An intersectional gene regulatory strategy defines subclass diversity of C. elegans motor neurons
Figures
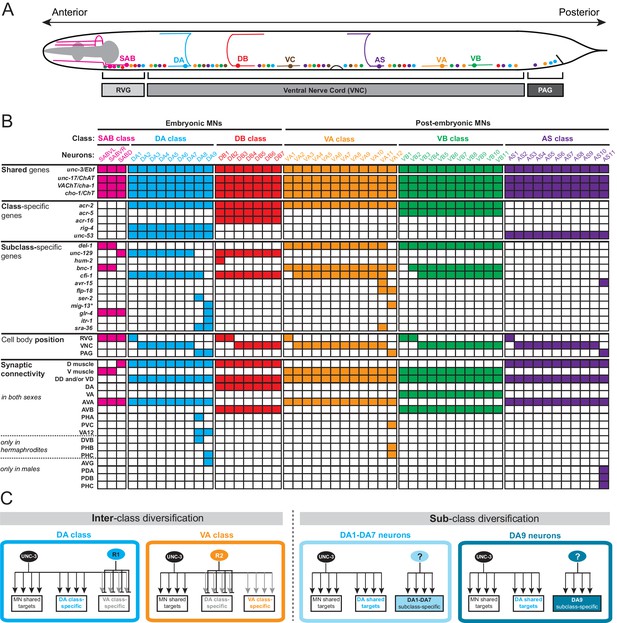
A map of subclass-specific genes provides an entry point to study MN subclass diversification.
(A) Schematic showing seven C. elegans MN classes (SAB, DA, DB, VA, VB, AS, VC), which are color-coded. Individual neurons of each class intermingle and populate three regions along the A-P axis; retrovesicular ganglion (RVG), ventral nerve cord (VNC), preanal ganglion (PAG). Axonal trajectory is shown only for one member of each class. (B) A map of effector gene expression with single-cell resolution. Each column represents an individual neuron. Genes that are shared, class- and subclass-specific are shown on the left. Cell body position and connectivity similarities and differences are also shown for each individual motor neuron. Asterisk next to mig-13 indicates additional expression (not shown) in MNs located in the VNC but anterior to vulva. (C) Inter-class versus sub-class diversification. The left panel shows known mechanisms for interclass-specification. Class-specific repressor proteins counteract unc-3’s activity, thereby generating ‘inter’-class diversity in C. elegans MNs (example of DA and VA class is shown) (Kerk et al., 2017). The right panel illustrates the problem of subclass diversification: Within a given class, the mechanisms controlling the expression of subclass-specific genes and thereby generate ‘intra’-class diversity are not known (example of DA class is shown).
-
Figure 1—source data 1
Summary of synaptic wiring data.
(extracted from www.wormwiring.org). <> = gap junction (electrical synapse), > = chemical synapse, bwm = body wall muscle, MSN = male-specific neuron (all such connections lettered in blue). Note that only some subclass-specific connections are non-dimorphic (green), while most are sexually dimorphic (red). Only connections represented by >3 serial sections are shown. The extent of dimorphism of the VB1 neurons is not currently known because of absence of available male data.
- https://doi.org/10.7554/eLife.25751.003
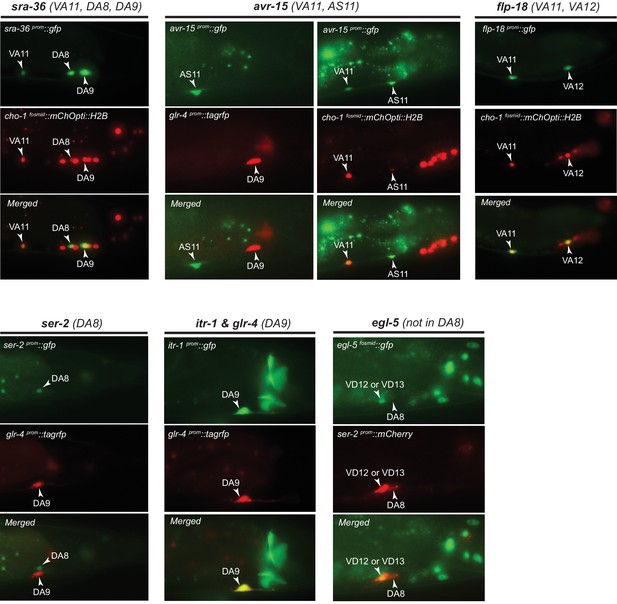
Validation of MN subclass-specific gene expression.
Transgenic gfp reporter animals for sra-36, avr-15, flp-18, ser-2, itr-1, and egl-5 were crossed with subclass-specific red fluorescent markers (ser-2, glr-4) or a marker that labels all cholinergic MNs (cho-1). Co-localization of red and green fluorescence, axonal anatomy, time of birth and the relative location and order of neurons along the A-P axis allowed for identification of MN subclasses expressing the respective reporter.
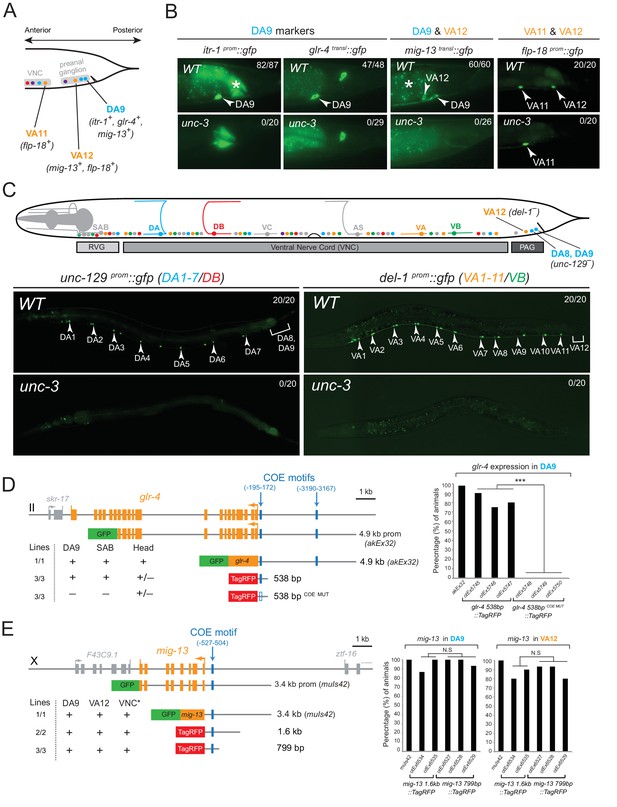
The terminal selector UNC-3 is required for MN subclass diversification.
(A) Schematic showing subclass-specific gene expression in posterior MNs. (B–C) The expression of multiple subclass-specific markers (DA9: itr-1, glr-4, mig-13; VA12: mig-13, flp-18; DA1-7/DB: unc-129; VA1-11/VB: del-1) is dramatically affected in unc-3(e151) null mutant animals. Note that flp-18 expression in VA11 is unaffected in unc-3 null animals. Translational fusion reporters were used for glr-4 (glr-4 transl::gfp) and mig-13 (mig-13 transl::gfp), while promoter fragments were fused to gfp for itr-1, flp-18, unc-129, and del-1. Asterisks in B mark autofluorescence from the C. elegans intestinal cells. Numbers at top right of each image indicate the fraction of animals that showed marker expression in the respective MNs. Arrowheads in B and C mark MN subclasses (DA9, VA12, DA1-7 and VA1-11). In schematic shown in C, only DA, DB, VA, and VB MNs are color-coded. (D–E) Cis-regulatory mutational analysis is shown for glr-4 (expressed in DA9 and SAB neurons) and mig-13 (expressed in DA9 and VA12) genes. The glr-4 and mig-13 loci are schematized. Lines indicate genomic region fused to gfp (green) or tagrfp (red). (+) indicates robust reporter gene expression in the respective neurons. (±) indicates significant reduction in the number of neurons expressing the reporter when compared to transgenic animals carrying longer genomic fragments of the cis-regulatory region. (–) indicates complete loss or very faint expression in the respective neurons. Multiple transgenic lines were analyzed for each construct. At least twenty animals were analyzed per transgenic line. Detailed quantification of this analysis is provided on the right. Location of each COE motif (blue vertical lines) is presented as distance from ATG (+1). MUT indicates that COE motif has been mutated (shown as empty blue box) through substitution of 2 nucleotides in the core sequence (for example, COE wild-type site: TCCCNNGGGA >> COE MUT site: TGGCNNGGGA). ***p value < 0.001. Asterisk (*) on VNC in E indicates additional mig-13 expression in VNC MNs anterior to the vulva.
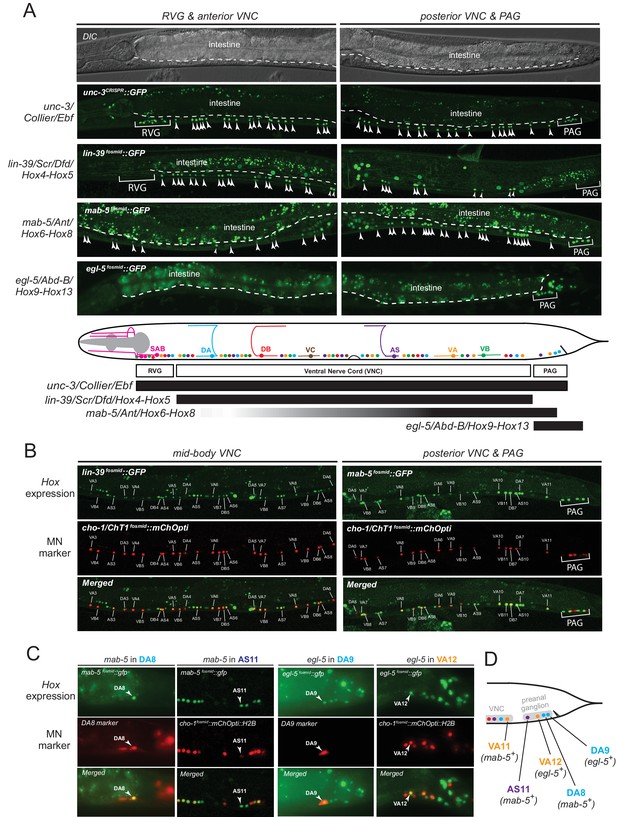
The C. elegans HOX genes egl-5/Abd-B/Hox9-Hox13, lin-39/Scr/Dfd. /Hox4-Hox5 and mab-5 (Antp)/Hox6-Hox8 are expressed in a subclass-specific manner.
(A) Fosmid-based reporters for egl-5, lin-39 and mab-5 reveal MN subclass-specific expression. A differential interference contrast (DIC) image of the anterior and posterior half of a C. elegans animal is provided at the top. This DIC image comes from the unc-3CRISPR::gfp animal, which is used to monitor endogenous unc-3 expression. As previously reported (Kratsios et al., 2011; Pereira et al., 2015), unc-3 is expressed in all individual neurons of a given MN class; expression is detected in MNs at the ganglia (RVG and PAG), as well as the ventral nerve cord (VNC). Arrowheads mark individual MN nuclei as expected since gfp is fused to the UNC-3, LIN-39, MAB-5, EGL-5 nuclear proteins. Autofluorescence of the intestinal cells is evident in the green channel. A dotted white line marks the limit of the intestinal cells, which are located dorsally to MNs. A schematic summarizing the intersectional expression of unc-3 and C. elegans Hox genes in MNs is provided at the bottom. Hox gene names are provided with their fly and vertebrate paralogs. (B–C) Animals carrying fosmid-based Hox gfp reporters were crossed with animals carrying red fluorescent reporters for either all cholinergic MNs (cho-1 fosmid::mChOpti::H2B) or subclass-specific markers (DA8: ser-2prom::mCherry; DA9: glr-4prom::tagrfp). We found that Hox genes are expressed in a subclass-specific manner during larval and adult stages. Representative images of adult (day 1) animals are shown. (D) Schematic that summarizes HOX gene (egl-5, mab-5) expression in MNs of the preanal ganglion (PAG).
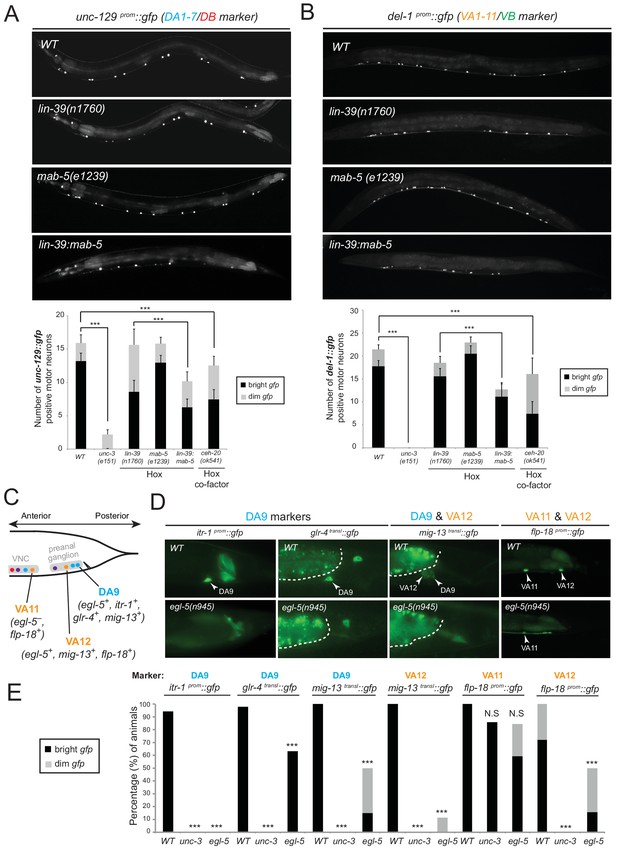
Hox genes – like unc-3 – control the expression of MN subclass-specific genes along the A-P axis.
(A–B) The expression of the subclass-specific markers unc-129 (DA1-7/DB) and del-1 (VA1-11/VB) is significantly affected in lin-39 mab-5 double mutants, as well as in animals lacking ceh-20, C. elegans PBX homolog known to function as Hox co-factor. Quantification of the number of MNs expressing the gfp reporter (bright or dim) is provided at the bottom. Effects on reporter expression are milder in Hox null mutants when compared to unc-3 null mutants. Error bars represent standard deviation (STDV). ***p value < 0.001. N > 20 animals. (C–D) The posterior Hox gene egl-5/Abd-B/Hox9-Hox13 controls subclass-specific genes (itr-1, glr-4, mig-13, flp-18) expressed in the most posterior member of the DA (DA9) and VA class (VA12). Effects on reporter expression are milder in egl-5 null mutants when compared to unc-3 null mutants. Similar effects were observed for multiple egl-5 loss-of-function alleles (n945, u202, tm4746). A dotted white line marks the limit of the intestinal cells. (E) Quantification (percentage of animals) is provided with grey bars representing very dim gfp expression in the respective neuron, while black bars represent bright gfp expression. ***p value < 0.001. N > 20 animals.
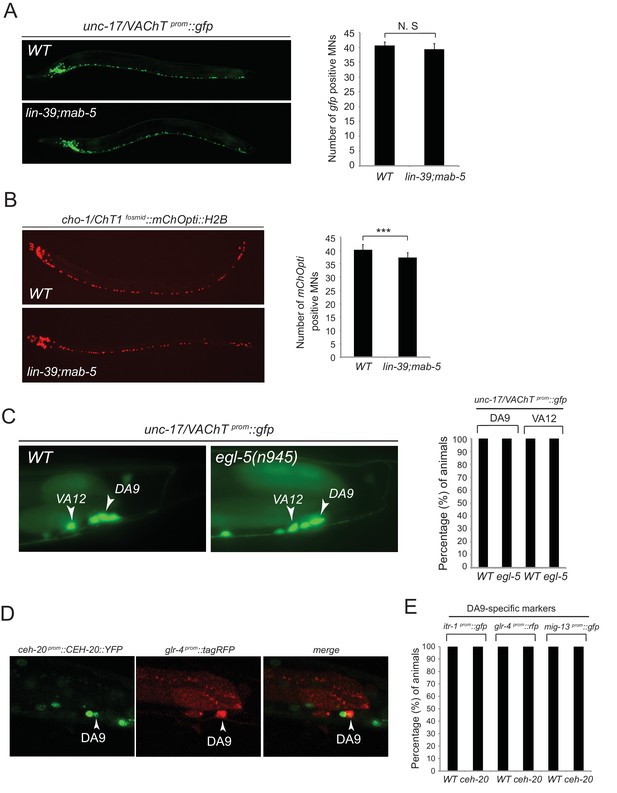
Analysis of acetylcholine pathway gene expression in Hox mutants and ceh-20/Pbx analysis.
(A) The expression of the acetylcholine (ACh) pathway gene unc-17/VAChT is not affected in cholinergic ventral nerve cord MNs of lin-39(n1760)mab-5(e1239) double mutant animals. Quantification is provided on the right. N.S, not statistically significant differences. N = 15. Expression was assessed using a transcriptional reporter for unc-17/VAChT (unc-17 prom::gfp). (B) Expression of the ACh pathway gene cho-1/ChT1 was mildly affected in lin-39(n1760);mab-5(e1239) double mutant animals because the cho-1 fosmid mChOpti::H2B fosmid-based reporter (unlike unc-17 prom::gfp) also labels six MNs of the VC class, which are not generated in lin-39(n1760);mab-5(e1239) mutants. N = 15. ***p value < 0.001. Error bars represent standard deviation (STDV). (C) The expression of unc-17/VAChT is not affected in DA9 and VA12 neurons of egl-5(n945) mutant animals. Quantification is provided on the right. N = 25. (D) The expression of ceh-20 in the DA9 neuron at the L4 stage is confirmed using glr-4prom::tagRFP as a DA9-specific marker. (E) The expression of the DA9-specific markers itr-1, glr-4, and mig-13 is unaffected in ceh-20(ok541) mutants (L4 stage). N = 10.
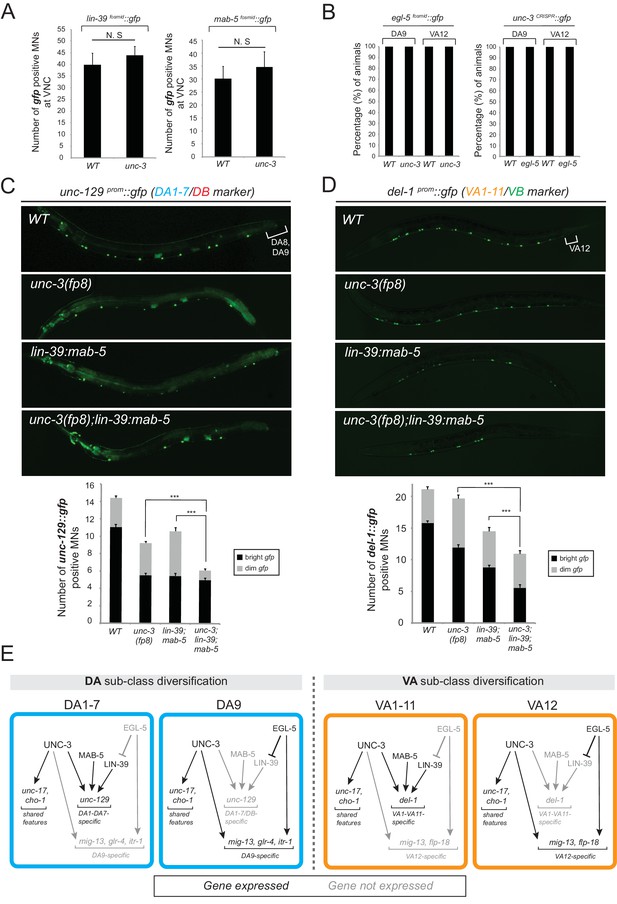
The terminal selector unc-3 and the HOX genes egl-5/Abd-B/Hox9-Hox13, lin-39/Scr/Dfd/Hox4-Hox5 and mab-5/Antp/Hox6-Hox8 act synergistically.
(A) The expression of lin-39 and mab-5 is not affected in ventral cord MNs of unc-3 mutants. The number of lin-39 and mab-5 positive MNs was quantified in wild-type and unc-3(e151) animals. N.S: not statistically significant differences. Error bars represent standard deviation (STDV). N = 15. (B) The percentage of animals that show egl-5 expression in DA9 and VA12 neurons of unc-3(e151) mutants was quantified and no differences were observed. Similarly, no differences in the expression of unc-3 in the DA9 and VA12 neurons of egl-5(n945) mutants were observed. N = 10. (C–D) Animals carrying a hypomorphic unc-3 allele (fp8) show mild defects on unc-129 (DA1-7/DB marker) and del-1 (VA1-11/VB marker) expression that are comparable to lin-39 (n1760) mab-5 (e1239) double mutants. Triple mutant animals unc-3(fp8); lin-39 (n1760) mab-5 (e1239) display even stronger (additive) effects providing evidence that unc-3, lin-39 and mab-5 act synergistically. Quantification of the number of neurons expressing the gfp reporter is provided. Error bars represent standard deviation (SEM). ***p value < 0.001. N = 20. Animals from all genotypes shown here were grown at 15°C because fp8 is a hypomorphic, temperature-sensitive allele. (E) Genetic model to summarize our data for DA and VA subclass diversification.
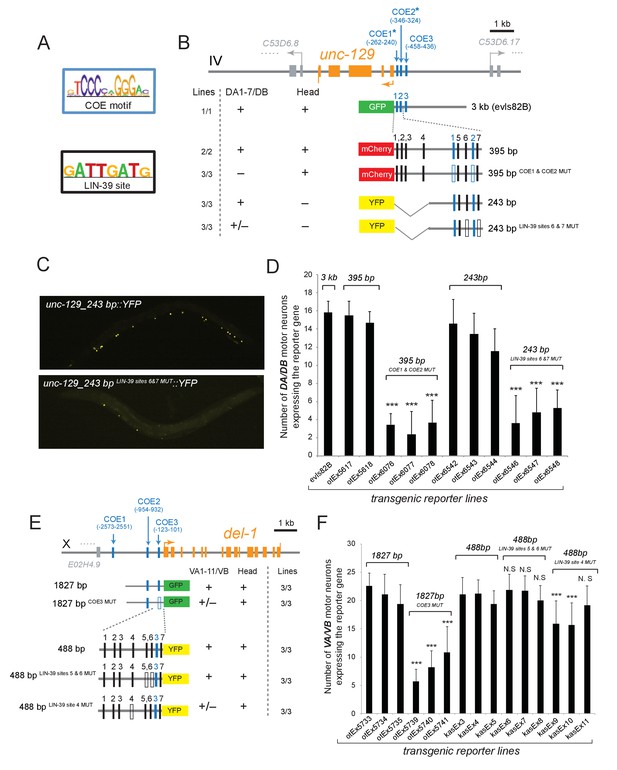
The Hox gene lin-39/Scr/Dfd/Hox4-Hox5 and the terminal selector unc-3 regulate directly the expression of the subclass-specific genes unc-129/TGFβ and del-1.
(A) The binding site for UNC-3 (COE motif) and LIN-39 are shown. The COE motif has been previously characterized (Kratsios et al., 2011), while the LIN-39 site was identified though ChIP-seq experiments (Boyle et al., 2014). (B–D) A schematic of the unc-129 locus is shown. Cis-regulatory mutational analysis was performed in the context of transgenic animals. Lines indicate genomic region fused to gfp (green), mCherry (red) or yfp (yellow). (+) indicates robust reporter gene expression in DA1-7/DB and head neurons. (±) indicates significant reduction in the number of neurons expressing the reporter. (–) indicates complete loss or very faint expression in the respective neurons. Multiple transgenic lines were analyzed for each construct. At least 20 animals per transgenic line were analyzed. Detailed quantification of this analysis is provided in D. Location of each COE motif (UNC-3 binding site, shown as blue vertical line) is presented as distance from ATG (+1). The blue asterisk indicates that the COE motif is conserved in at least three other nematode species. The 395 bp and the 243 bp fragments contain two well-conserved COE motifs, which are required for expression in DA1-7/DB neurons. The 395 bp fragment contains 7 LIN-39 sites (1–7, represented as black vertical lines), while the 243 bp fragment contains 3 LIN-39 sites (5-7), two of which are required for reporter gene expression in DA1-7/DB neurons. MUT indicates deletion of the LIN-39 site or that the COE motif has been mutated through substitution of 2 nucleotides in the core sequence (for example, COE wild-type site: TCCCNNGGGA >> COE MUT site: TGGCNNGGGA). Empty blue or black boxes indicate that the COE motif and LIN-39 sites are mutated, respectively. Representative images of animals carrying unc-129_243 bp::YFP and unc-129_243 bp LIN-39 sites 6 and 7 MUT::YFP are shown in C and detailed quantification is provided in D. Error bars represent standard deviation (STDV). ***p value < 0.001. N > 20 per transgenic line. (E–F) Cis-regulatory analysis of the del-1 locus. The COE3 motif in the 1827 bp fragment is required for expression in VA and VB neurons, while we have previously shown that mutation of the COE2 does not affect expression in VA and VB neurons (Kratsios et al., 2015). The 488 bp fragment is sufficient to drive reporter gene expression in VA and VB neurons. Apart from the COE3 motif, the 488 bp fragment contains 7 LIN-39 sites (1–7, represented as black vertical lines) that are predicted with p value < 0.005. Unlike the combined deletion of LIN-39 sites 5 and 6, deletion of the LIN-39 site 4 (del-1_488 bp LIN-39 site 4 MUT::YFP) results in a statistically significant loss of reporter gene expression in VA and VB neurons in two out of three transgenic lines. Detailed quantification is provided in F. Error bars represent standard deviation (STDV). ***p value < 0.0001. N > 20 per transgenic line.
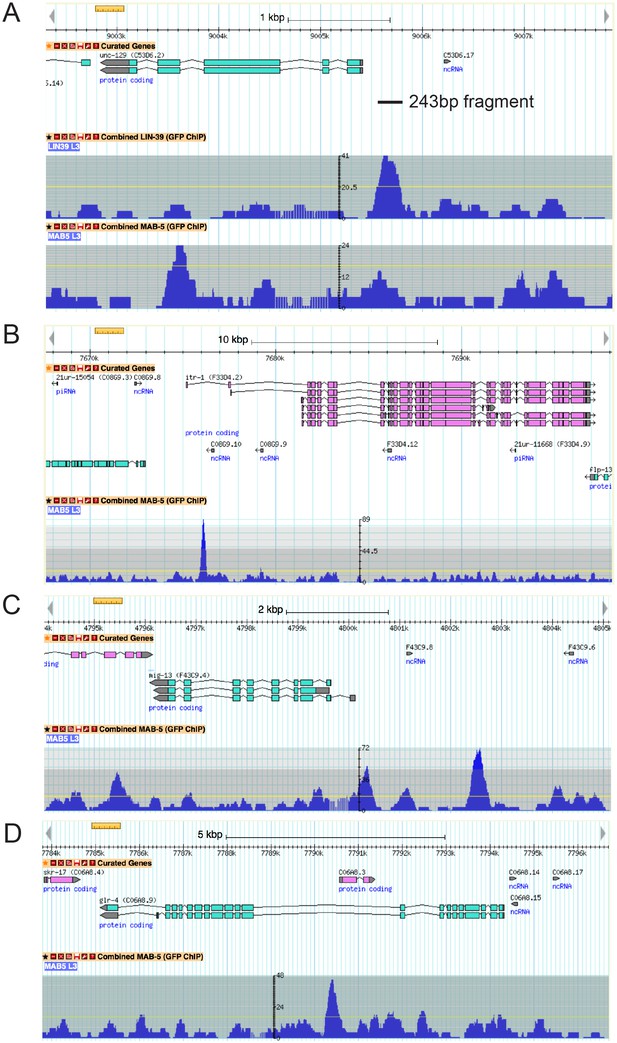
The Hox genes lin-39/Scr/Dfd/Hox4-Hox5 and mab-5 (Antp) bind directly to the cis-regulatory region of MN subclass-specific genes.
(A) Genome browser view of the unc-129 locus (www.wormbase.org). ChIP-seq for LIN-39 reveals binding at the cis-regulatory region of unc-129 (243bp fragment shown as a black solid line), which contains several LIN-39 sites that were functionally tested in Fig.6. Binding (although smaller peak) is also observed for MAB-5 on the unc-129 243bp region. (B-D): Genome browser view of itr-1, mig-13 and glr-4 loci (www.wormbase.org). ChIP-seq for MAB-5 shows that it binds directly to the cis-regulatory region of the MN subclass-specific genes itr-1, mig-13, and glr-4 which are de-repressed in DA8 neurons of mab-5 mutants. For A-D, data were generated through the modENCODE project and deposited into WormBase, from which the images presented here were downloaded.
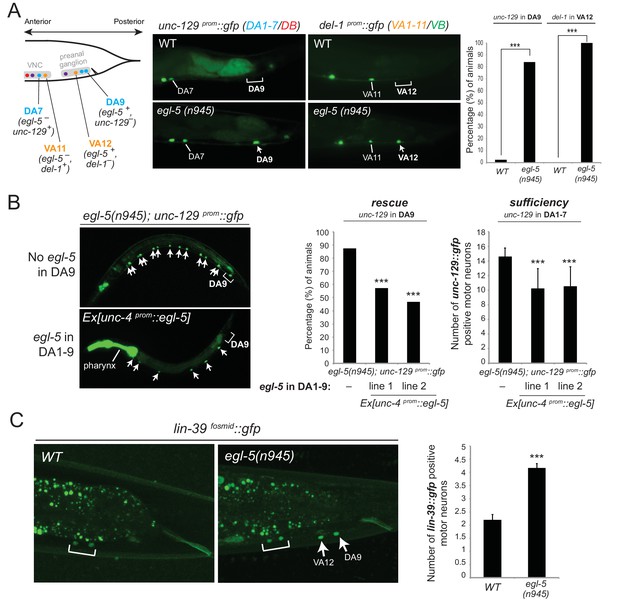
Evidence for homeotic activities of egl-5/Abd-B/Hox9-Hox13 in motor neuron subclasses.
(A) Schematic showing that the subclass-specific markers unc-129 (DA1-7/DB) and del-1 (VA1-11/VB) are not expressed in DA9 and VA12 of WT animals, respectively. In egl-5(n945) mutants, unc-129 is de-repressed in the DA9 neurons, while the expression of del-1 is de-repressed in VA12 neurons. DA9 and VA12 were identified based on their axonal trajectory and cell body position. Quantification (percentage of animals) is provided on the right. ***p value < 0.001. N > 25. (B) Mis-expression of egl-5 in all 9 DA neurons (DA1-9) (using a fragment of the unc-4 promoter, Ex [unc-4prom::egl-5]) results in partial rescue of unc-129 de-repression in DA9 of egl-5 mutants. Quantification for egl-5 rescue (percentage of animals) is provided on the right. ***p value < 0.001. In addition, mis-expression of egl-5 in all 9 DA neurons results in repression of unc-129 expression in anterior DA neurons (DA1-7), demonstrating that EGL-5 is also sufficient to repress unc-129 expression. The number of DA1-7 and DB neurons that show gfp expression was quantified in two different transgenic lines Ex [unc-4prom::egl-5] line 1(kasEx1) and 2(kasEx2). Transgenic animals were identified based on the co-injection marker (myo-2prom::gfp), which drives gfp expression in the C. elegans pharynx. Error bars represent standard deviation (STDV). N > 15. (C) The expression of lin-39 is de-repressed in DA9 and VA12 of egl-5(n945) mutants. White bracket indicates two VNC MNs that express lin-39. Quantification is provided on the right. ***p value < 0.001. N > 10.
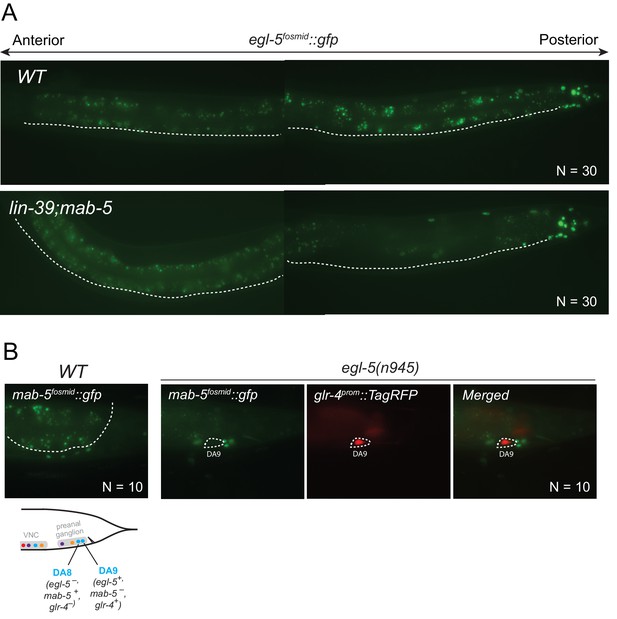
Analysis of potential Hox cross-regulatory interactions.
(A) The expression of egl-5 expression is not affected (not de-repressed in VNC MNs) in lin-39(n1760);mab-5(e1239) double mutants. N indicates number of animals. White dotted line in A and B delineates the ventral boundary of the intestinal cells, which are autofluorescent in the gfp channel. (B) The expression of mab-5 is not de-repressed in DA9 of egl-5(n945) mutants. The DA9 marker in red [glr-4prom::TagRFP] was crossed into egl-5(n945); mab-5fosmid::gfp animals. No mab-5fosmid::gfp expression was observed in DA9 neurons of egl-5(n945) mutants. Schematic is shown to summarize mab-5 and egl-5 expression in DA8 and DA9 neurons.
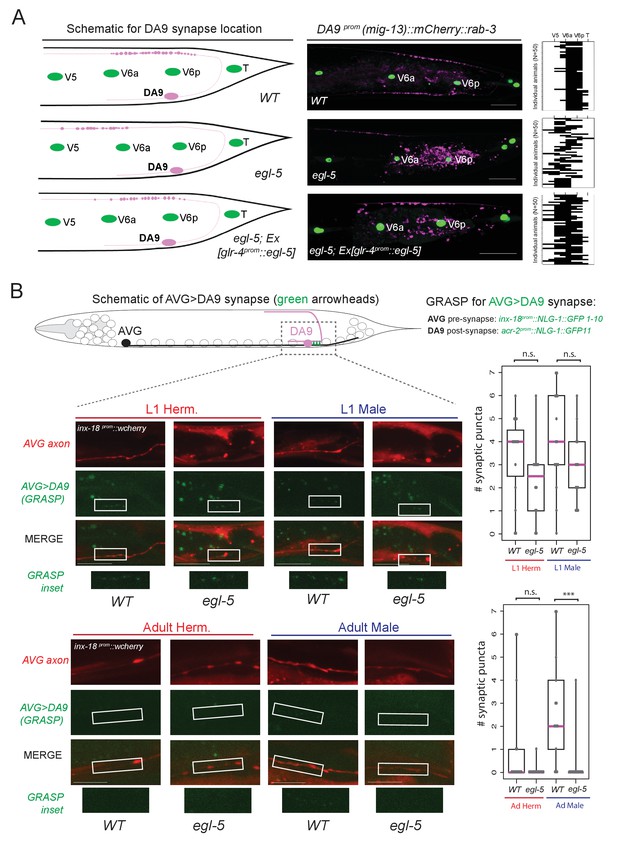
egl-5/Abd-B/Hox9-Hox13 affects synaptic wiring of the DA9 neurons.
(A) Schematic showing the location of DA9 neuromuscular synapses in WT, egl-5(n945), egl-5(n945); Ex [glr-4prom::egl-5] animals. On the right, the pre-synaptic boutons of the DA9 synapses were visualized using mig-13 prom::mCherry::rab-3. Since mig-13 expression is partially affected in DA9 by egl-5 (Figure 4E), we quantified the location of presynaptic boutons only in egl-5 mutant animals in which mig-13 expression was unaffected by the absence of egl-5. To quantify the location of the DA9 neuromuscular synapses (data provided on the right of each image), the seam cells (V5, V6a, V6p, T) were used as a reference since their location is not affected in egl-5(n945) mutants. It is noted that the gene promoter used for rescue (glr-4) is also under the partial control of egl-5 in DA9 (Figure 4E). For the heat maps shown on the right N = 50. Each row represents a single animal. P value = 0.0238 for egl-5(n945) and egl-5(n945); Ex [glr-4prom::egl-5] comparison. (B) Schematic of AVG>DA9 synapse, which is male-specific in the adult stage (see also Figure 1B). Synaptic inputs from AVG to DA9 were visualized with GRASP in C. elegans males and hermaphrodites at the first larval (L1) and adult stages and found to be defective in egl-5(n945) mutant male adult animals. The otEx6342 (inx-18prom::nlg-1::GFP1-10, acr-2 prom::NLG-1::GFP11, inx-18 prom::wCherry) transgene was used to visualize the AVG>DA9 synapse with gfp and the AVG axon with wCherry. Quantification of fluorescent micrographs was performed by quantifying the number of synaptic puncta observed using GRASP (AVG>DA9) in L1 and adult hermaphrodites and males. ***p<0.001; n.s., not significant. Magenta horizontal bars represent the median, black boxes indicate quartiles, black lines indicate range. Each gray dot represents one quantified animal. Region of observed synaptic puncta marked with white boxes. Scale bar, 10 µm.
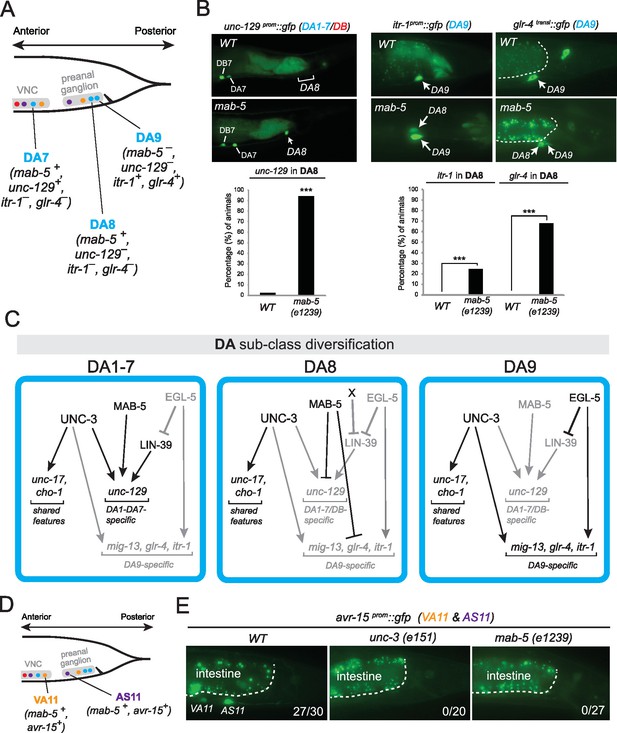
The Hox gene mab-5/Antp/Hox6-Hox8 is required for DA and AS subclass diversification.
(A) Schematic summarizing the expression of mab-5 and several subclass-specific markers (unc-129, itr-1, glr-4) in DA7, DA8 and DA9 neurons of WT animals. (B) The expression of the DA1-7/DB marker unc-129 is de-repressed in DA8 neurons of mab-5 (e1239) animals. The WT image of unc-129prom::gfp is repeated from Figure 7A. The DA9 markers itr-1 and glr-4 are de-repressed in DA8 neurons of mab-5 (e1239) animals. Quantification (% of animals) is provided. DA8 was identified based on its axonal trajectory and cell body position. ***p value < 0.001. N > 30. (C) Genetic model that summarizes the function of mab-5 in DA8 neurons, which appears distinct from the role of mab-5 in DA1-7 neurons. (D) Schematic showing the expression of mab-5 and avr-15/GluR, a subclass-specific gene expressed in VA11 and AS11, in posterior MNs. (E) The terminal selector unc-3 and the Hox gene mab-5 control avr-15/GluR expression in VA11 and AS11 neurons. Numbers at bottom right of each image indicate the fraction of animals that showed avr-15/GluR expression in VA11 and AS11. The white dotted line indicates the limits of the intestinal cells.
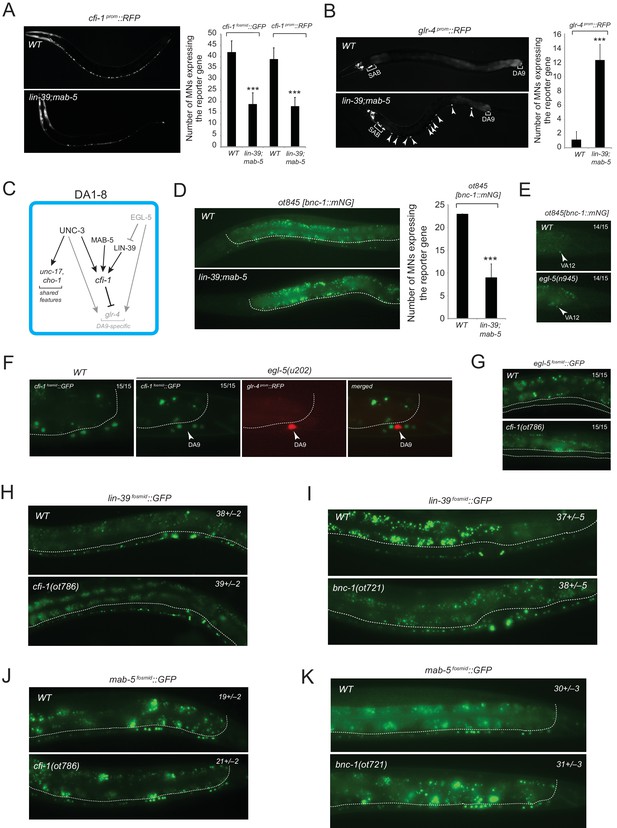
unc-3 and HOX also collaborate to control expression of intermediary regulatory factors.
(A) The expression of cfi-1 (based on transcriptional and a fosmid-based reporter) is affected in VNC MNs (including DA1-8) of lin-39(n1760);mab-5(e1239) mutants (L4 stage). Quantification on the right (N = 15). Error bars represent standard deviation (STDV). ***p value < 0.001. (B) The terminal differentiation gene glr-4 is de-repressed in DA1-8 (and probably DB) neurons of lin-39(n1760);mab-5(e1239) mutants (L4 stage). Quantification on the right (N = 15). Error bars represent standard deviation (STDV). ***p value < 0.001. (C) Genetic model summarizing how UNC-3 and HOX control the expression of the regulatory factor CFI-1 in DA1-8 neurons. Grey gene name indicates no expression; grey activation or inhibition arrow indicates that this genetic relationship does not take place in DA1-8 neurons. Compare with model in Figure 5E. (D) The expression of the transcription factor bnc-1 (based on a knock-in mNeonGreen (mNG) reporter allele ot845[bnc-1::mNG]) is affected in VA and VB2-11 MNs of lin-39(n1760);mab-5(e1239) mutants. Quantification on the right (N = 15). Error bars represent standard deviation (STDV). ***p value < 0.001. White dotted line in D (and F, H–K) indicates the ventral boundary of the nematode’s intestinal cells, which are autofluorescent in the gfp channel. Representative images of the anterior VNC are shown. (E) bnc-1 expression is unaffected (14 out of 15 animals) in VA12 neurons of egl-5(n945) mutants (L4 stage). Representative images of the preanal ganglion are shown. (F) cfi-1 expression is also unaffected (15 out of 15 animals) in posterior MNs of egl-5(n945) mutants (L4 stage). There is no co-localization of a red DA9 marker (glr-4prom::RFP) and cfi-1, indicating that cfi-1 is no-de-repressed in DA9 neurons of egl-5 mutants. (G) The posterior Hox gene egl-5 is not de-repressed along the VNC MNs of cfi-1(ot786) mutants (L4 stage). N = 15. Two white dotted lines indicate the location of VNC MN cell bodies. (H–K) The expression of both Hox genes, lin-39 (H–I) and mab-5 (J–K), is unaffected in VNC MNs of cfi-1(ot786) and bnc-1(ot721) mutant animals (L4 stage). N = 15. Numbers at top right show the average of gfp positive MNs ± standard deviation (STDV). The increased number of mab-5 expressing MNs in K compared to J is due to the larger region of the VNC imaged in K.
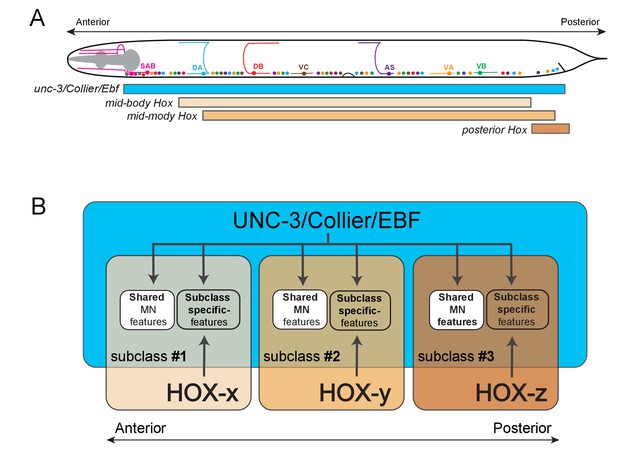
Model that conceptually summarizes the key findings of this paper.
(A) A schematic summarizing the intersectional expression of unc-3 and C. elegans Hox genes in MNs along the A-P axis. (B) Our findings reveal an intersectional strategy in which a non-regionally restricted, MN class selector gene (unc-3/Collier/Ebf) cooperates with a region-specific transcriptional code (HOX-x, HOX-y, HOX-z) to control the expression of MN subclass-specific features, providing a simple solution to the intriguing problem of how neuronal subclass diversity is generated along the A-P axis of the nervous system. Blue box indicates unc-3/Collier/Ebf expression in all MN classes along the A-P axis. Expression of distinct Hox genes (HOX-x, HOX-y, HOX-z) is presented with colored boxes of different shades of orange. The area of the intersection of unc-3/Collier/Ebf and Hox expression represents distinct MN subclasses (#1, #2, #3).
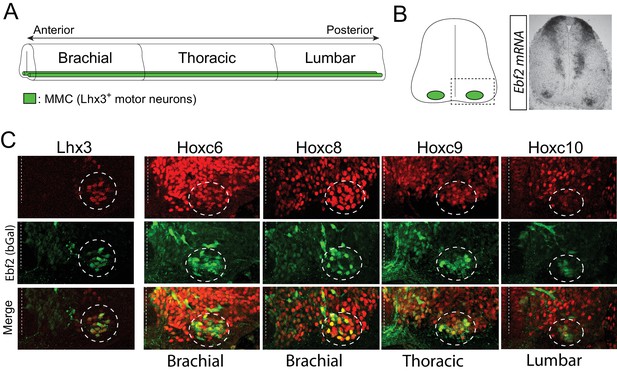
The unc-3 ortholog Ebf2 is co-expressed with distinct Hox genes in spinal motor neurons.
(A) Side view of the mouse spinal cord. Motor neurons of the medial motor column (MMC, shown in green) are located on the ventral side along the A-P axis of the spinal cord. Lhx3 is marker for MMC MNs. (B) Cross-section of the spinal cord. The dotted rectangular box indicates the region of the spinal cord that was imaged and shown in C. A representative image of in situ hybridization for Ebf2 on a cross-section of e13.5 spinal cord. (C) The unc-3 ortholog Ebf2 is expressed in MMC MNs as evident by double immunofluorescence staining. To characterize the Ebf2 expression pattern, we used a mouse line, which carries a promoterless lacZ cDNA cassette that replaces the first five exons of the endogenous Ebf2 locus (Corradi et al., 2003). By performing immunohistochemistry for β-galactosidase (product of LacZ cDNA and a proxy for Ebf2 expression), we find β-gal (Ebf2) expression, among other cells, in neurons at the ventral domain of the mouse spinal cord, a finding that corroborates our Ebf2 in situ result. Co-localization of green (antibody against β-gal/Ebf2) and red (antibody against Lhx3) fluorescence was observed in MMC MNs along the A-P axis of the spinal cord. Double immunofluorescence staining for β-gal/Ebf2 and distinct Hox proteins (Hoxc6, Hoxc8, Hoxc9, Hoxc10) reveals co-expression in MMC MNs at distinct regions of the mouse spinal cord (brachial, thoracic, lumbar).
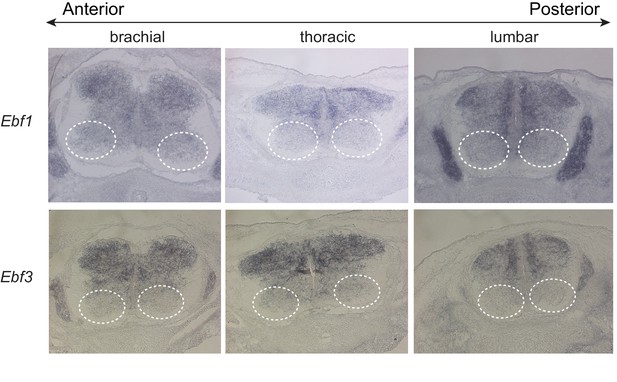
Expression analysis for Ebf1 and Ebf3 in mouse spinal motor neurons.
In situ hybridization analysis shows no detectable expression of Ebf1 and Ebf3 in spinal motor neurons from brachial, thoracic and lumbar regions at the embryonic day 14 (e14.5) of mouse embryo development. The white dotted circles indicate the location of MN cell bodies. Similarly, no Ebf4 expression was detected at e14.5 spinal MNs (data not shown).
Tables
Motor neuron subclass markers.
Gene | Function | Previously reported subclass expression | Confirmed subclass expression | Revised/new subclass expression | Stage for onset of expression (in hermaphrodites) | Sex-specificity of expression | UNC-3 dependency and COE motif number |
---|---|---|---|---|---|---|---|
del-1 | DEG/ENaC ion channel | VA1-VA12 VB1-VB11 SABVL/R* | VA1-VA11 VB1-VB11 SABVL/R† | VA1-VA11: late L2‡ VB1-VB11: early L2 SABVL/R: 3-fold embryo | Yes, in males VA12 also express del-1 | Yes (3 motifs) | |
unc-129 | TGF-beta like molecule | DA1-DA7, DB1-DB7, SABD§ | DA1-DA7, DB1-DB7, SABD# | DA1-DA7, DB1-DB7, SABD: L1 | No | Yes (3 motifs) | |
hum-2 | unconventional myosin | DB1¶ | DB1** | DB1: L1 or earlier | No | N. D (1 motif) | |
bnc-1 | C2H2 Zn finger transcription factor | VA1-VA12, VB2-VB11, SABVL/R†† | VA1-VA12, VB2-VB11, SABVL/R‡‡ | VA1-VA12, VB2-VB11, SABVL/R: late L1 | Yes, CP7 and CP8†† in male VNC | Yes7 (4 motifs) | |
cfi-1 | ARID-type transcription factor | DA1-DA8§§, DB1-DB7 | DA1-DA8##, DB1-DB7 | VA2-VA11, VB3-VB11## | DA1-DA8: not determined VA2-11, VB3-VB11: late L1 | Not determined | Yes (>5 motifs) |
avr-15 | Glutamate-gated cloride channel | DA9, VA12¶¶ | VA11, AS11*** | VA11, AS11: late L3 | Yes, 4 additional neurons (not ACh) in male PAG | Yes (4 motif) | |
flp-18 | FMRF-like neuropeptide | none | VA11 VA12††† | VA11: L2/L3 VA12: L2/L3 | Yes, 8 additional cells/neurons in male tail | Yes (1 motif) | |
ser-2 | tyramine receptor | DA9‡‡‡ | DA8§§§ | DA8: L1 or earlier | No | No (0 motifs) | |
mig-13 | transmembrane protein | DA9, VA12### | DA9 VA12¶¶¶ | DA9: L1 or earlier VA12: L2 | Yes, 2 additional ACh tail neurons | Yes (1 motif) | |
glr-4 | Glutamate receptor subunit | none | DA9**** | DA9: L1 or earlier | No | Yes (2 motifs) | |
itr-1 | Inositol triphosphate receptor | PDA, DA9†††† | DA9†††† | DA9: L2/L3 | No | Yes (1 motif) | |
sra-36 | G-protein coupled receptor | none | DA8 DA9 VA11‡‡‡‡ | DA8, DA9: L1 or earlier VA11: L2 | No | N. D (2 motifs) |
-
*Reagent: wdIs3 X or wdIs6 II [del-1prom::gfp]; Winnier et al., 1999.
-
†Expression was confirmed in VA1-11, VB1-11, and SABVL/R based on cell body position, axonal trajectory, and expected number of neurons for each class. Also, see Figure 2C.
-
‡As described in Winnier at al. (1999), by the end of L2, del-1prom::gfp is visible in a few anterior VAs. This expression progresses in a wave from anterior to posterior VAs, with all VAs (except VA12) expressing del-1prom::gfp by the L4/adult stage.
-
§Reagent: evIs82B IV [unc-129prom::gfp]; Colavita et al. (1998), Kratsios et al. (2015)
-
#Expression was confirmed in DA1-7, DB1-7, and SABD based on cell body position, axonal trajectory, cell type-specific markers, and expected number of neurons for each class. Also, see Figure 2C.
-
¶Reagent: mdIs123 [hum-2prom::gfp] from James Rand and Stephen Fields; www.wormatlas.org
-
**Expression was confirmed in DB1 based on cell body position and axonal trajectory. Also, see Figure 1B.
-
††Reagent: bnc-1 (ot845[bnc-1::+mNG+AID]) CRISPR allele; Kerk et al., 2017.
-
‡‡Expression was confirmed in VA1-VA12, VB2-VB11, SABVL/R based on cell body position and expected number of neurons for each class.
-
§§Reagent: otEx6502 [cfi-1fosmid::gfp]; Kerk et al., 2017.
-
##Expression was confirmed in DA1-8 based on cell body position and the cell-type specific markers juIs14[acr-2::gfp] that labels DA, DB, VA, VB. This analysis also revealed that cfi-1 is not expressed in VA12. No expression in VA1, VB1 and VB2 was inferred based on cell body position and total number (3) of MNs expressing cfi-1 at the retrovesicular ganglion.
-
¶¶Reagent: adEx1299[avr-15prom::gfp]; Dent et al., 1997.
-
***Expression was confirmed in AS11 based on cell body position, axonal trajectory, and cell type-specific markers. Also, see Figure 9D–E and Figure 1-figure supplement 1 .
-
†††Reagent: ynIs59[flp-18prom::gfp] from Chris Li. Expression in VA11 and VA12 was confirmed with cell type-specific markers. Also, see Figure 1B, Figure 2A–B, and Figure 1—figure supplement 1.
-
‡‡‡Reagent: otIs107 [ser-2prom1::gfp]; Tsalik et al., 2003.
-
§§§Expression in DA8 was confirmed with cell type-specific markers. Also, see Figure 1B and Figure 1—figure supplement 1.
-
###Reagent: muIs42[mig-13prom::MIG-13::gfp]; Sym et al., 1999, Klassen and Shen (2007)
-
¶¶¶Expression in DA9 and VA12 was confirmed with cell type-specific markers. Also, see Figure 2A–B.
-
****Reagent: otIs476[glr-4prom::tagrfp]. Expression in DA9 was confirmed with cell type-specific markers. Also, see Figure 1—figure supplement 1.
-
††††Reagent: otIs453 [itr-1prom::gfp]. Plasmid used for otIs453 transgene is pBT001, which was generated by Gower et al., 2001 and gfp expression in C.elegans in DA9 and PDA is described in Gower et al., 2001 and Klassen and Shen (2007). Expression was confirmed in DA9 using cell type-specific markers. Also, see Figure 2A–B and Figure 1—figure supplement 1.
-
‡‡‡‡Reagent: sEx11976[sra-36prom::gfp]. Expression in VA11, DA8 and DA9 was confirmed with cell type-specific markers. Also, see Figure 1B and Figure 1—figure supplement 1. †, #, **, ‡‡, ##, ¶¶, †††, §§§, ###, ****, ††††See also ‘Motor Neuron Subclass Identification’ section in Materials and methods.
-
N. D: Not determined.