A sharp Pif1-dependent threshold separates DNA double-strand breaks from critically short telomeres
Figures
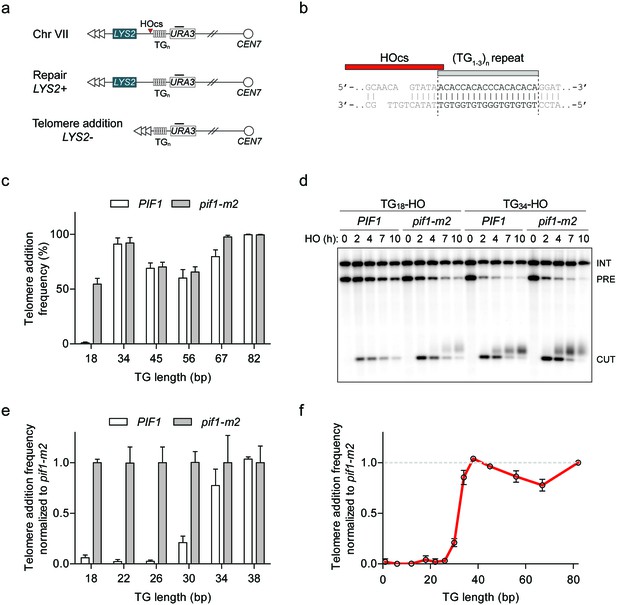
Characterization of Pif1 activity at DNA ends reveals a DSB-telomere transition.
(a) Schematic of a system to study the fate of DNA ends. Telomeric repeats are placed adjacent to an HO cut site (HOcs) at the ADH4 locus on Chr VII. Telomere addition can be measured using a genetic assay based on the loss of the distal LYS2 gene as measured by resistance to α-aminoadipic acid. Southern blotting with a probe complementary to URA3 (black bar) allows for visualization of DNA end stability. (b) Sequence of the TG18 substrate and the overhang produced by the HO endonuclease. The C-rich strand runs 5’ to 3’ toward the centromere and is resected following DSB induction to expose a 3’ G-rich overhang. (c) Telomere addition frequency at DNA ends containing 18–82 bp of TG sequence. Data represent the mean ± s.d. from a minimum of n = 3 independent experiments. See Supplementary file 1a for the sequences of all DNA ends and source data are found in Figure 1—source data 1. (d) Southern blot of DNA ends containing TG18 and TG34 ends in wild-type and pif1-m2 cells following HO induction. A URA3 probe was used to label the ura3-52 internal control (INT) and the URA3 gene adjacent to the TGn-HO insert (PRE) which is cleaved by HO endonuclease (CUT). Newly added telomeres are visualized as a heterogeneous smear above the CUT band. (e) Telomere addition frequency normalized to pif1-m2 cells at DNA ends containing 18–38 bp of TG sequence. Data represent the mean ± s.d. from n = 3 independent experiments. Source data are found in Figure 1—source data 2. (f) Summary of telomere addition frequency normalized to pif1-m2 across the spectrum of TG repeat substrates. Source data are found in Figure 1—source data 3.
-
Figure 1—source data 1
Raw data for telomere addition assays shown in Figure 1c.
- https://doi.org/10.7554/eLife.23783.004
-
Figure 1—source data 2
Raw data for telomere addition assays shown in Figure 1e.
- https://doi.org/10.7554/eLife.23783.005
-
Figure 1—source data 3
Raw data telomere addition assays shown in Figure 1f.
- https://doi.org/10.7554/eLife.23783.006
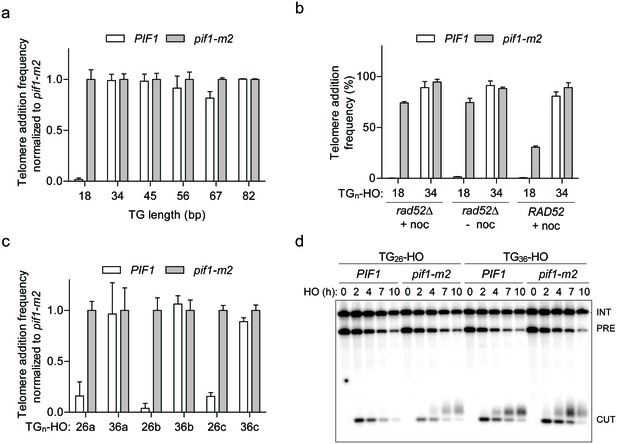
Characterizing a threshold of Pif1 activity at DNA ends.
(a) Telomere addition frequency normalized to pif1-m2 cells at DNA ends containing 18–82 bp of (TG1-3)n sequence. Data represent the mean ± s.d. from n = 3 independent experiments. Source data are found in Figure 1—figure supplement 1—source data 1. (b) Telomere addition frequency in rad52Δ cells synchronized with 15 µg/mL nocodazole for 2 hr (+noc), asynchronously growing cells (- noc), and in cells harboring a pRS415-RAD52 plasmid. Data represent the mean ± s.d. from n = 3 independent experiments. Source data are found in Figure 1—figure supplement 1—source data 2. (c) Telomere addition frequency normalized to pif1-m2 cells at DNA ends containing 26 bp or 36 bp versions of three different natural telomeric (TG1-3)n sequences. Data represent the mean ± s.d. from n = 3 independent experiments. Source data are found in Figure 1—figure supplement 1—source data 3. (d) Southern blot of DNA ends containing the TG26b and TG36b ends in wild-type (WT) and pif1-m2 cells following HO induction. A URA3 probe was used to label the ura3-52 internal control (INT) and the URA3 gene adjacent to the TGn-HO insert (PRE) which is cleaved by HO endonuclease (CUT).
-
Figure 1—figure supplement 1—source data 1
Raw data telomere addition assays shown in Figure 1—figure supplement 1a.
- https://doi.org/10.7554/eLife.23783.007
-
Figure 1—figure supplement 1—source data 2
Raw data for telomere addition assays shown in Figure 1—figure supplement 1b.
- https://doi.org/10.7554/eLife.23783.008
-
Figure 1—figure supplement 1—source data 3
Raw data for telomere addition assays shown in Figure 1—figure supplement 1c.
- https://doi.org/10.7554/eLife.23783.009
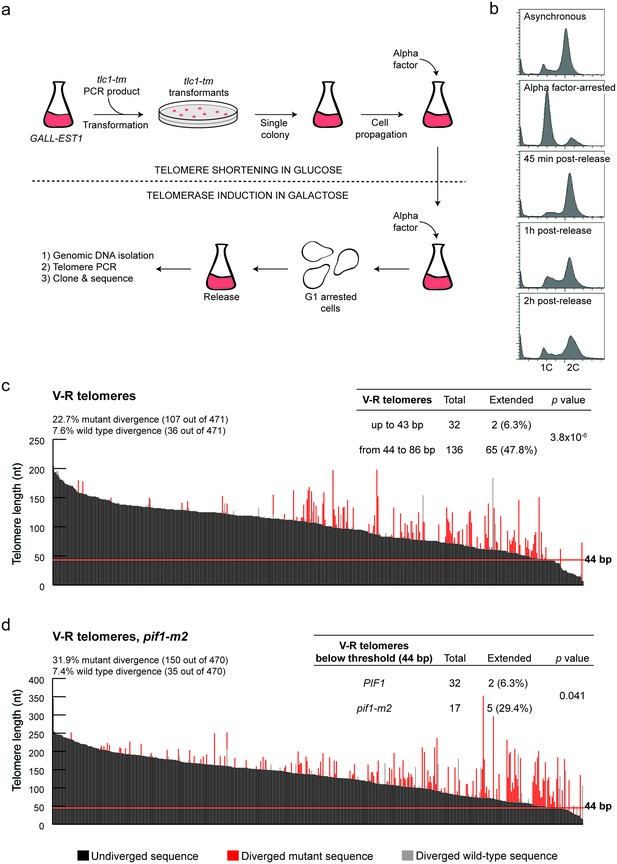
Characterization of the DSB-telomere transition at chromosome ends.
(a) Methodology of the iSTEX assay; see text for details. (b) Arrest and release efficiency monitored by flow cytometry. (c) Telomere V-R was amplified, cloned and sequenced after 2 hr of tlc1-tm telomerase induction. Each bar represents an individual telomere. The black portion of each bar represents the undivergent sequence, the red portion shows the mutant divergent sequence and the grey portion indicates the wild-type divergent sequence. Telomeres are sorted based on the length of the undiverged sequence (black portion). The horizontal red line indicates the threshold below which telomerase-mediated telomere extension becomes very inefficient. Statistical analysis was done using a Fisher’s exact test and for this analysis, telomeres containing only wild-type divergence were excluded. Source data are found in Figure 2—source data 1. (d) As in panel (c) except in a pif1-m2 background. Statistical analysis comparing extension of telomeres below 44 bp in length in wild type and pif1-m2 was done using a Fisher’s exact test. Source data are found in Figure 2—source data 2.
-
Figure 2—source data 1
Raw data for iSTEX shown in Figure 2c.
- https://doi.org/10.7554/eLife.23783.012
-
Figure 2—source data 2
Raw data for iSTEX shown in Figure 2d.
- https://doi.org/10.7554/eLife.23783.013
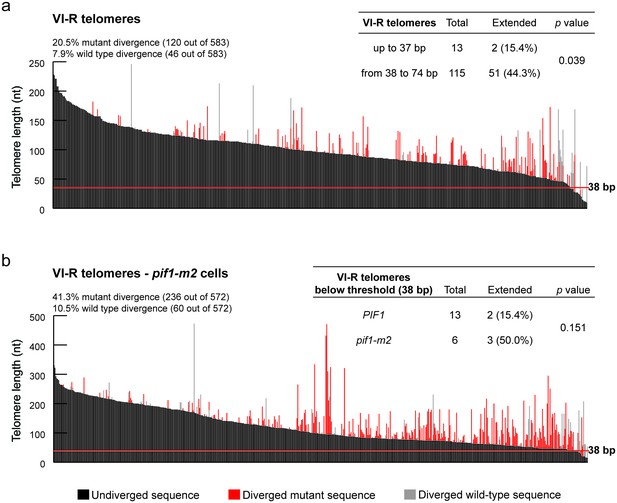
Characterization of the DSB-telomere transition at telomere VI-R.
(a) Telomere VI-R was amplified, cloned and sequenced after 2 hr of tlc1-tm telomerase induction. Each bar represents an individual telomere. The black portion of each bar represents the undivergent sequence, the red portion shows the mutant divergent sequence and the grey portion indicates the wild-type divergent sequence. Telomeres are sorted based on the length of the undiverged sequence (black portion). The red line indicates the threshold below which telomerase-mediated telomere extension becomes very inefficient. Statistical analysis was done using a Fisher’s exact test and for this analysis, telomeres containing only wild-type divergence were excluded. Source data are found in Figure 2—figure supplement 1—source data 1. (b) As in panel (a) except in a pif1-m2 background. Statistical analysis comparing extension of telomeres below 38 bp in length between in wild type and pif1-m2 was done using a Fisher’s exact test. Figure 2—figure supplement 1—source data 2.
-
Figure 2—figure supplement 1—source data 1
Raw data for iSTEX shown in Figure 2—figure supplement 1a.
- https://doi.org/10.7554/eLife.23783.014
-
Figure 2—figure supplement 1—source data 2
Raw data for iSTEX shown in Figure 2—figure supplement 1b.
- https://doi.org/10.7554/eLife.23783.015
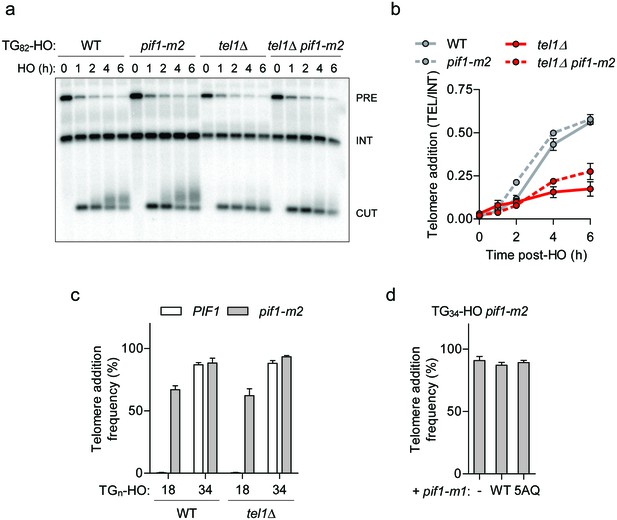
Pif1 is not inactivated by Tel1 at short telomeres.
(a, b) Southern blot of the TG82 DNA end following HO-induction in wild-type (WT) and pif1-m2 cells without or with a TEL1 deletion. An ADE2 probe was used to label the ade2Δ1 internal control (INT) and the ADE2 gene adjacent to the TGn-HO insert (PRE) which is cleaved by HO endonuclease (CUT). Quantification of the newly added telomere signal (b) calculated by subtracting the background signal present prior to HO-induction and by normalizing to the INT control. Data represent the mean ± s.d. from n = 2 independent experiments. Source data are found in Figure 3—source data 1. (c) Telomere addition frequency at the TG18 and TG34 DNA ends in tel1Δ mutants. Data represent the mean ± s.d. from n = 3 independent experiments. Source data are found in Figure 3—source data 2. (d) Telomere addition frequency at the TG34 DNA end in pif1-m2 cells (-) and cells expressing a wild-type (WT) or pif1-5AQ (S148A, S180A, T206A, S707A, T811A) nuclear-specific pif1-m1 allele. Data represent the mean ± s.d. from n = 3 independent experiments. The functionality of the pif1-m1 alleles was assessed by rescue of the telomere elongation associated with pif1-m2 (Figure 3—figure supplement 1e). Source data are found in Figure 3—source data 3.
-
Figure 3—source data 1
Raw data for telomere addition assays shown in Figure 3b.
- https://doi.org/10.7554/eLife.23783.018
-
Figure 3—source data 2
Raw data for telomere addition assays shown in Figure 3c.
- https://doi.org/10.7554/eLife.23783.019
-
Figure 3—source data 3
Raw data for telomere addition assays shown in Figure 3d.
- https://doi.org/10.7554/eLife.23783.020
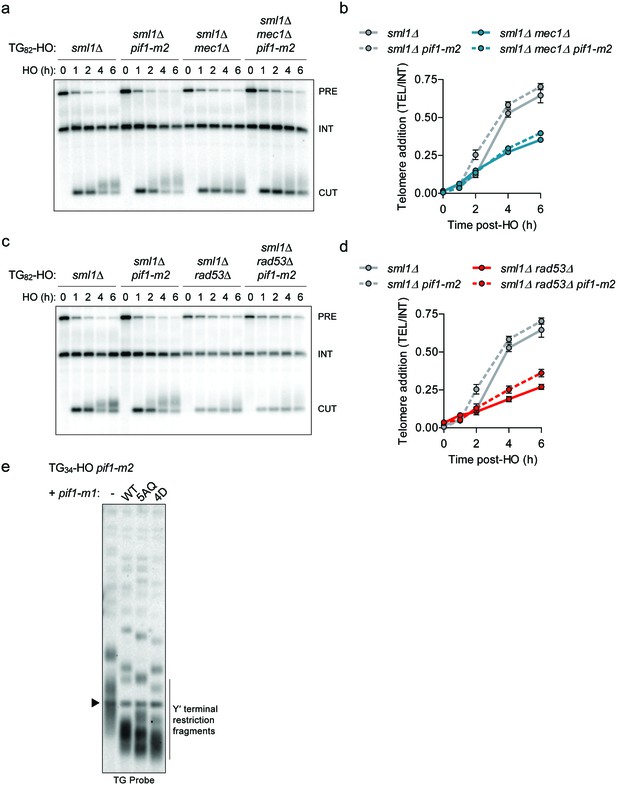
Loss of MEC1 or RAD53 does not affect Pif1 at short telomeres.
(a-–d) Southern blot of the TG82 DNA end following HO induction in sml1Δ and sml1Δ pif1-m2 cells combined with the deletion of MEC1 (a) or RAD53 (c). SML1 was deleted to suppress the lethality of mec1Δ and rad53Δ. An ADE2 probe was used to label the ade2Δ1 internal control (INT) and the ADE2 gene adjacent to the TGn-HO insert (PRE) which is cleaved by HO endonuclease (CUT). Quantification of the newly added telomere signal in mec1Δ cells (b) from n = 1 experiment, and rad53Δ cells (d) from n = 2 independent experiments. Data represent the mean ± s.d. The data for sml1∆ and sml1∆ pif1-m2 (n = 3) are identical in panels (b) and (d). (e) Telomere Southern blot of pif1-m2 strains carrying a vector control (-) or a plasmid expressing either pif1-m1 (WT), pif1-5AQ (5AQ), or pif1-4D (4D). Each sample is loaded with a digested plasmid containing telomeric sequences to give the 1.8 kb band (indicated by an arrow). Source data for panel b can be found in Figure 3—figure supplement 1—source data 1 and for panel d in Figure 3—figure supplement 1—source data 2.
-
Figure 3—figure supplement 1—source data 1
Raw data for telomere addition assays shown in Figure 3—figure supplement 1b.
- https://doi.org/10.7554/eLife.23783.021
-
Figure 3—figure supplement 1—source data 2
Raw data for telomere addition assays shown in Figure 3—figure supplement 1d.
- https://doi.org/10.7554/eLife.23783.022
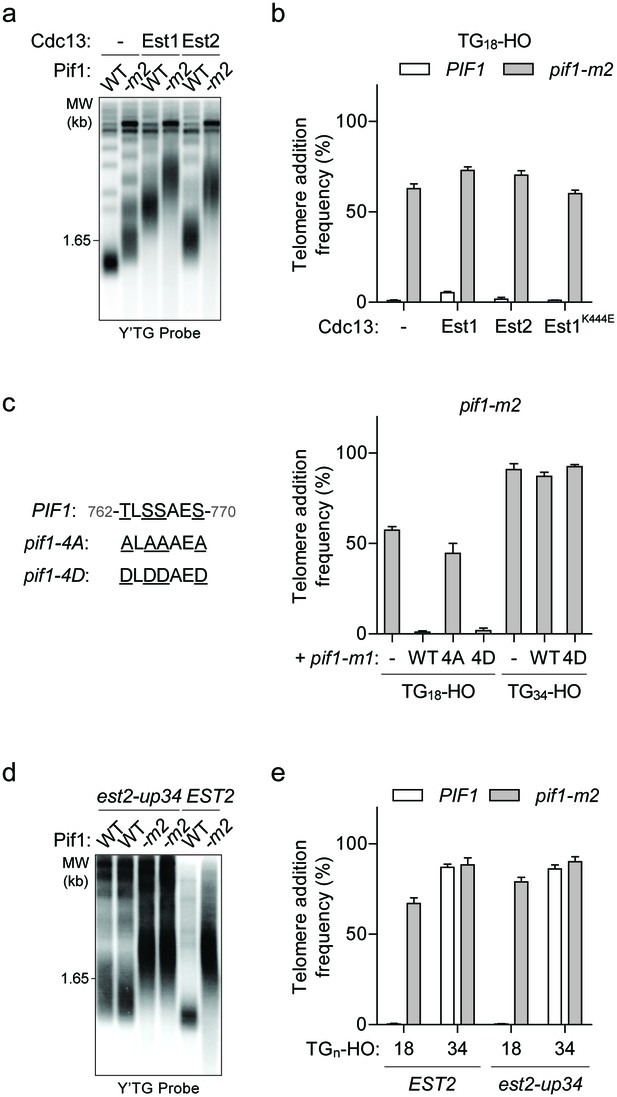
The DSB-telomere transition recapitulates the differential regulation of Pif1.
(a) Southern blot for telomere length in TG18-HO wild-type (WT) and pif1-m2 cells harbouring an empty plasmid (-) or expressing plasmid-based Cdc13-Est1 or Cdc13-Est2 fusions. Cells were passaged for approximately 75 generations before genomic DNA extraction. A Y’ TG probe was used to label telomeric sequences. (b) Telomere addition frequency of the cells described in panel (a) and est1Δ strains expressing a cdc13-est1-60 (K444E) fusion. Data represent the mean ± s.d. from n = 3 independent experiments. Source data are found in Figure 4—source data 1. (c) Telomere addition frequency at the TG18 and TG34 DNA ends in pif1-m2 cells (-) and cells expressing a wild-type (WT), pif1-4A (T763A/S765A/S766A/S769A), or pif1-4D (T763A/S765A/S766A/S769A) nuclear-specific pif1-m1 allele. Data represent the mean ± s.d. from n = 3 independent experiments. Source data are found in Figure 4—source data 2. (d) Southern blot for telomere length in PIF1 (WT) and pif1-m2 cells combined with or without the est2-up34 mutation. Cells were passaged for approximately 75 generations before genomic DNA extraction and a Y’ TG probe was used to label telomere sequences. (e) Telomere addition frequency at the TG18 and TG34 DNA ends in PIF1 and pif1-m2 cells with or without the est2-up34 mutation. Data represent the mean ± s.d. from n = 3 independent experiments. The EST2 data are the same as the WT data shown in Figure 3c. Source data are found in Figure 4—source data 3.
-
Figure 4—source data 1
Raw data for telomere addition assays shown in Figure 4b.
- https://doi.org/10.7554/eLife.23783.024
-
Figure 4—source data 2
Raw data for telomere addition assays shown in Figure 4c.
- https://doi.org/10.7554/eLife.23783.025
-
Figure 4—source data 3
Raw data for telomere addition assays shown in Figure 4e.
- https://doi.org/10.7554/eLife.23783.026
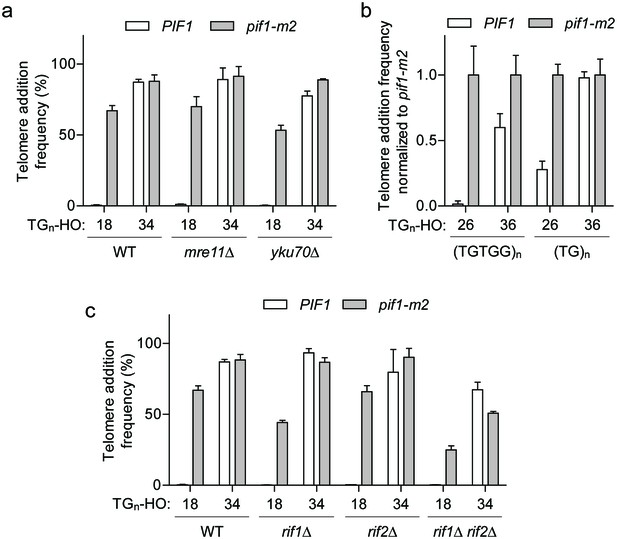
The DSB-telomere transition does not require Rap1.
(a) Telomere addition frequency at the TG18 and TG34 DNA ends in mre11Δ and yku70Δ mutants. Data represent the mean ± s.d. from n = 3 independent experiments. Source data are found in Figure 5—source data 1. (b) Telomere addition frequency normalized to pif1-m2 cells at DNA ends containing 26 bp or 36 bp of either (TGTGG)n or (TG)n repeats. Data represent the mean ± s.d. from n = 3 independent experiments. Source data are found in Figure 5—source data 2. (c) Telomere addition frequency at the TG18 and TG34 DNA ends in rif1Δ, rif2Δ, and rif1Δ rif2Δ double mutants. Data represent the mean ± s.d. from n = 3 independent experiments. The WT data in panels (a) and (c) are the same as that shown in Figure 3c. Source data are found in Figure 5—source data 3.
-
Figure 5—source data 1
Raw data for telomere addition assays shown in Figure 5a.
- https://doi.org/10.7554/eLife.23783.028
-
Figure 5—source data 2
Raw data for telomere addition assays shown in Figure 5b.
- https://doi.org/10.7554/eLife.23783.029
-
Figure 5—source data 3
Raw data for telomere addition assays shown in Figure 5c.
- https://doi.org/10.7554/eLife.23783.030
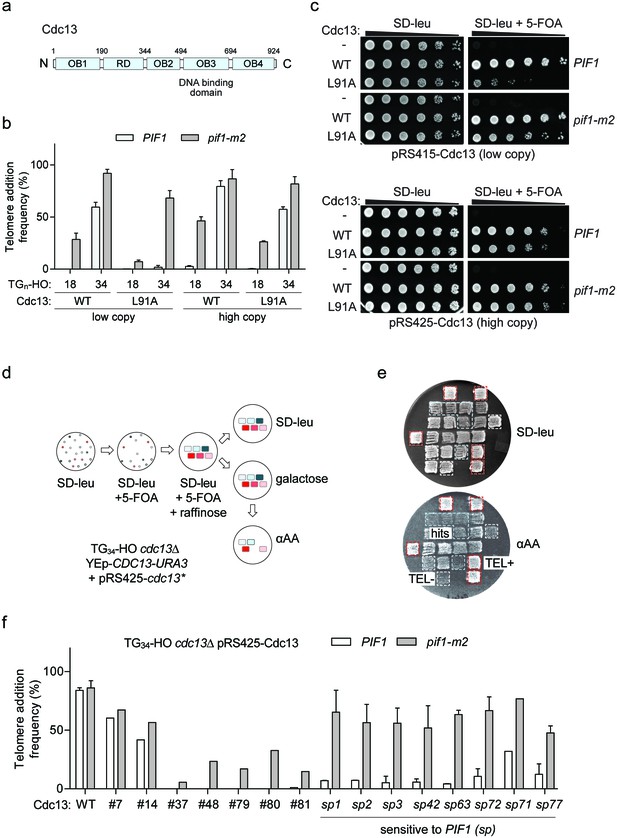
A genetic screen to identify Cdc13 mutants that prevent telomere addition at the TG34 end.
(a) Schematic of Cdc13 domain architecture consisting of four OB-fold domains (OB1-4) and a telomerase recruitment domain (RD). (b) Telomere addition frequency at the TG18 and TG34 DNA ends in cdc13Δ cells expressing wild-type CDC13 (WT) or cdc13-L91A from a low copy (pRS415) or high copy (pRS425) plasmid. Data represent the mean ± s.d. from n = 3 independent experiments. Source data are found in Figure 6—source data 1. (c) Spot assays to determine cell viability in cdc13Δ cells with a covering YEp-CDC13-URA3 plasmid and pRS415- or pRS425-derived plasmids expressing wild-type Cdc13 (WT) or Cdc13-L91A. Fivefold serial dilutions of yeast cultures were grown on SD-leu as a control, and on SD-leu +5 FOA to determine viability in the absence of the covering plasmid. Plates were grown at 30°C for 2–3 days. (d) Schematic of a screen in TG34 cdc13Δ cells using a plate-based genetic assay for telomere addition. Repaired mutant cdc13 plasmids were selected on SD-leu and the covering YEp-CDC13-URA3 removed by plating on 5-FOA before DSB induction. This step also eliminates all inviable cdc13 mutations. Plates were incubated for 2–3 days at 30°C with the exception of galactose plates which were incubated for 4 hr. An agar plate was used to reduce cell number before final selection. (e) Example of re-testing plate from the screen. Cdc13 mutants that prevent telomere addition are identified by the inability to grow on media containing α-aminoadipate (α-AA) (blue box), compared to positive control wild-type cells which add telomeres (red box) and TG18 cells that do not (white box). (f) Telomere addition frequency at the TG34 DNA end in PIF1 and pif1-m2 cells in a cdc13Δ background expressing recovered pRS425-Cdc13 mutants from the screen. Data represent the mean ± s.d. from n = 1 experiment for hits #7–81, and n = 2 independent experiments for all cdc13-sp alleles. Source data are found in Figure 6—source data 2.
-
Figure 6—source data 1
Raw data for telomere addition assays shown in Figure 6b.
- https://doi.org/10.7554/eLife.23783.032
-
Figure 6—source data 2
Raw data for telomere addition assays shown in Figure 6f.
- https://doi.org/10.7554/eLife.23783.033
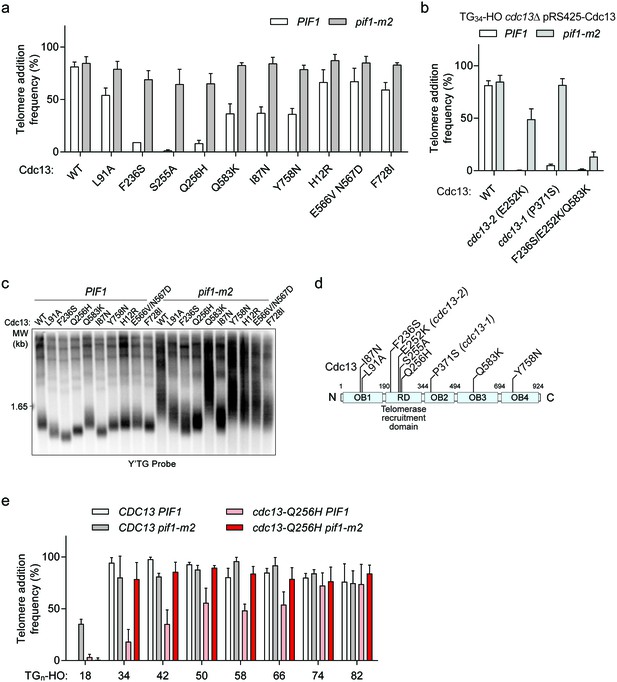
Cdc13 mutations that sensitize the TG34 end to Pif1 activity.
(a) Telomere addition frequency at the TG34 DNA end in PIF1 and pif1-m2 cells in a cdc13Δ background expressing wild-type or mutated Cdc13 from pRS425. Data represent the mean ± s.d. from n = 3 independent experiments. Source data are found in Figure 7—source data 1. (b) Telomere addition frequency at the TG34 DNA end in PIF1 and pif1-m2 cells in a cdc13Δ background expressing plasmid-borne wild-type or mutated Cdc13. The cdc13-1 mutant was grown at a permissive temperature of 23°C. Data represent the mean ± s.d. from n = 3 independent experiments. Source data are found in Figure 7—source data 2. (c) Southern blot for telomere length of the strains examined in panel (a). Cells were passaged for approximately 75 generations before genomic DNA extraction. A Y’ TG probe was used to label telomere sequences. (d) Schematic of Cdc13 domain architecture, with the most significant cdc13-sp amino acid mutations indicated. The majority of these mutations lie within the telomerase recruitment domain. (e) Telomere addition frequency at DNA ends containing 18–82 bp of TG sequence. Data represent the mean ± s.d. from a minimum of n = 3 independent experiments. Source data are found in Figure 7—source data 3.
-
Figure 7—source data 1
Raw data for telomere addition assays shown in Figure 7a.
- https://doi.org/10.7554/eLife.23783.035
-
Figure 7—source data 2
Raw data for telomere addition assays shown in Figure 7b.
- https://doi.org/10.7554/eLife.23783.036
-
Figure 7—source data 3
Raw data for telomere addition assays shown in Figure 7e.
- https://doi.org/10.7554/eLife.23783.037
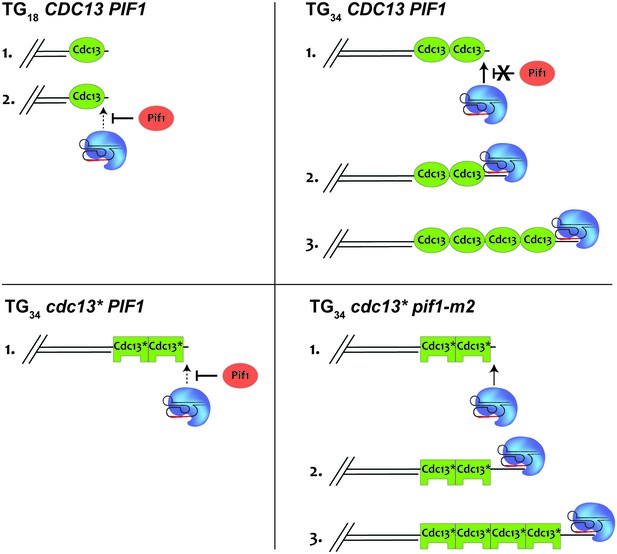
A model to explain how Cdc13 and Pif1 cooperate to establish the DSB-telomere transition.
At the TG18 end, Pif1 is able to efficiently prevent telomerase-mediated extension (top left). At the TG34 end, Cdc13-mediated recruitment of telomerase overpowers Pif1 activity, allowing the extension of the end (top right). This is impaired if the recruitment domain of Cdc13 is mutated (bottom left). Further mutation of PIF1 restores the ability of telomerase to elongate the TG34 end (bottom right).
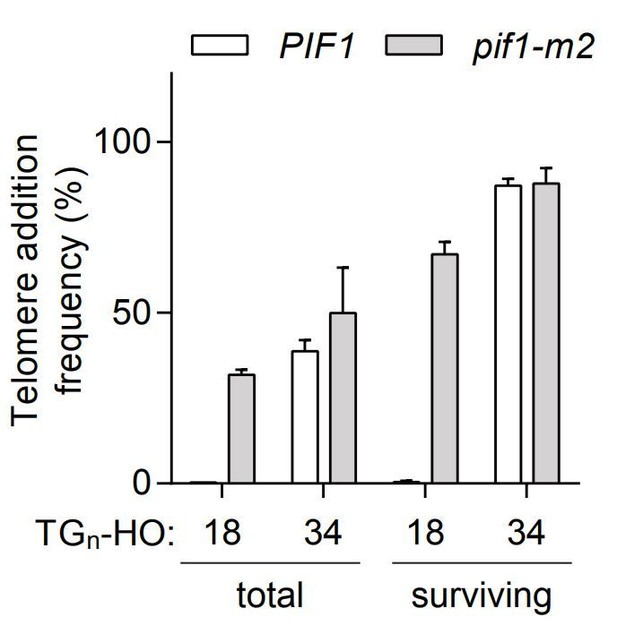
Telomere addition frequency at DNA ends containing 18 or 34 bp of TG sequence normalized either to the total amount of cells (total) or only the surviving cells after the 4h galactose induction (surviving).
Data represent the mean ± s.d. from a minimum of n=3 independent experiments.
Tables
Mutations in cdc13-sp alleles.
pRS425-cdc13* plasmids were recovered from cells grown on SD-leu media that were unable to grow on α-AA containing media. Cdc13 mutations were identified by plasmid sequencing. Mutations highlighted in red were identified by mapping experiments to determine which amino acid substitutions contribute to the mutant phenotype. Mutations highlighted in blue target important Cdc13 residues identified in this study or in previous work (Nugent et al., 1996; Lendvay et al., 1996), which are predicted to contribute to the defect, although these exact substitutions were not specifically tested.
Allele | Mutations | |||||||
---|---|---|---|---|---|---|---|---|
cdc13-sp1 | Y27F | S175P | D322G | L386M | T733A | S737C | ||
cdc13-sp3 | Q220K | L242P | K695R | |||||
cdc13-sp42 | Q36R | F58L | L131S | D150G | K161I | S170A | N194D | V217I |
S228T | K329E | L362I | F389L | |||||
cdc13-sp63 | V396I | F539Y | E716G | T756P | I767V | A807T | P896S | |
cdc13-sp72 | F96L | K129N | L179S | T291A | K296E | N426S | K469R | |
E566G | E570G | I648N | P896S | |||||
cdc13-sp77 | T3P | V38A | D102G | K135N | N240Y | E264D | T266S | |
S288C | I346V | D430G | S467R | N470S | S490A | M498V | K618R | |
E636V | H687R | L721M | V725L | D792N | T779A | |||
cdc13-sp2 | R83K | P101L | I174F | N180S | E197G | G243E | V367L | G404A |
S494P | R503G | L571R | I594M | E679G | ||||
cdc13-sp71 | H168R | I247N | E252G | T291P | V424I | K504R | F587L | T710S |
Y816H | ||||||||
clone 37 | Q66R | F96L | E121K | F142L | I342T | N378D | ||
E416A | L425F | L452M | ||||||
clone 40 | Y626F | F665Y | T907S | |||||
clone 48 | F58S | T112S | F187I | A280V | D601A | S643P | ||
clone 79 | I72V | K73R | Q94L | E192G | D219G | V238A | K296E | |
G325R | K365I | K469R | R495G | I523T | H777Q | |||
clone 80 | N14K | Y70H | I72F | L436F | F575L | |||
clone 81 | E121V | N180D | N199D | A231S | M258N | M276T | G295R | |
S314N | I366F | I412V | N455I | M525V | M579V | M625I | E716G | |
D773V | K909E |
Additional files
-
Supplementary file 1
Description of telomere sequences and yeast strains used in this study.
- https://doi.org/10.7554/eLife.23783.040
-
Transparent reporting form
- https://doi.org/10.7554/eLife.23783.041