF3B1neutral cell lines without (black) or with (red) doxycycline-induced shSF3B1 expression using TR-shSF3B1 #5. Growth curves are measured as changes in CellTiterGlo luminescence relative to day 0 of doxycycline treatment. (D) SF3B1 immunoblot from SF3B1neutral and SF3B1loss cells expressing shLacZ (c) or shSF3B1#3 (sh3) hairpins performed in parallel to growth assays in Figure 2A. (E) Ratio of GFP-expressing cells to uninfected controls in SF3B1neutral and SF3B1loss breast and hematopoietic cell lines expressing shLacZ-GFP (black) or shSF3B1#4-GFP (red). (F) Heatmap of False Discovery Rate q-values indicating the significance of associations between copy numbers of SF3b complex members (rows) and sensitivity of those cells to suppression of SF3b complex members by shRNA (columns). (G) PHF5A immunoblot three days after infection with shLacZ or shPHF5A targeting hairpins from HCC1954 cells profiled in panel Figure 2—figure supplement 1G. (H) Growth of breast cancer cell lines expressing shLacZ (black) or shPHF5A (red), measured as changes in CellTiterGlo luminescence relative the day of infection. For all panels, error bars represent ± SD from at least three technical replicates.
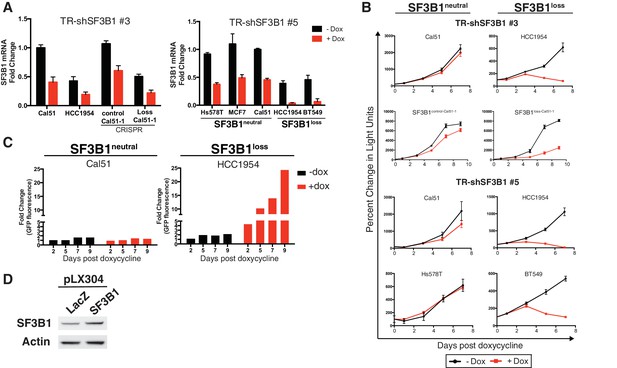
Further characterization of SF3B1 as a CYCLOPS gene.
(A) Quantitative RT-PCR of SF3B1 expression without (black) or with (red) doxycycline-induced shSF3B1 expression relative to the diploid cell line Cal 51. (B) Growth of breast cancer cell lines without (black) or with (red) doxycycline-induced shSF3B1 expression, measured as changes in CellTiterGlo luminescence relative to day 0 of doxycycline treatment. (C) GFP fluorescence from cells expressing SF3B1-IRES-GFP without (black) or with (red) doxycycline-induced TR-shSF3B1#5 expression. (D) SF3B1 immunoblot from HCC1954 cells expressing LacZ or SF3B1. For all panels, error bars represent ± SD.
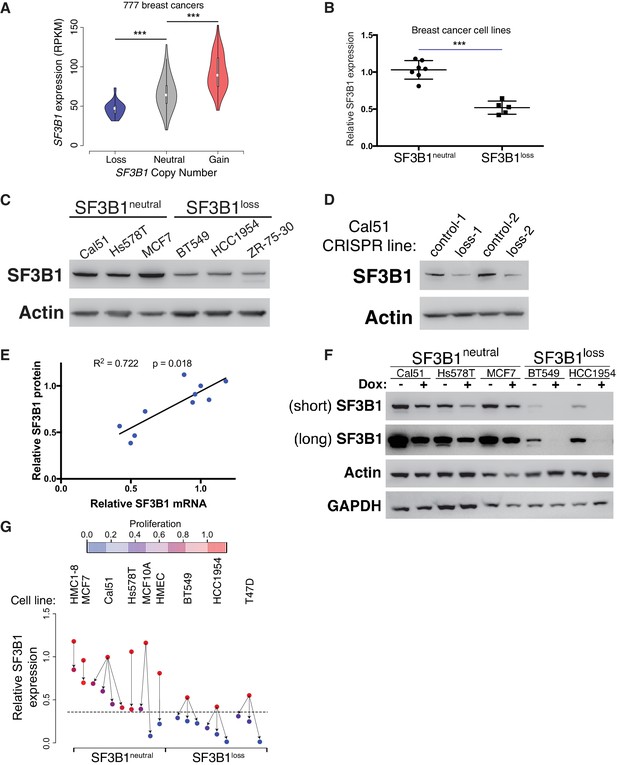
SF3B1neutral cells contain excess SF3B1 beyond the requirement for survival.
(A) SF3B1 expression from 777 TCGA breast adenocarcinomas segregated by SF3B1 copy number. Whiskers represent min/max values and boxes represent upper and lower quartile ranges. Width of plots represents relative sample density. (B) Quantitative RT-PCR of SF3B1 expression in breast cancer cell lines. Data points represent individual cell lines, horizontal lines indicate means. (C) SF3B1 protein levels in breast cancer cell lines by immunoblot. (D) SF3B1 immunoblot from control cells and those with single-copy SF3B1 inactivation by CRISPR. (E) Scatterplot of SF3B1 mRNA and protein expression relative to diploid cell line Cal51 after normalization to actin in a panel of breast cancer cell lines (p=0.0018, R2 = 0.772, regression line slope = 0.789). (F) SF3B1 immunoblot from SF3B1neutral and SF3B1loss cells 4 days after TR-shSF3B1#5 induction by doxycycline. (G) Differences in proliferation 7 days after SF3B1 suppression (per CellTiter-Glo, see Appendix Methods; red=high, blue=low), against the relative level of SF3B1 expression (assessed by qPCR; y-axis) in SF3B1neutral (left) or SF3B1loss (right) cells expressing either shLacZ (origins of arrows) or shSF3B1 (ends of arrows). Origins with multiple arrows represent cell lines subject to more than one SF3B1 shRNA. Each data point represents the mean from at least two replicate experiments. The dashed line represents the estimated minimum threshold of SF3B1 expression required for survival. For all panels, *p<0.05 **p<0.01 ***p<0.001, and error bars represent ± SD.
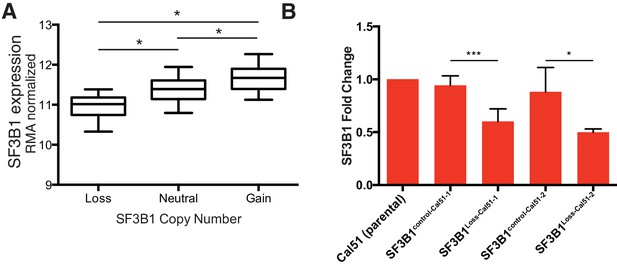
Further characterization of SF3B1 as a CYCLOPS gene.
(A) SF3B1 expression from 974 cell lines from the CCLE classified by SF3B1 copy-number status. Boxes represent the upper and lower quartiles, whiskers represent the 5–95th percentiles. *p<0.0001. (B) SF3B1 mRNA expression from control cells and those with CRISPR-induced copy-loss. *p<0.05 and ***p<0.001.
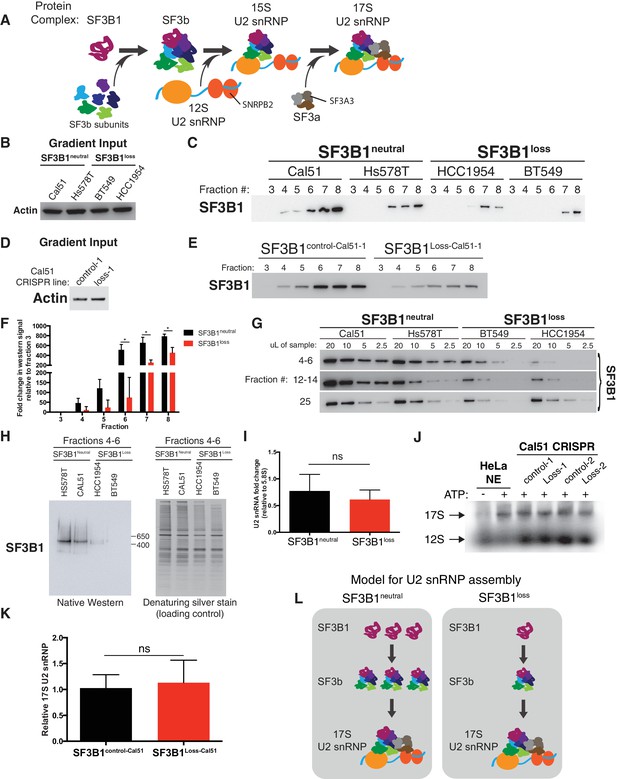
SF3B1 copy-loss selectively reduces the abundance of the SF3b complex.
(A) Diagram of U2 snRNP assembly. (B–C) Glycerol gradient fractionation of native whole-cell lysates probed by western blot in breast cancer cell lines and (D–E) isogenic cells generated by CRISPR. (F) Quantification of SF3B1 immunoblots from glycerol gradient fractions 3–8, relative to fraction 3 (n = 3 for each group, see Appendix Methods). (G) Serial dilution of pooled glycerol gradient fractions probed for SF3B1 by immunoblot. (H) (left) SF3B1 Native PAGE immunoblot of pooled glycerol gradient fractions. (right) denaturing silver stain of total protein from the same pooled fractions as loading control. (I) Quantitative RT-PCR for U2 snRNA expression in three SF3B1neutral and three SF3B1loss breast cancer cell lines. (J) Native agarose gel of U2 snRNP complexes visualized with radiolabeled 2’ O-methyl oligonucleotides complementary to the U2 snRNA. Nuclear extracts were generated from SF3B1control-Cal51 and SF3B1Loss-Cal51 cells. HeLa cell nuclear extracts (NE) ± ATP were used as controls. Representative image from triplicate experiments. (K) Densitometric quantification of 17S U2 snRNP bands in (J) presented as fold change relative to SF3B1control-Cal51 cells. Data are from three replicate experiments. (L) Model for changes to U2 snRNP assembly associated with SF3B1 copy-loss. For all panels, *p<0.05, and error bars represent ± SD, ns = not significant.
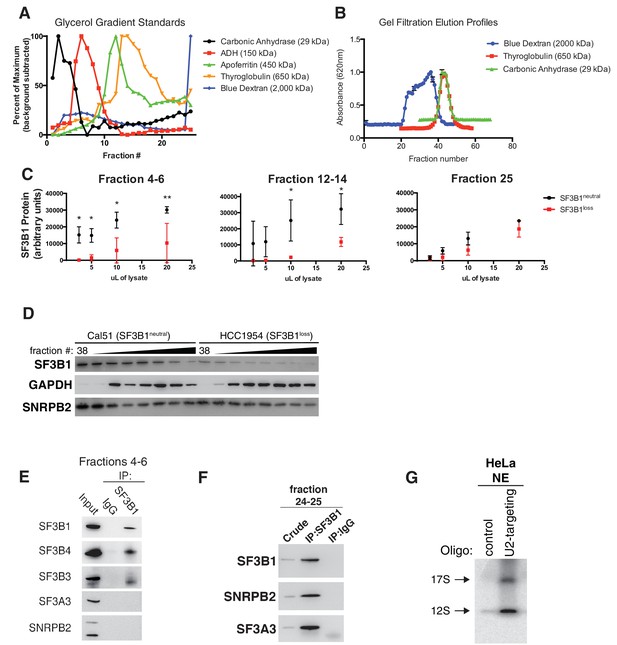
SF3B1 copy-loss selectively reduces the abundance of the SF3b complex.
(A) Sedimentation of mass standards in 10–30% glycerol gradients. (B) Elution profiles of mass standards in gel filtration chromatography columns. (C) Quantification of SF3B1 immunoblots from serial dilutions of glycerol gradient fractions in Figure 4G. SF3B1 abundance normalized by actin loading controls and calculated by densitometry using ImageJ (see Appendix Methods). SF3B1neutral and SF3B1loss cells (n = 2 each) quantified from western blot images performed in triplicate. Dots represent mean and error bars are ± SD. (D) Immunoblot of indicated gel filtration fractions. GAPDH and SNRPB2 represent markers for complexes <700 kDa and spliceosome precursors respectively. (E) Immunoblot after SF3B1 immunoprecipitation from pooled glycerol gradient fractions 4–6. (F) Immunoblot after SF3B1 immunoprecipitation from pooled glycerol gradient fractions 24–25. (G) Characterization of U2 oligo specificity. Control HeLa nuclear extracts (NE) were incubated with either U2 snRNA targeting oligonucleotides or control oligonucleotides. For all panels, *p<0.05, **p<0.01 and ***p<0.001.
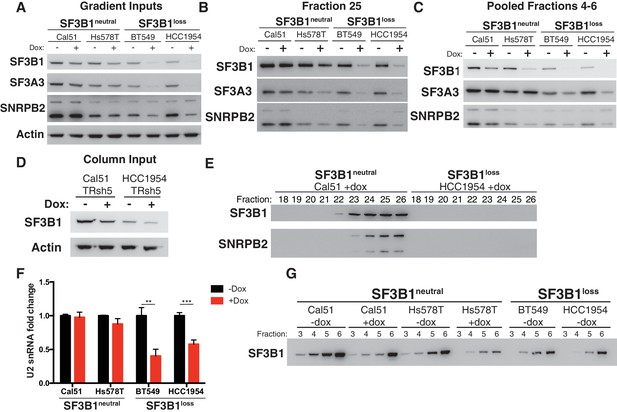
Reduced spliceosome precursors and U2 snRNP abundance upon SF3B1 suppression in SF3B1loss cells.
(A) Western immunoblots without and with SF3B1 suppression prior to glycerol gradient fractionation. (B) Western immunoblots from glycerol gradient fraction 25 (protein complexes >650 kDa). (C) Western immunoblots from pooled glycerol gradient fractions 4–6 (protein complexes ~150–450 kDa). (D) Immunoblot from lysates prior to gel filtration chromatography. (E) Immunoblot of gel filtration fractions 18–26 (protein complexes >650 kDa) from lysates with SF3B1 suppression. (F) Quantitative RT-PCR for U2 snRNA expression without and with SF3B1 suppression. (G) Glycerol gradient fractions from SF3B1neutral cells without and with SF3B1 suppression compared to SF3B1loss without suppression. For all panels, TR-shSF3B1#5 was used. *p<0.05 **p<0.01 ***p<0.001.
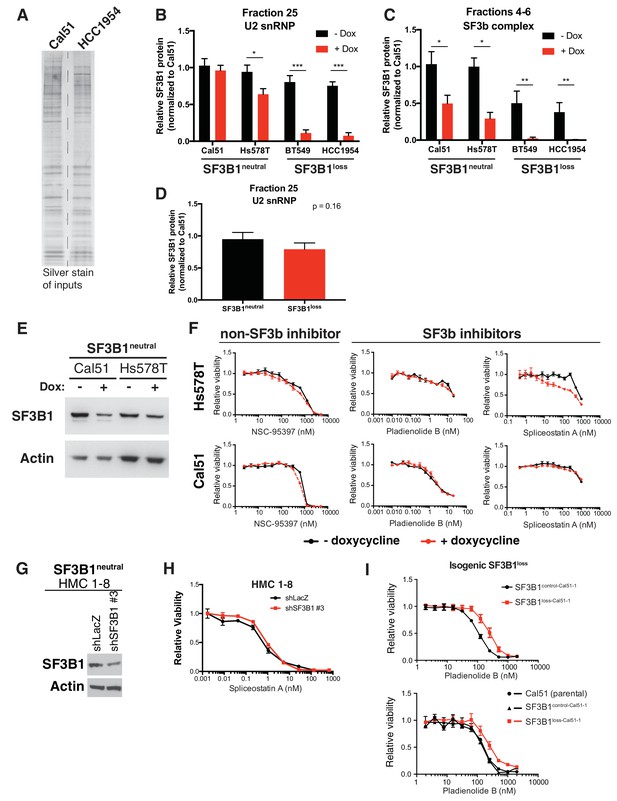
Reduced spliceosome precursors and U2 snRNP abundance upon SF3B1 suppression in SF3B1loss cells.
(A) Silver stain of gel filtration inputs for Figure 5E. (B) Quantification of SF3B1 immunoblots from glycerol gradient fraction 25 in Figure 5B, relative to Cal51 (n = 3 for each cell line, see Appendix Methods). (C) Quantification of SF3B1 immunoblots from glycerol gradient fractions 4–6 in Figure 5C, relative to Cal51 (n = 3 for each cell line, see Appendix Methods). (D) Quantification of SF3B1 immunoblots of only the minus doxycycline treated lanes from glycerol gradient fraction 25 in Figure 5B, relative to Cal51. (n = 2 cell lines per group averaged from three technical replicates per cell line). (E) SF3B1 immunoblot from cells used in panel F (with TR-shSF3B1#5). (F) Drug sensitivity curves for indicated splicing modulators in cells without and with SF3B1 suppression (TR-shSF3B1#5). (G) SF3B1 immunoblot from HMC1–8 cells stably expressing shLacZ or shSF3B1 #3 used in panel H. (H) Spliceostatin A drug sensitivity curve for SF3B1neutral HMC1–8 cells stably expressing shLacZ or shSF3B1 #3 hairpins. (I) Pladienolide B drug sensitivity curves in SF3B1loss-Cal51 cells and SF3B1control-Cal51 or parental Cal51 cells. For all panels, *p<0.05 **p<0.01 ***p<0.001.
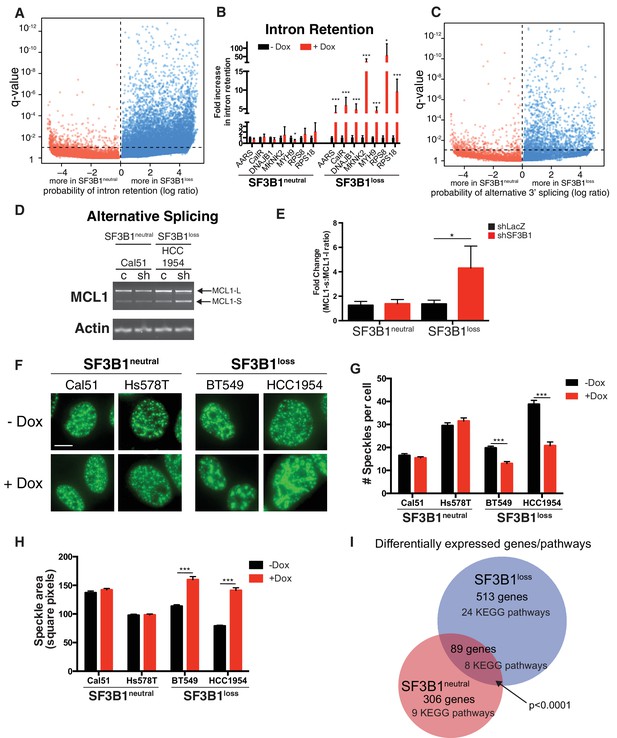
Partial SF3B1 suppression results in splicing defects in SF3B1loss cells.
(A) Statistical significance of intron retention (see Materials and methods) across all exon-intron junctions (dots) in SF3B1neutral (red) and SF3B1loss cells (blue) after SF3B1 suppression. The horizontal dashed line represents the significance threshold (q < 0.01) and the vertical dashed line segregates intron-exon junctions more likely to be altered in SF3B1neutral (left) or SF3B1loss cells (right). (B) qPCR for a single intron within the indicated gene without and with shSF3B1 induction by doxycycline (SF3B1neutral n = 3, SF3B1loss n = 3, averaged from TR-shSF3B1#3 and TRshSF3B1#5. SF3B1control-Cal51 and SF3B1Loss-Cal51 n = 2 each, averaged from TR-shSF3B1#3). (C) Statistical significance of alternative 3’ splice site selection (see Materials and methods) across 3’ splice junctions (dots) in SF3B1neutral (red) and SF3B1loss cells (blue) after SF3B1 suppression (as in panel A). (D) Representative RT-PCR from SF3B1neutral and SF3B1loss cells after SF3B1 knockdown. ‘c’ represents LacZ control hairpins, ‘sh’ represents shSF3B1#4. Arrows represent product sizes for MCL-L and MCL-S. (E) Densitometric quantification of the ratio of MCL1-S:MCL1-L, relative to shLacZ-expressing controls (mean ± SD, n = 3 per group averaged from shSF3B1#3, and #4). (F) Immunofluorescent images of nuclear speckles by anti-SC35 (SRSF2) staining. Scale bar = 5 uM. (G) Quantification of number of nuclear speckles and (H) speckle area per cell across at least 100 nuclei. For (F–H) TRshSF3B1#5 was used. (I) Number of differentially expressed genes upon SF3B1 suppression (q < 0.1) and the number of enriched KEGG pathways amongst indicated gene set (q < 0.05). For all panels, *p<0.05 **p<0.01 ***p<0.001.
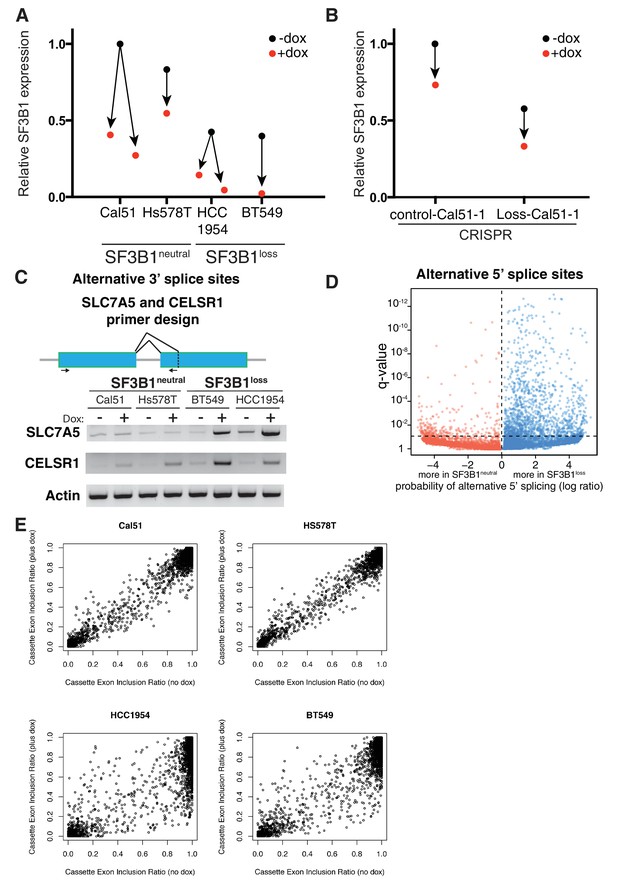
Partial SF3B1 suppression results in splicing defects in SF3B1loss cells.
(A) Relative level of SF3B1 expression (assessed by quantitative PCR; y-axis) in SF3B1neutral (left) or SF3B1loss (right) cells without doxycycline (black dots, origins of arrows) or with doxycycline (red dots, ends of arrows). Origins with one arrow represents TR-shSF3B1#3, origins with multiple arrows represent cell lines with TR-shSF3B1#3 and TR-shSF3B1#5 done in parallel. Each data point represents the mean from at least two replicate experiments. (B) Relative level of SF3B1 expression in SF3B1control-Cal51-1 and SF3B1Loss-Cal51-1 with TR-shSF3B1#3 presented as described in (A). (C) Schematic of primers designed to detect alternative 3’ splice sites in SLC7A5 and CELSR1 and representative RT-PCR results from SF3B1 suppression using TR-shSF3B1#5 in SF3B1neutral and SF3B1loss cells (n = 2 per group). (D) Statistical significance of 5’ splice site junctions across all 5’ splice junctions (dots) in SF3B1neutral (red) and SF3B1loss cells (blue) after SF3B1 suppression. The horizontal dashed line represents the significance threshold (q < 0.01) and the vertical dashed line segregates 5’ splice junctions more likely to be altered in SF3B1neutral (left) or SF3B1loss cells (right). Alternative 5’ splice site selection occurs more frequently in SF3B1loss cells after SF3B1 suppression (p=9×10−165). (E) Scatter plots for ratio of RNA sequencing reads that include a cassette exon without SF3B1 suppression (no doxycycline, x-axis), or with SF3B1 suppression (plus doxycycline, y-axis). Each dot represents a single cassette exon in SF3B1neutral cells (Cal51, Hs587T) and SF3B1loss cells.
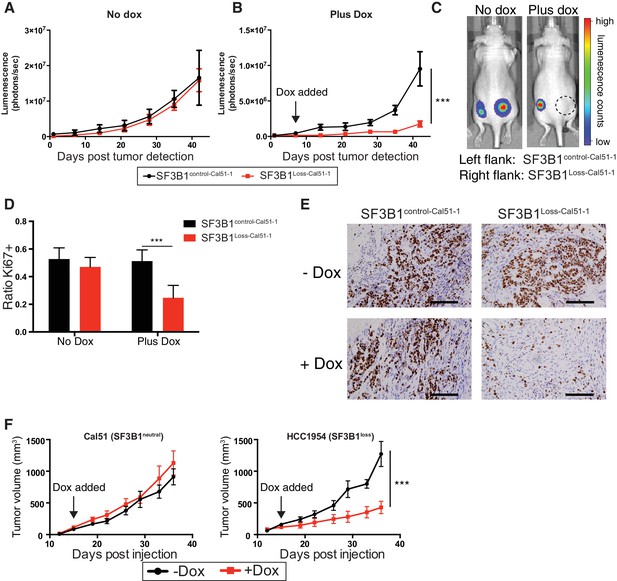
SF3B1 suppression inhibits tumor growth in vivo.
Luminescent quantification of xenograft growth from SF3B1control-Cal51-1 (black) and SF3B1Loss-Cal51-1 (red) tumors (A) without doxycycline (n = 4) and (B) with doxycycline (n = 17) using TR-shSF3B1 #3. (C) Representative animal images overlaid with heat maps from bioluminescent tumor detection. Dashed circle represents region where established tumor was detected prior to doxycycline treatment. (D) Quantification of Ki67+ cells from xenografts 42 days post tumor detection using CellProfiler ≥ 2440 nuclei were scored for each tumor, ≥ 3 tumors per group (see Materials and methods). (E) Representative Ki67 immunohistochemistry images of xenografts quantified in panel (D). (F) Growth of established tumors for Cal51 and HCC1954 xenografts without doxycycline (black) or with doxycycline (red) using TR-shSF3B1 #3. For Cal51 and HCC1954 no doxycycline groups (n = 13); plus doxycycline groups (n = 12). For all panels, ***p≤0.001.
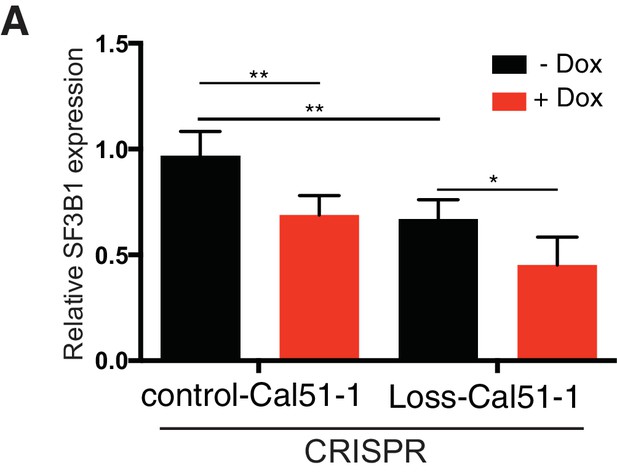
SF3B1 suppression inhibits tumor growth in vivo.
(A) Quantitative RT-PCR for SF3B1 expression from xenograft tumors without (black) or with (red) doxycycline-induced TR-shSF3B1#3 expression (n≥4 for each group).
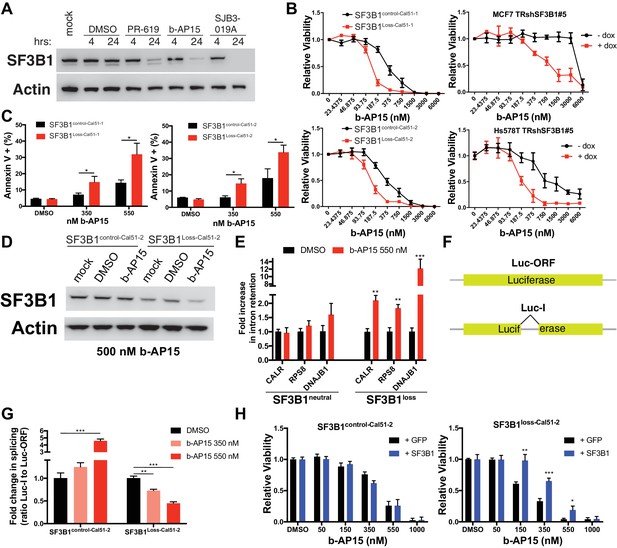
Deubiquitinase inhibitor b-AP15 can induce SF3B1 degradation and selectively kill SF3B1loss cells.
(A) SF3B1 immunoblot after DUBi treatment of the SF3B1neutral cell line, Cal51, at the indicated time points. (B) b-AP15 dose response curves in isogenic cell contexts. SF3B1control-Cal51 and SF3B1Loss-Cal51 or SF3B1neutral cells with or without doxycycline expressing TR-shSF3B1 #5, to phenocopy the reduced SF3B1 expression observed in SF3B1loss cells, were assayed 48 hr after b-AP15 treatment. (C) Percent of Annexin V positive cells 24 hr after b-AP15 treatment in SF3B1control-Cal51 and SF3B1Loss-Cal51 cells. (D) SF3B1 immunoblot 48 hr after treatment with 500 nM b-AP15 in SF3B1control-Cal51-2 and SF3B1Loss-Cal51-2 cells. (E) qPCR for a single intron within the indicated gene with 550 nM b-AP15 or DMSO control (SF3B1neutral n = 2, SF3B1loss n = 2, averaged from SF3B1control-Cal51 and SF3B1Loss-Cal51 cells). (F) Schematic of luciferase reporter constructs used in (G). Yellow rectangles represent luciferase protein coding regions. Angled black line represents the location of the intron. (G) Fold change in luciferase splicing reporter signal after b-AP15 treatment. Data represented as the ratio of spliced luciferase signal (Luc-I) to luciferase ORF signal (Luc-ORF) relative to their respective DMSO luciferase signal. DMSO treatment (black), 350 nM b-AP15 (light red) and 550 nM b–AP15 (dark red). (H) b-AP15 dose response in SF3B1control-Cal51-2 and SF3B1Loss-Cal51-2 cells expressing GFP (black) or SF3B1 (blue). For all panels, *p<0.05 **p<0.01 ***p<0.001.
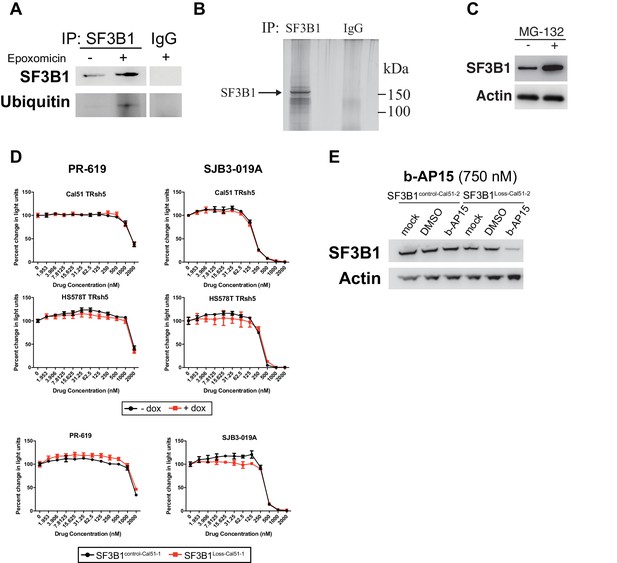
Deubiquitinase inhibitor b-AP15 can induce SF3B1 degradation and selectively kill SF3B1loss cells.
(A) SF3B1 immunoprecipitation (IP) under denaturing conditions in the presence of proteasome inhibitor epoxomicin (10 uM) to increase ubiquitinated proteins. (B) Silver stain of IP eluate from high stringency conditions in (A) to demonstrate the enrichment of SF3B1. (C) SF3B1 immunoblot from SF3B1neutral Cal51 cells 16 hr after treatment with the proteasome inhibitor MG-132 (10 uM). (D) Cell titer glo dose response curves for pan-DUBi PR-619 and the more selective DUBi SJB-019A in SF3B1control-Cal51-1 and SF3B1Loss-Cal51-1 cells. Additional isogenic cell line pairs were generated with or without partial SF3B1 suppression by TRshSF3B1#5 in SF3B1neutral cells. (E) SF3B1 immunoblot in SF3B1control-Cal51-2 and SF3B1Loss-Cal51-2 cells 24 hr after treatment with 750 nM b-AP15.
Additional files
-
Supplementary file 1
Supplementary tables.
(A) Results of copy-number associated dependencies. (B) Results of CYCLOPS gene analysis. (C) Frequency of genetic SF3B1 alterations in cancer. (D) KEGG Pathways Enriched from significant candidate CYCLOPS genes. (E) Significantly differentially expressed genes after SF3B1 suppression in SF3B1neutral and SF3B1loss cells. (F) Summary of KEGG pathway enrichment from differentially expressed genes in each class (SF3B1loss or SF3B1neutral) from genes in Supplementary file E. (G) Significantly differentially expressed genes after enhanced SF3B1 expression. (H) PCR primer sequences used in this study.
- https://doi.org/10.7554/eLife.23268.019