Paternal nicotine exposure alters hepatic xenobiotic metabolism in offspring
Figures
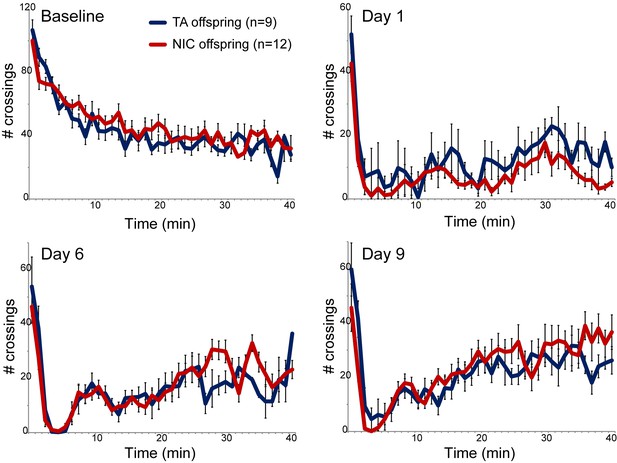
Nicotine suppression of locomotor activity is unaffected by paternal nicotine history.
Nicotine effects on locomotor activity were assayed in male offspring of control (TA) or nicotine-exposed (NIC) fathers. Data for females and alternative administration regimens are shown in Figure 1—figure supplement 3. For each plot, males were injected with either saline or nicotine immediately prior to being placed in a novel environment for 40 min, during which locomotor activity was assessed by the number of times the animal interrupted a light beam during each minute. Each time point shows the number of beam crossings in that minute, shown as average plus/minus s.e.m. for all animals tested. Importantly, here and throughout the manuscript, the listed number of animals represent the number of litters analyzed, as we only assess one animal per litter in a given assay. Data are shown for saline injection (‘Baseline’) – exploratory behavior decreases over time in saline-injected animals as they habituate to the locomotor cage – and for 1.5 mg/kg nicotine injection in animals naïve to nicotine (Day 1) or following five or eight prior days of the same nicotine injection and locomotor assessment protocol.
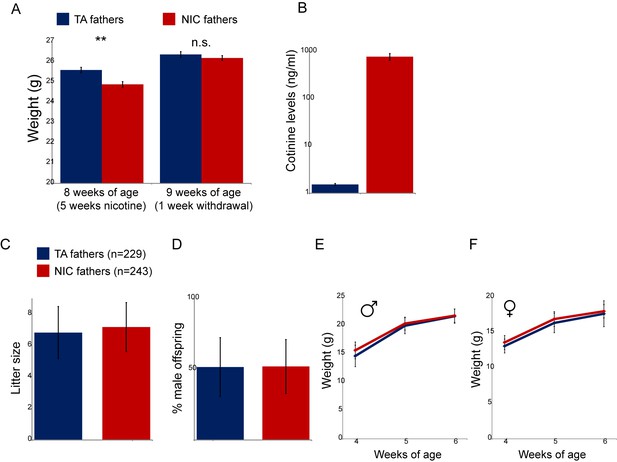
Physiological effects of nicotine exposure on treated males.
(A) Weight of males subject to 5 weeks of exposure to nicotine (NIC) or control (TA) solution. Data are shown for animals at the end of 5 weeks of nicotine exposure (** indicates p<0.01), and following a week of withdrawal (n.s.: not significant), as indicated. (B) Blood levels of cotinine, a relatively long-lived metabolite of nicotine, in males (at 8 weeks of age, following 5 weeks of nicotine/control treatment) consuming control or nicotine solutions. (C) Average litter size for offspring of control and nicotine-treated males. Data show average plus/minus s.e.m. (D) Average gender ratio for offspring of control and nicotine-treated males. Data show average plus/minus s.e.m. (E–F) Average weights for male (E) and female (F) TA and NIC offspring at 4, 5, and 6 weeks of age. Data are shown as average plus/minus S.D.
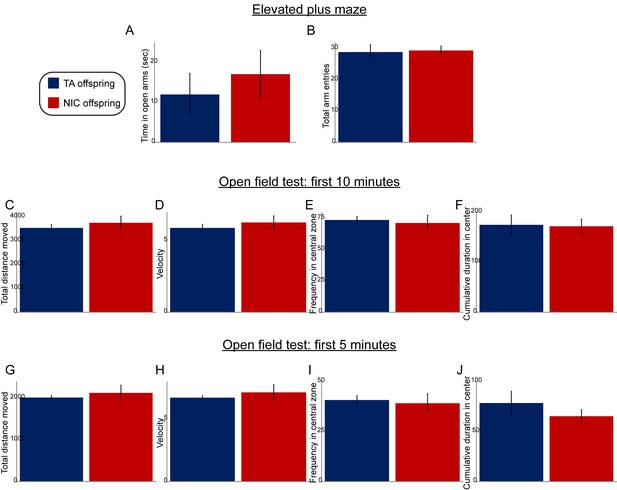
Paternal nicotine exposure does not affect offspring anxiety-related behaviors.
(A–B) Data are shown for elevated plus maze performance – time spent in open arms (A), or total entries into the open arms (B) – for TA offspring (n = 7) and NIC offspring (n = 11). (C–J) Open field test performance, shown for the first 10 min (C–F) or first 5 min (G–J) following introduction of the animal into the enclosure. Panels show total distance moved (C,G), velocity (D,H), fraction of time spent in the center of the open field enclosure (E,I), and cumulative time spent in the central zone (F,J). All data show average +/- s.e.m. None of the differences between TA and NIC offspring are statistically significant.
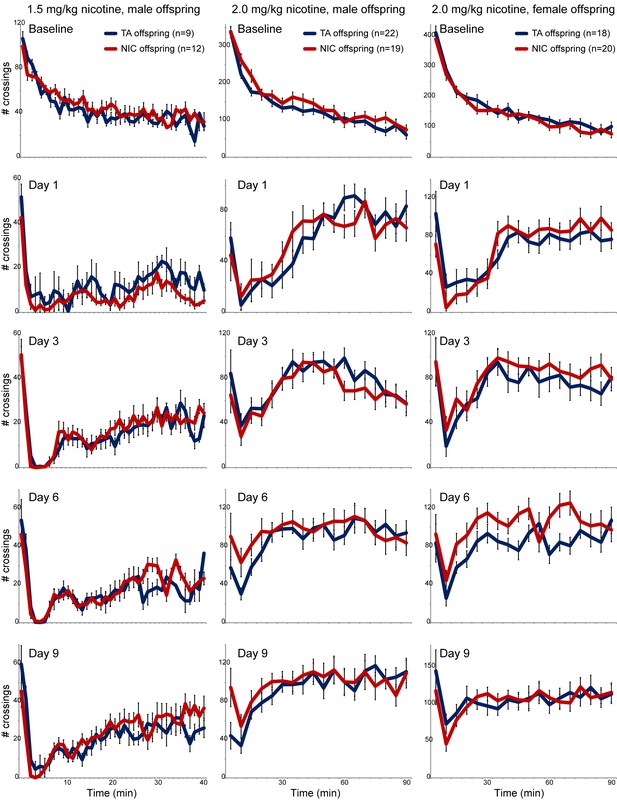
No significant effects of paternal nicotine exposure on offspring locomotor response to nicotine.
For each column, offspring of control and nicotine-treated males (TA and NIC, respectively) were subject to a locomotor activity assay as follows. Animals were first acclimated to intraperitoneal saline injections once a day for three days. On day three (Baseline), offspring were injected with saline, then placed in a novel environment – a box equipped with infrared photodiodes to enable detection of locomotor activity. Saline-injected animals actively explore the novel environment, and locomotor activity is quantitated over a 40 or 90 min time course by the number of times the animal interrupts the light beam. Exploratory behavior decreases over time in saline-injected animals as they fully explore the enclosure. On nine subsequent days (data for four representative days are shown in each column), animals are injected with nicotine (1.5 or 2.0 mg/kg, as indicated) and immediately introduced to the measurement box. In this paradigm, injection of nicotine results in rapid suppression of locomotor activity, followed by a gradual recovery of exploratory behavior over the time course of the assay. Data here are shown as mean plus/minus s.e.m.
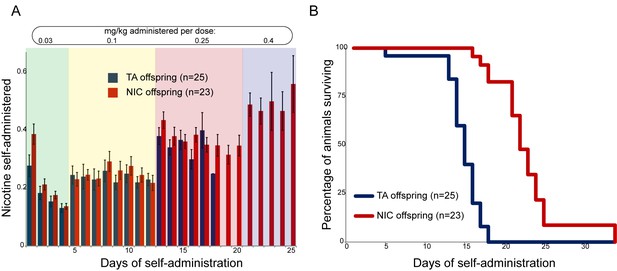
Paternal experience affects nicotine toxicity, but not self-administration, in offspring.
(A) Paternal nicotine exposure does not affect nicotine self-administration in offspring. Each day, a mouse trained to self-administer nicotine (Materials and methods) was connected to the self-administration apparatus for one hour, with the dose of nicotine administered via cannula for every correct nose poke ramping up every 4–8 days, as indicated. Total nicotine self-administered is shown for each day of the protocol as average and s.e.m. Note that the numbers of animals participating in the trial decreased over time due to removal from the protocol (clogged catheter) or death – the listed n represents all animals that remained on the protocol until death. (B) Offspring of nicotine-exposed fathers exhibit significant protection from nicotine toxicity. Survival curve is shown for all animals on the self-administration protocol (underlying data are provided in Figure 2—source data 1). Nicotine offspring exhibited significantly increased survival during the time course of the assay relative to control offspring (Kaplan-Meyer survival curve, p<0.0001 for both Log-rank test and Gehan-Breslow-Wilcoxon test).
-
Figure 2—source data 1
Offspring of nicotine-exposed fathers exhibit significant protection from nicotine toxicity.
Survival curve for all animals on the nicotine self-administration protocol.
- https://doi.org/10.7554/eLife.24771.008
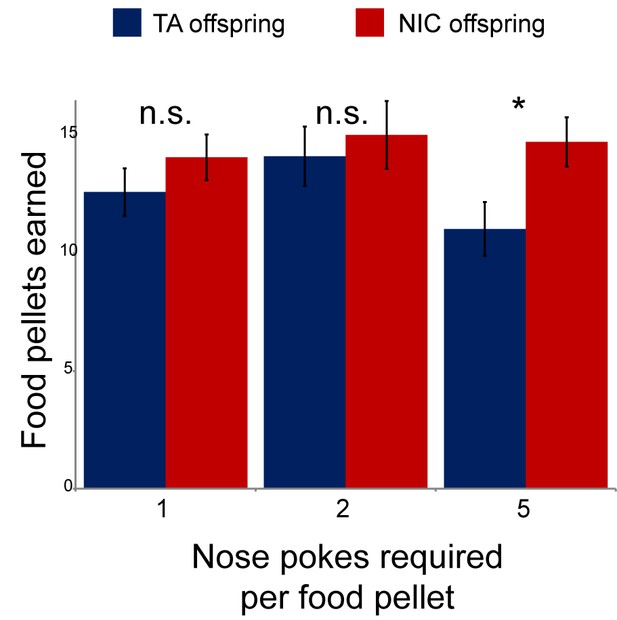
Modest effect of paternal nicotine exposure on dietary training.
Following surgical implantation of a central line, TA and NIC offspring were allowed to recover for 3 days. Animals were then subject to caloric restriction (80% of daily diet w/w compared to animals feeding ad libitum), placed in a self-administration box with two buttons, one of which was marked with a small light. Animals were then provided with sucrose pellets in response to a nose poke on the lit button – for 3 days a pellet was provided following each correct nose poke, then for one more day two nose pokes were required for a pellet, and finally five nose pokes were required for a food pellet for 3 days. Bars here show the number of food pellets earned in 1 hr for TA and NIC offspring – NIC offspring earned moderately more sucrose pellets in the final reward regime than TA offspring (p=0.03). This enhanced food training carried over to the first day of nicotine self-administration (Figure 2A), when NIC animals self-administered slightly more nicotine than TA animals, but this difference only persisted for the first day.
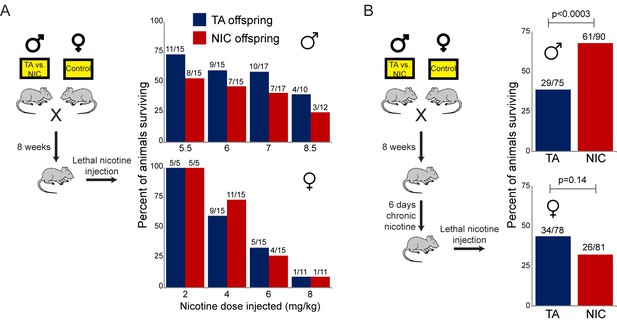
Paternally-induced protection from nicotine toxicity is primed by nicotine exposure in offspring.
(A) Survival of TA or NIC offspring following a single injection of nicotine at the indicated dose. Above each bar, fraction shows the number of surviving animals over number of animals injected. For all four doses tested, there was no significant difference in toxicity between TA and NIC offspring (p>0.7 across all four doses for males, p>0.8 for females). (B) Survival of TA and NIC offspring following a single injection of nicotine at roughly the LD50 for naïve animals in (A) – 7.2 mg/kg for male offspring, shown in the top panel, 5.04 mg/kg for females, shown in the bottom panel. Here, offspring were acclimated to chronic nicotine in their drinking water for 6 days, with nicotine challenge being administered 24 hr following the last day of nicotine consumption.
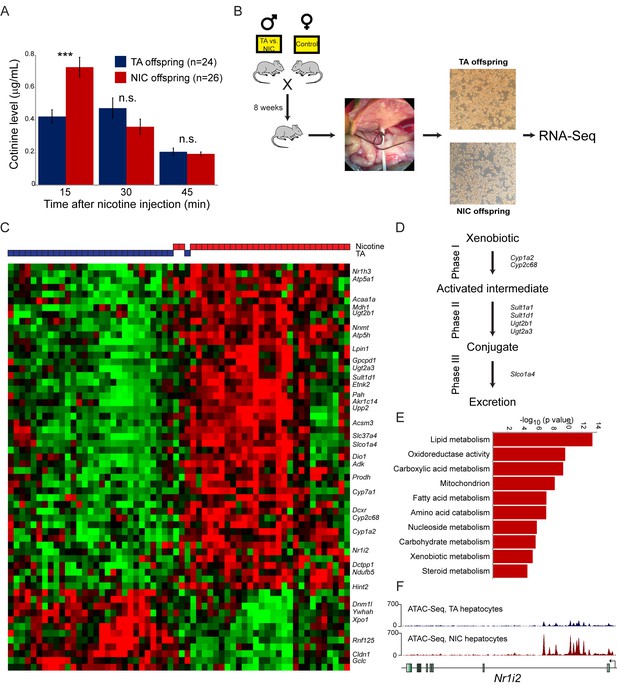
Paternal nicotine exposure induces an exaggerated protective response to xenobiotics.
(A) Paternal nicotine exposure enhances nicotine metabolism in offspring. Male TA and NIC offspring were acclimated to nicotine for 6 days, then 24 hr later were injected with 1.5 mg/kg nicotine. Serum levels of the long-lived nicotine metabolite cotinine were measured at the indicated times after nicotine injection, with significantly (p<0.0002, t-test with Holm-Sidak correction) elevated cotinine levels being observed at the earliest time point analyzed, indicating enhanced nicotine clearance in NIC offspring. (B) Schematic of hepatocyte RNA-Seq experiment. (C) Cluster of hepatocyte RNA-Seq dataset. For each paternal treatment group (TA or NIC), data are shown for ten individual male offspring from ten separate litters, with hepatocytes from five animals also being cultured for varying times (0 to 21 hr) following isolation. Data are z score normalized for each culture time point. The heatmap shows 60 genes (filtered for average expression >25 ppm) changing with a multiple hypothesis-corrected p value<0.1. Underlying data are provided in Figure 4—source data 1. (D) Genes upregulated in NIC offspring encode enzymes involved in all three phases of xenobiotic metabolism, as indicated. (E) Selected Gene Ontology categories enriched among genes upregulated (adjusted p<0.1) in NIC hepatocytes. (F) ATAC-Seq coverage for TA and NIC hepatocytes, as indicated, across Nr1i3. See also Figure 4—figure supplement 3.
-
Figure 4—source data 1
Cluster of hepatocyte RNA-Seq dataset.
RNA-Seq data for hepatocytes obtained from TA and NIC offspring, showing 60 genes (filtered for average expression >25 ppm) changing with a multiple hypothesis-corrected p value <0.1. Data are z score normalized for each culture time point.
- https://doi.org/10.7554/eLife.24771.012
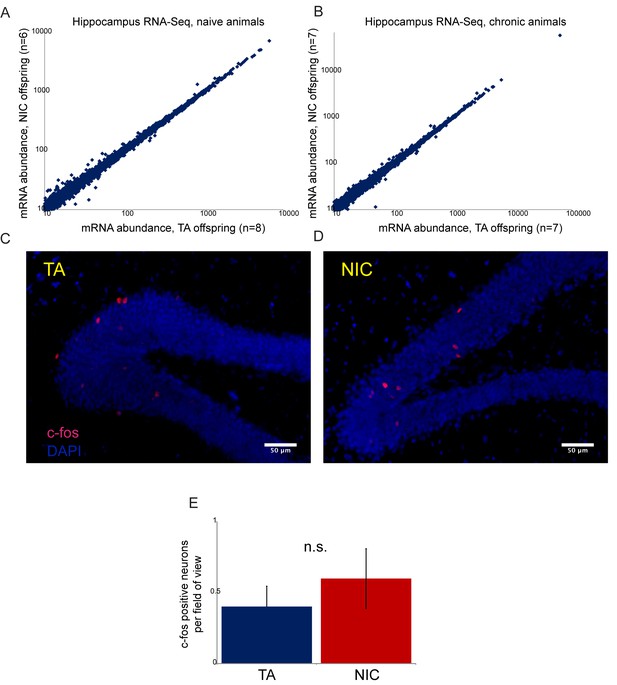
Paternal nicotine has no significant effects on offspring hippocampal gene regulation or neural activity.
(A–B) RNA-Seq of isolated hippocampus from TA and NIC offspring. Scatterplots show average mRNA abundance (minimum tpm of 10), with x axis showing average for TA offspring and y axis showing average for NIC offspring. (A) shows data for TA and NIC animals that had not experienced nicotine (‘naïve’), (B) shows data for animals provided with chronic nicotine for 6 days. There are no significant effects of paternal nicotine exposure on any mRNAs in either condition. Similar results were obtained in preliminary studies of the ventral tegmental area, nucleus accumbens, and prefrontal cortex (not shown). Importantly, while recent reports document an increase in Igf2 mRNA abundance in the hippocampus of 8 week old male offspring of stressed fathers (Short et al., 2016), we observed no significant change in Igf2 levels in NIC offspring (see Supplementary file 1), providing another argument against the hypothesis that our paternal nicotine exposure paradigm affects offspring via a paternal stress response. (C–D) Paternal nicotine treatment does not affect offspring neural activity in the hippocampus. Representative images showing c-fos staining as a proxy for neural activity in hippocampus isolated from TA (C) and NIC (D) offspring. Here, animals were put on chronic nicotine (200 µg/ml nicotine free-base) for six days. After 24 hr without nicotine, animals were injected with 1.5 mg/kg nicotine free-base. Tissue was collected 90 min after the injection. (E) Quantitation of c-fos staining data. Y axis shows number of c-fos-positive neurons in the gyrus dentatus for nicotine-injected TA (n = 12), and NIC (n = 13) offspring. Several lines of evidence thus argue against the drug resistance of NIC offspring resulting from altered neural physiology. First, the fact that drug-acclimated animals exhibit enhanced resistance to both nicotine and cocaine toxicity (Figures 3 and 5) rules out mechanisms involving downregulation or desensitization of either nicotinic acetylcholine receptors or the dopamine receptor. Second, RNA-Seq analysis of several brain regions – hippocampus, ventral tegmental area, nucleus accumbens, and prefrontal cortex -- revealed minimal effects of paternal nicotine exposure on the transcriptome. Finally, we found no significant differences in staining patterns of the activity marker c-fos in the hippocampus of TA and NIC offspring. Thus, while we cannot definitively rule out a neural basis for the phenotypes observed in NIC offspring, we found no evidence to support such a hypothesis.
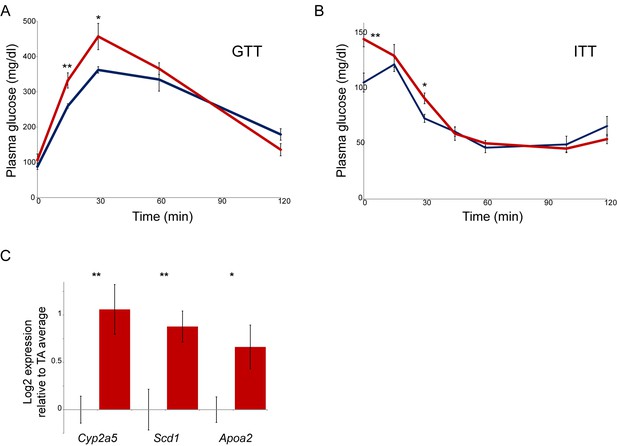
Paternal nicotine exposure affects multiple phenotypes in offspring.
(A–B) Glucose tolerance (A) and insulin tolerance (B) are significantly altered in NIC offspring relative to TA offspring. Plasma glucose levels are shown for six male NIC or TA offspring at varying times after a 2 g/kg glucose bolus at 7 weeks of age (A), or a 0.75 U/kg insulin bolus at 10 weeks of age (B). * and ** represent p values of <0.05 and <0.01, respectively (t-test). (C) q-RT-PCR data for the indicated genes. In each case, expression level (after normalizing to Actb and Gapdh) is plotted relative to the average expression level for 4 TA livers (n = 6 NIC livers), with bars showing average and s.e.m.
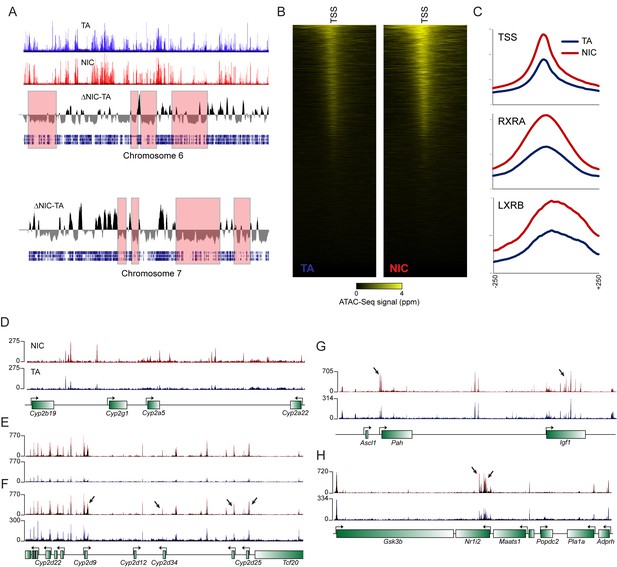
Global differences in hepatocyte chromatin architecture between TA and NIC offspring.
Aggregated ATAC-Seq data for hepatocytes isolated from TA and NIC offspring (n = 4 animals each, with dexamethasone-treated and -untreated samples for each animal). (A) Multimegabase-scale differences in the accessibility landscape of TA and NIC hepatocytes. Top two panels show ATAC-Seq data for NIC and TA offspring for chromosome 6, along with an averaged NIC-TA score, followed by gene density. For chromosome 7, only NIC-TA and gene density are shown. Red boxes highlight a subset of genomic regions of low gene density in which TA hepatocytes exhibit greater ATAC-Seq signal than NIC hepatocytes. Conversely, gene-dense regions generally exhibit higher ATAC signal in NIC hepatocytes (not highlighted). The mechanistic basis for this global difference is unclear – it does not appear to reflect contamination of TA samples with dead cells, for example, as these samples (from nicotine-naïve animals) did not differ in viability, and plating of hepatocytes also effectively selects against dead cells. As fragment length distributions were consistent from library to library, it also seems unlikely that there were gross differences in the concentration or activity of the added Tn5. Nonetheless, while this difference could reflect meaningful biology, such as a global difference in heterochromatin condensation, global differences in any genome-wide assay should of course be viewed with skepticism. (B) ATAC-Seq data for 500 bp surrounding all annotated transcription start sites (TSSs), sorted from high to low average ATAC signal intensity. (C) Increased ATAC signal in NIC hepatocytes is shown for all TSSs, or RXRA or LXRB binding sites, as indicated. (D–H) Examples of loci exhibiting enhanced chromatin accessibility in NIC offspring, relative to TA offspring. (D–E) show ATAC-Seq tracks in which TA and NIC data are set to the same vertical range, as in Figure 4F. For panels F-H, y axes are set independently for TA and NIC datasets, visually correcting for the global differences between TA and NIC datasets. In these panels, a subset of significantly NIC-enriched peaks (Supplementary file 3) are indicated with arrows.
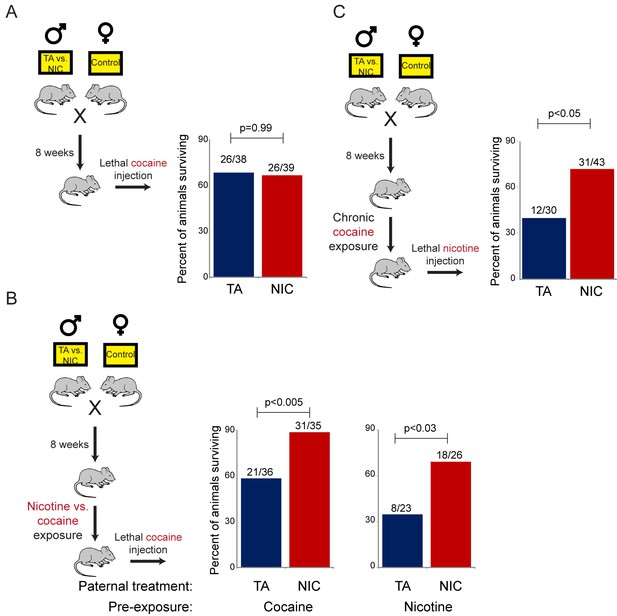
NIC offspring are protected from multiple xenobiotics.
(A) Paternal nicotine exposure does not affect susceptibility of drug-naïve offspring to cocaine toxicity. Male TA and NIC offspring were injected with a single 100 mg/kg dose of cocaine. Survival is shown as in Figure 3. (B) Acclimation of TA and NIC offspring to either nicotine or to cocaine reveals protective effect of paternal nicotine exposure on offspring cocaine resistance. As in (A), for male offspring acclimated to chronic nicotine (200 µg/mL nicotine free-base in drinking water for six days) or cocaine (twice-daily injections with 15 mg/kg cocaine for five days). Twenty-four hours following final drug exposure, animals were injected with a single 100 mg/kg dose of cocaine. (C) Cocaine acclimation induces nicotine resistance in NIC offspring. Here, male TA and NIC offspring were acclimated to cocaine injections (twice-daily, 15 mg/kg) over five days. Twenty-four hours after the final cocaine injection, animals were injected with 7.2 mg/kg nicotine.
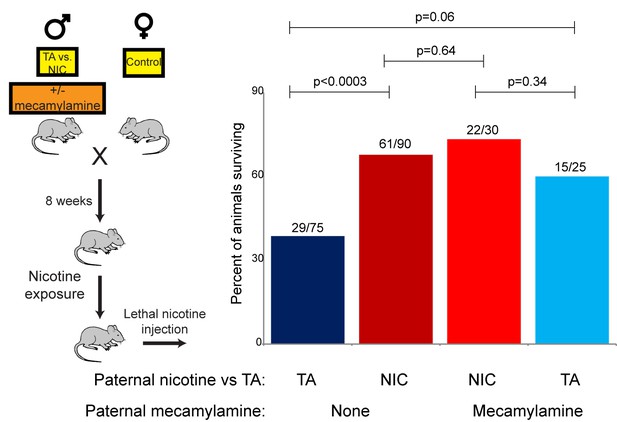
Offspring drug resistance is induced by a nicotine antagonist.
Here, we modified the paternal exposure paradigm by implanting pumps to deliver the nicotine antagonist mecamylamine to male mice. Mecamylamine-treated mice were provided with nicotine or control solution for four weeks, then mated to control females. Male offspring were acclimated to chronic nicotine for six days and then subject to a toxic nicotine challenge, and survival is shown as in Figures 3 and 5. Data for no mecamylamine animals are reproduced from Figure 3B. Note that concurrent mecamylamine and nicotine exposure resulted in a protective effect on offspring, and even mecamylamine alone was able to modestly induce nicotine resistance in the next generation.
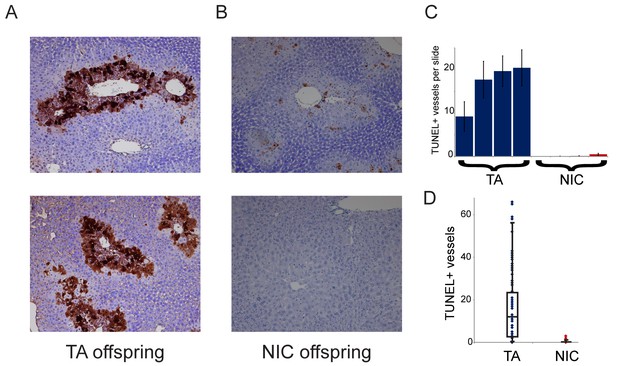
NIC offspring exhibit relative sparing of hepatocytes following chronic drug exposure.
(A–B) Effects of chronic cocaine treatment on hepatocyte viability. Two representative sections are shown for TUNEL-stained livers from TA (A) and NIC (B) offspring following five days of cocaine injections (twice-daily, 15 mg/kg). Prominent centrilobular apoptosis is seen in TA offspring, but is almost completely absent in NIC offspring. (C–D) Quantitation of TUNEL staining data. (C) shows the average (plus/minus s.e.m.) number of TUNEL+ centrilobular regions per slide (staining of >25% of central vein circumference was counted as TUNEL+, and was assessed at five different levels for each liver lobe I-IV) for four individual TA (blue) and NIC (red) offspring, treated as in (A–B). (D) shows data for all individual slides as dots, with boxplot showing median, one standard deviation, and 5th/95th percentile for the 80 data points.
Additional files
-
Supplementary file 1
RNA-Seq of TA and NIC hippocampus.
RNA-Seq data for hippocampus isolated from male TA or NIC offspring, with samples being collected from drug-naïve animals, from ‘chronic’ animals subject to 6 days of nicotine administration prior to 24 hr of withdrawal, or subject to chronic nicotine exposure followed by a single sublethal injection of nicotine.
- https://doi.org/10.7554/eLife.24771.019
-
Supplementary file 2
Hepatocyte RNA-Seq, naïve hepatocytes.
RNA-Seq data for hepatocytes isolated from male TA or NIC offspring, then cultured in vitro for varying times as indicated.
- https://doi.org/10.7554/eLife.24771.020
-
Supplementary file 3
Hepatocyte sATAC-Seq.
List of peaks exhibiting significantly increased ATAC-Seq signal in NIC hepatocytes, relative to TA hepatocytes.
- https://doi.org/10.7554/eLife.24771.021