Molecular architecture underlying fluid absorption by the developing inner ear
Figures
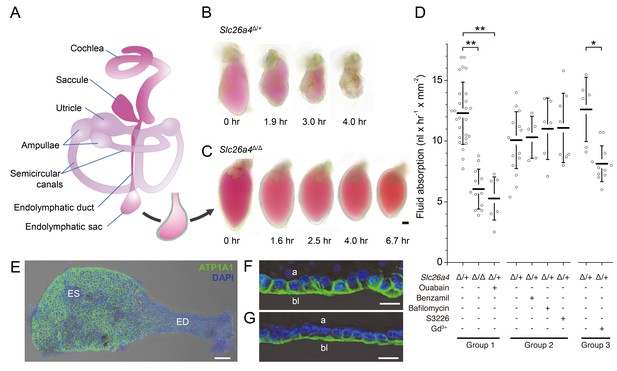
Fluid absorption in isolated endolymphatic sacs of Slc26a4Δ/+ and Slc26a4Δ/Δ mice.
(A) Schematic diagram of the membranous labyrinth illustrating the location of the endolymphatic sac and other structures of the inner ear. (B–C) Endolymphatic sacs isolated from E14.5 Slc26a4Δ/+ and Slc26a4Δ/Δ mice were filled with the fluorescent dye SNARF-1 and repeatedly imaged by laser-scanning microscopy at the indicated time points. SNARF-1 has a strong absorption in the visible range causing the lumen to be colored red. Note that the sac from the Slc26a4Δ/+ mouse diminished in size faster than the sac from the Slc26a4Δ/Δ mouse. The luminal volume was recorded at each time point with 3D confocal microscopy to determine the rate of fluid absorption (Figure 1—figure supplement 1A). The bar represents 50 µm. (D) Summary of data obtained in three groups of experiments. Group 1: Rate of fluid absorption in E14.5 Slc26a4Δ/+ (n = 26), E14.5 Slc26a4Δ/Δ (n = 12) and E14.5 Slc26a4Δ/+endolymphatic sacs in the presence of ouabain (n = 7). Group 2: Rate of fluid absorption in E14.5 Slc26a4Δ/+endolymphatic sacs filled with control (n = 13), benzamil (n = 5), bafilomycin (n = 6) or S3226 solution (n = 8). Group 3: Rate of fluid absorption in E14.5 Slc26a4Δ/+endolymphatic sacs filled with control (n = 6) or gadolinium solution (n = 11). **p<0.001, *p<0.008. (E) Maximum intensity projection image of a whole-mounted E15.5 Slc26a4∆/+ endolymphatic sac labeled by anti-ATP1A1 antibody (green) and stained with DAPI (blue). Note the higher levels of anti-ATP1A1 signal in endolymphatic sac (ES) compared to the endolymphatic duct (ED). Scale bar = 50 µm. (F,G) Optical cross-sections of the epithelium in the endolymphatic sac (F) and endolymphatic duct (G). Note that anti-ATP1A1 signal is located in basolateral (bl) but not apical (a) membranes. Bars represents 10 µm.
-
Figure 1—source data 1
Rates of fluid absorption in endolymphatic sacs of E14.5 Slc26a4Δ/+ and Slc26a4Δ/Δ mice.
- https://doi.org/10.7554/eLife.26851.004
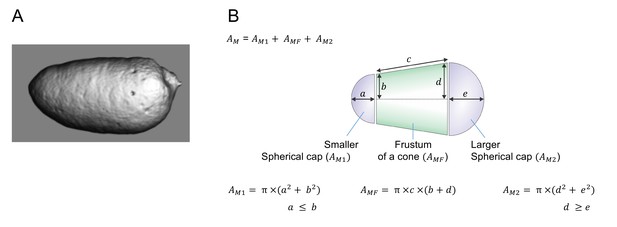
Geometric approximation of the luminal surface of isolated endolymphatic sacs.
(A) 3D reconstruction of the luminal volume of an endolymphatic sac isolated from an E14.5 Slc26a4Δ/Δ mouse. The lumen was stained with the fluorescent dye SNARF-1 and observed by 3D confocal microscopy. (B) Luminal volumes of isolated endolymphatic sacs were modeled to consist of two spherical caps enclosing a frustum of a cone. Five linear measurements were taken to estimate the luminal surface (A") according to the provided formulas.
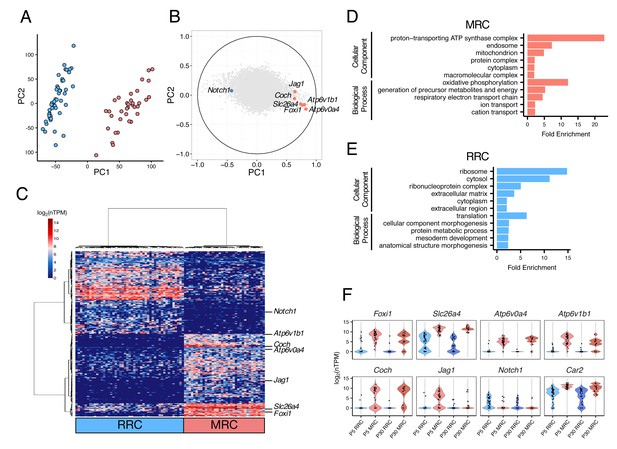
Single-cell RNA-seq enables unbiased clustering of postnatal endolymphatic sac epithelial cells.
(A) Plot of single-cell transcriptomes of 82 P5 endolymphatic sac epithelial cells (captured on three C1 IFCs) projected onto the first two principal components (PCs) calculated by PCA using all expressed genes. Cells clearly separated into two groups. (B) Correlation circle map on PC1 and PC2. Each point corresponds to a gene and the known cell markers are highlighted in color. These results suggest that PC1 mainly contributes separation of MRCs and RRCs. (C) Hierarchical clustering of 82 P5 cells (x-axis) using the top 300 genes (y-axis) that are highly correlated with PC1 positively or negatively. Two distinct clusters of cells were identified and assigned into MRCs and RRCs based upon the expression of the known markers. (D–E) Functional enrichment analysis of gene ontology (GO) terms was performed for 594 differentially expressed genes in MRCs (MRC genes) and 426 differentially expressed genes in RRCs (RRC genes) at P5 (false discovery rate: FDR < 0.05, specificity score >0.5, Figure 2—source data 2) from the Gene Ontology Consortium website. Only annotations of PANTHER GO-Slim dataset with the Bonferroni corrected p-value of <0.05 and fold-enrichment >2 are shown. (F) Violin plots of canonical MRC and RRC genes. Four known EVA genes and other previously reported marker genes were differentially expressed between P5 MRCs and P5 RRCs, or between P30 MRCs and P30 RRCs (Figure 2—figure supplement 1). Although Slc26a4 was significantly highly expressed in P5 and P30 MRCs, a subset of RRCs express Slc26a4 at low levels (Figure 2—figure supplement 2). Jag1 and Notch1 were not differentially expressed at P30. Black dots show the expression level for each cell.
-
Figure 2—source data 1
Summary of numbers of cells captured and sequenced.
- https://doi.org/10.7554/eLife.26851.008
-
Figure 2—source data 2
Cell-type specific genes identified by differential expression analysis.
- https://doi.org/10.7554/eLife.26851.009
-
Figure 2—source data 3
List of TaqMan® gene expression assays.
- https://doi.org/10.7554/eLife.26851.010
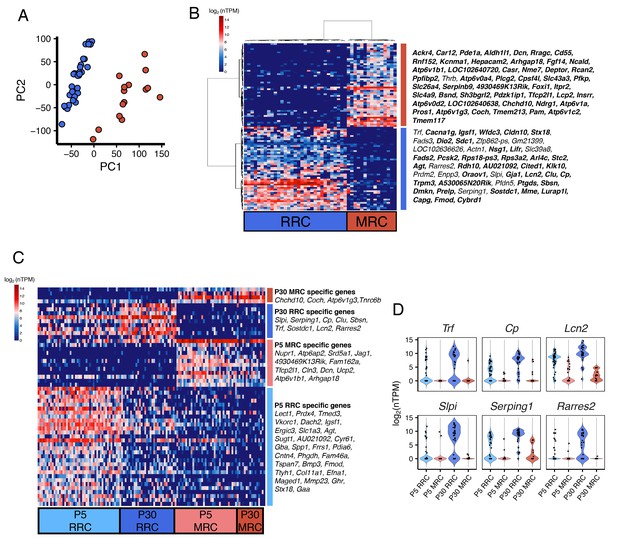
Unbiased clustering of P30 endolymphatic sac epithelial cells.
(A) Plot of single-cell transcriptomes of 47 P30 endolymphatic sac epithelial cells (captured on two C1 IFCs) projected onto the first two PCs calculated by PCA using all expressed genes. (B) Hierarchical clustering of 47 P30 cells (x-axis) using the top 100 genes (y-axis) that are highly correlated, positively or negatively, with PC1. As with the P5 results, cells and genes are clustered to two groups. Genes in each cluster are listed in order from top to bottom in the heatmap. Genes identified as differentially expressed at P5 are shown in bold. (C) A heatmap of differentially expressed genes across P5 RRCs, P5 MRCs, P30 RRCs, and P30 MRCs (FDR < 0.05, specificity score >0.65). Genes are listed in decreasing order of specificity score. (D) Violin plots of representative genes significantly highly expressed in P30 RRCs.
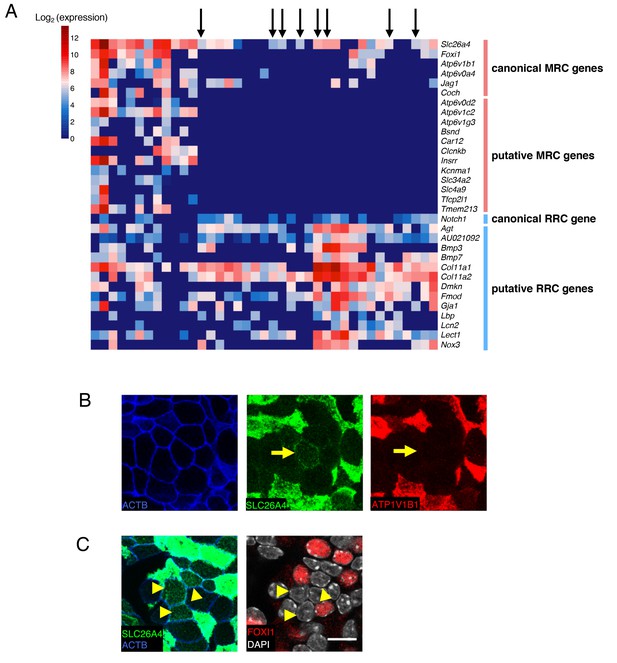
A subset of RRCs express pendrin at low levels.
(A) Hierarchical clustering of P5 single-cells (captured on two C1 IFCs) using qPCR data generated on the BioMark HD platform with TaqMan gene expression assays. Based on the P5 single-cell RNA-seq results, 18 MRC genes and 13 RRC genes were selected in an arbitrary manner for analysis. Thirty-nine P5 cells were captured with two C1 IFCs. Expression level is displayed as log2 (expression), which is equivalent to the difference between the limit of detection Ct value and the measured Ct value. There are several cells (black arrows) expressing Slc26a4 but not other MRC genes. (B) Confocal images of apical membranes of whole-mount P5 endolymphatic sac epithelium co-labeled with anti-SLC26A4 (green) and anti- ATP6V1B1 (red) antibodies. The yellow arrows indicate a cell that expresses SLC26A4 but not ATP6V1B1. (C) Two representative images at different levels (apical membrane and cytosol) from a z-stack of whole-mount P5 endolymphatic sac epithelium co-labeled with anti-SLC26A4 (green) and anti-FOXI1 (red) antibodies. Yellow arrowheads indicate cells that express SLC26A4 but not FOXI1. Original magnification, 63x. Scale bars, 10 μm.
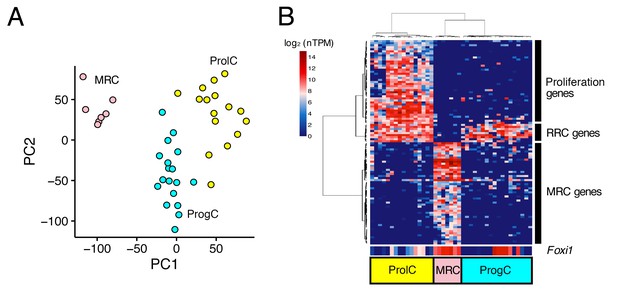
Unbiased clustering of E16.5 endolymphatic sac epithelial cells.
(A–B) Plots show PCA (A) and hierarchical clustering (B) of 41 E16.5 cells (captured on two C1 IFCs). Cells are clustered into three groups, which are color-coded and labeled as MRCs (pink), progenitor cells (ProgCs, light blue), and proliferating cells (ProlCs, yellow). Seven MRCs were segregated from other cells by PC1. The top 100 genes with the highest correlation to PC1 were used for hierarchical clustering. The genes are clustered into three groups, which are assigned to MRC genes, RRC genes, and proliferation genes (29 out of 39 genes are associated with the GO term ‘cell cycle’ (GO:0007049)). Foxi1 is not specific to MRCs at E16.5.
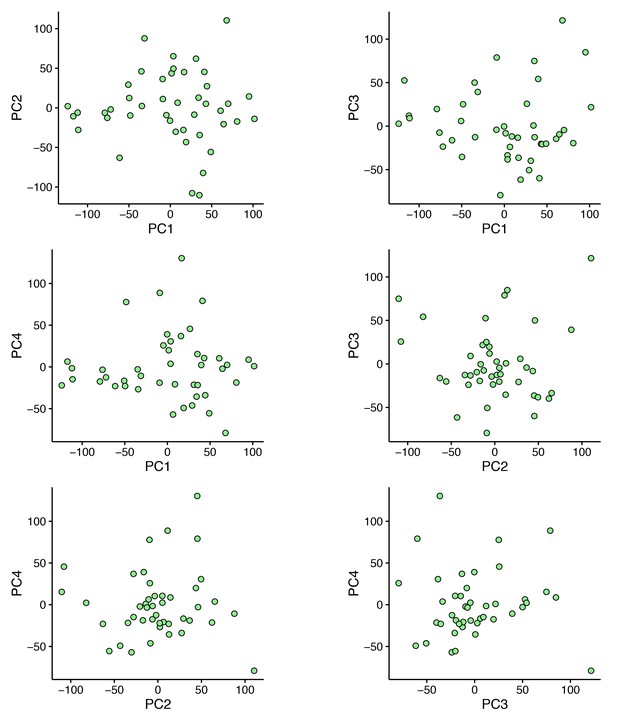
Principal component analysis of 44 E12.5 cells.
The first four principal components do not segregate any distinct cell groups.
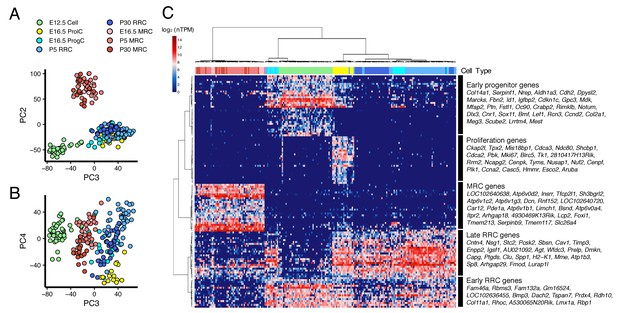
Cross-age transcriptome analysis resolves cell differentiation pathway of endolymphatic sac.
(A–B) PCA plots of 213 endolymphatic sac epithelial cells onto PC2, PC3 and PC4. Each cell has already been annotated and color-coded by prior analyses (Figures 2 and 3, Figure 2—figure supplement 1). PC2, PC3, and PC4 contribute to segregation of MRCs, E12.5 cells and ProlCs from others, respectively. (C) Hierarchical clustering of the 213 cells using the 123 genes with positive or negative correlation to PC2 or PC3, or with negative correlation to PC4. The same color-codes as those used in (A–B) were shown (top color bar). Four MRC genes, LOC192640638, LOC102640720, Lcp2, and 4930469K13Rik, are located adjacent to Foxi1 on chromosome 11 (Figure 4—figure supplement 1).
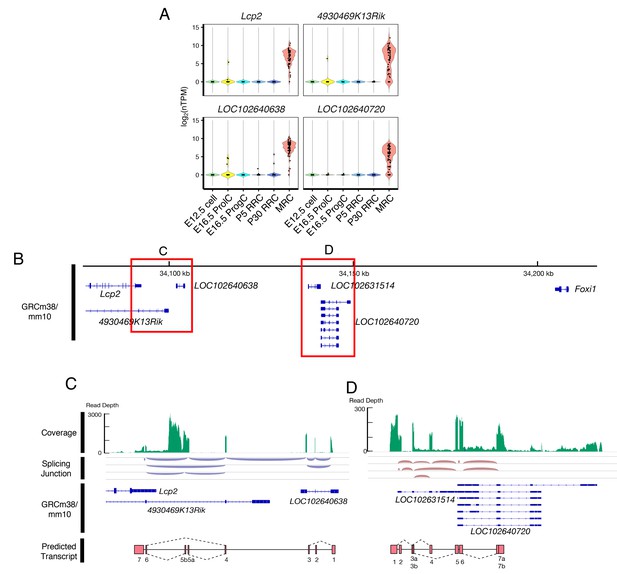
Potential MRC-specific lncRNAs located adjacent to Foxi1.
(A) Violin plots of four genes whose expression is highly specific to MRCs. (B) These four genes are located adjacent to Foxi1. (C–D) Representative coverage and splicing tracks of the RNA-seq library prepared from 150 pooled P5 cells for the regions enclosed by red rectangles in (B). Reads are aligned only to the 3’-UTR in Lcp2 but not to other exons of Lcp2. Based upon splicing junction tracks, two transcripts are predicted that include some alternative splicing isoforms.
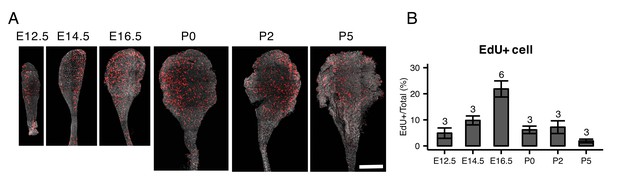
EdU staining of whole mount endolymphatic sacs during development.
(A) Average intensity projection of whole mount endolymphatic sacs from E12.5 to P5 labeled by EdU (red) and DAPI (grey). Original images were captured as a tiled z-stack with confocal microscopy. (B) The percentages of EdU-labeled cells in the endolymphatic sac epithelia. Values are expressed as mean ± SD and the total number of embryo analyzed is indicated above each bar plot.
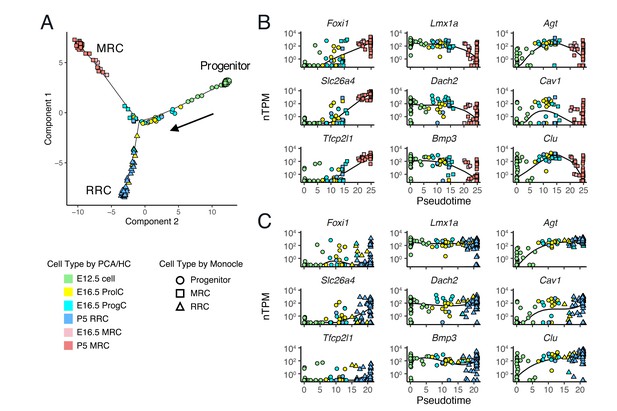
Single-cell trajectory analysis of E12.5 to P5 cells using Monocle 2.
(A) The plot shows single-cell expression profiles of differentially expressed genes (FDR < 0.01) across time points in a two-dimensional component space. The unsupervised ordering method reconstructs a branched trajectory from progenitors to MRCs (squares) or RRCs (triangles). Cell types assigned by PCA and hierarchical clustering (HC) are color-coded. Note that a subset of E16.5 ProgCs, E16.5 ProlCs, and P5 RRCs are clustered into the branch of MRCs. (B–C) Expression levels of representative MRC genes (Foxi1, Slc26a4, Tfcp2l1), early RRC genes (Lmx1a, Dach2, Bmp3), and late RRC genes (Agt, Cav1, Clu) in cells are ordered along pseudo-time. Two branches are shown separately: (B), progenitor to MRC; and (C), progenitor to RRC. Kinetic curves of two branches are shown as solid lines.
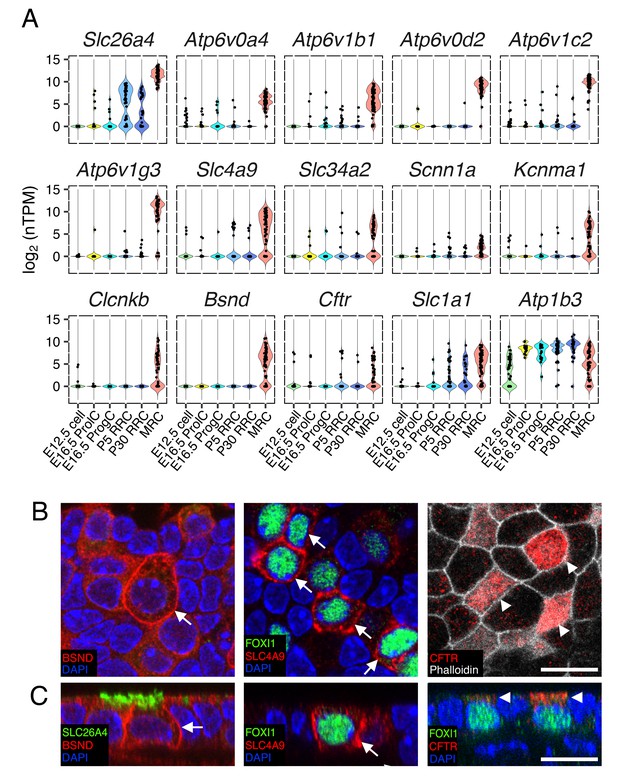
Ion transporter/channels in the endolymphatic sac epithelia.
(A) Violin plots of representative genes differentially expressed in MRCs or RRCs (Table 1). (B–C) Immunohistochemisty validation of BSND (Barttin), SLC4A9 (AE4), and CFTR. Representative images from z-stacks of whole mount endolymphatic sac epithelia at P5 are shown in (B) and reconstructed cross-sections are shown in (C). BSND and SLC4A9 localize to basolateral membranes (arrows) and CFTR localizes to apical membranes (arrowheads). Scale bars, 10 µm.
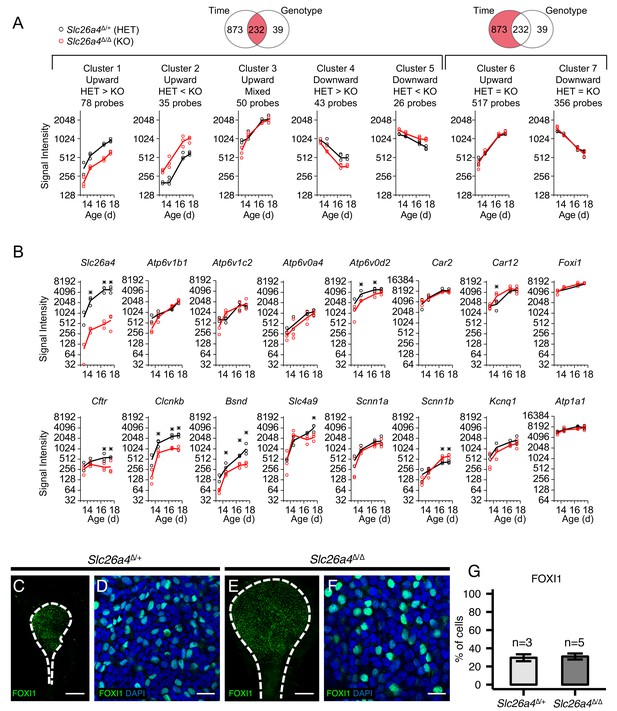
Gene expression profiles of embryonic endolymphatic sacs from Slc26a4∆/+ and Slc26a4∆/∆ mice.
(A) Differential expression analysis and subsequent clustering by temporal expression patterns. Regression-based analysis identified 232 probes with significant differences across time points and between genotypes, and also identified 873 probes only with significant difference across time points. The two significant probe groups are clustered to five and two clusters, respectively, using hierarchical clustering (Figure 8—source data 2). Each point (circle or triangle) represents the median signal intensity of multiple probes per biological replicate and each line connects the mean values of replicates. (B) Expression levels of ion transport genes in endolymphatic sacs at ages E13.5, E14.5, E16.5 and E17.5. Each point (black or red circle) represents the signal intensity of a biological replicate and each line represents the mean of the replicates. Expression of all genes increased significantly with age in Slc26a4Δ/+ and Slc26a4Δ/Δ mice with the exception of the expression of Slc26a4 and Cftr in Slc26a4Δ/Δ mice. Significant differences in expression levels between genotypes are indicated with asterisks. (C, E) Maximum intensity projection images of whole mount endolymphatic sacs in P0 Slc26a4∆/+ and Slc26a4∆/∆ mice labeled by anti-FOXI1 antibodies (green). (D, F) Representative images of magnified Z-stacks of the whole mount endolymphatic sacs. Nuclei were stained with DAPI (blue). (G) Mean percentages of FOXI1+ cells per total cells. Error bars indicate SD. Scale bars, 250 µm (C, E); 20 µm (D, F).
-
Figure 8—source data 1
Quality and quantity of total RNA and quality metrics of gene array chips.
- https://doi.org/10.7554/eLife.26851.023
-
Figure 8—source data 2
Gene array analysis of embryonic endolymphatic sac in Slc26a4Δ/+ and Slc26a4Δ/Δ mice.
- https://doi.org/10.7554/eLife.26851.024
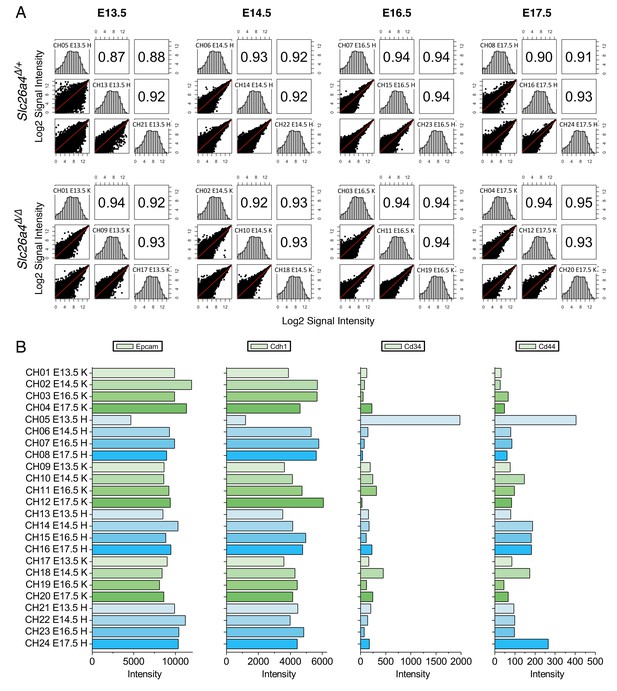
Microarray analysis of gene expression of endolymphatic sac.
(A) Correlation between three biological replicates for each time point. Scatter plot matrices of signal intensities from all 45,101 probes have histograms of intensity of each probe in the diagonal, and Pearson correlation coefficients in the upper part of the matrix. (B) Intensity of representative cell-type-specific markers. One replicate at E13.5 showed lower expression of epithelial cell markers Epcam and Cdh1 (E-cadherin) and higher levels of endothelial cell marker Cd34, suggesting this sample was contaminated. This sample was not included in further analyses.
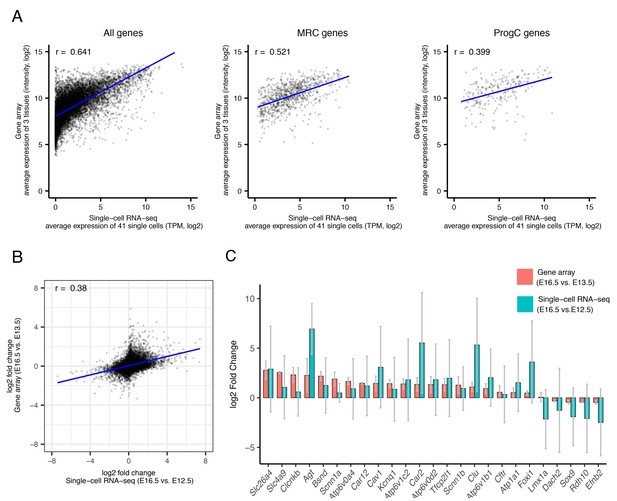
Comparative analysis of gene array and single-RNA-seq data.
(A) Comparison of average expression levels for three samples from E16.5 Slc26a4Δ/+endolymphatic sacs and for 41 single cells from E16.5 wild type endolymphatic sacs. Plotted is the correlation pattern for genes whose RNA was detected in both datasets (11,027 genes, left), E16.5 MRC genes (975 genes, center), or E16.5 ProgC genes (278 genes, right). Blue lines show linear regression. (B) Correlation in fold-changes (log2) in levels of expression between the two time points. (C) Changes of average expression for representative genes between two time points in each of the two methods. Error bars indicate SDs.
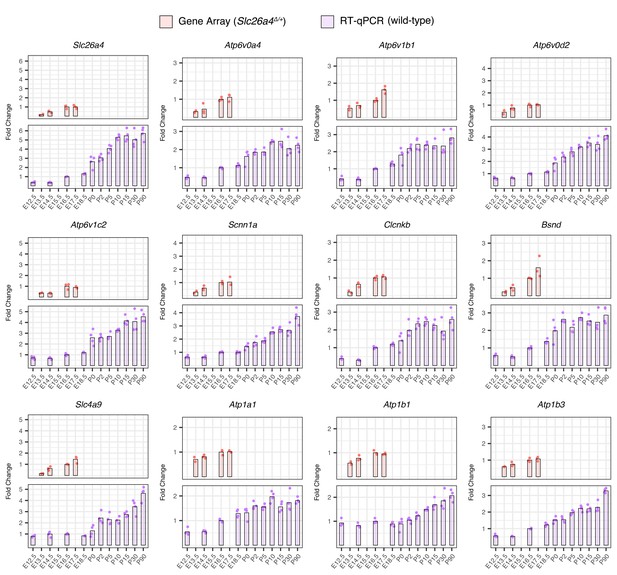
Temporal expression trends of gene array and RT-qPCR data from whole endolymphatic sacs.
Expression levels of representative ion-transport genes relative to mean values of E16.5 samples were calculated for gene array data from whole endolymphatic sacs of Slc26a4Δ/+ mice at ages E13.5 to E17.5 (top) and for RT-qPCR data from those of wild-type mice at ages E12.5 to P90 (bottom). Dots show the expression level of each sample (two replicates for gene array data at E13.5, three replicates for gene array data at other ages, and four replicates for RT-qPCR data at all ages). Bars show mean levels of replicates at each age.
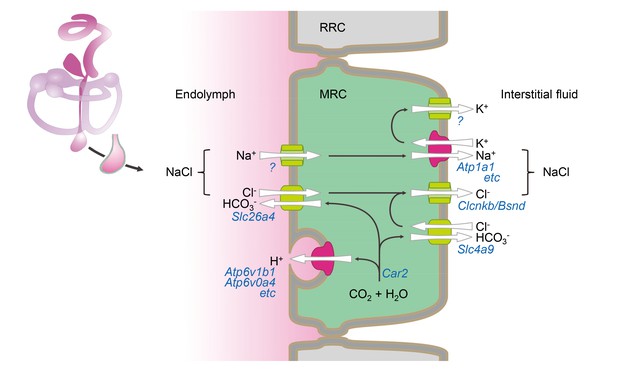
Hypothetical model of NaCl absorption by mitochondria-rich cells of the endolymphatic sac.
Mitochondria-rich cells (MRC) are interspersed among ribosomal-rich cells (RRC). Ion pumps including Na+ /K+ ATPase and v-ATPase are colored (red) different from channels and transporters (green). The direction of ion transport is indicated (arrows). Ion pumps, channels and transporters are annoted with their corresponding genes. Immunolocalization data reported by other published studies or our current study are summarized in Figure 9—source data 1.
-
Figure 9—source data 1
Summary of immunolocalization of proteins in mitochondria-rich cells.
- https://doi.org/10.7554/eLife.26851.026
Tables
Differentially expressed genes associated with ‘ion transmembrane transporter activity’
https://doi.org/10.7554/eLife.26851.018Cell type | Category | Gene symbol | Protein/Subunit |
---|---|---|---|
MRC | V-ATPase (V0 domain) | Atp6v0a4 | a4 (kidney, epididymis) |
Atp6v0b | b (c'') (ubiquitous) | ||
Atp6v0c | c (ubiquitous) | ||
Atp6v0d2 | d2 (kidney, epididymis) | ||
V-ATPase (V1 domain) | Atp6v1a | A (ubiquitous) | |
Atp6v1b1 | B1 (kidney, epididymis) | ||
Atp6v1c2 | C2 (lung, kidney, epididymis) | ||
Atp6v1e1 | E1 (ubiquitous) | ||
Atp6v1f | F (ubiquitous) | ||
Atp6v1g3 | G3 (kidney, epididymis) | ||
Atp6v1h | H (ubiquitous) | ||
Cl-/HCO3- exchanger | Slc26a4 | Pendrin | |
Na+/HCO3- cotransporter, Cl-/HCO3- exchanger | Slc4a9 | AE4 | |
Na+/phosphate cotransporter | Slc34a2 | NPT2B | |
Na+ channel | Scnn1a | ENaC α subunit | |
K+ channel | Kcnma1 | MaxiK/BK | |
Ca2+ channel | Itpr2 | ITPR2 | |
Cl- channel | Clcnkb | CLC-Kb | |
Bsnd | Barttin | ||
Cftr | CFTR | ||
Voltage-gated anion channel | Vdac1 | VDAC1 (porin) | |
Vdac2 | VDAC2 | ||
Integrin | Itgav | Integrin α-V | |
Na+/amino acid symporter | Slc1a1 | EAAT3 | |
L-amino acid transporter | Slc43a2 | LAT4 | |
Serinc2 | Serine incorporator 2 | ||
Serinc4 | Serine incorporator 4 | ||
Pyruvate transporter | Mpc1 | Mitochondrial pyruvate carrier 1 | |
ATP Synthase (F1 domain) | Atp5a1 | α | |
Atp5b | β | ||
Atp5o | O | ||
ATP Synthase (F0 domain) | Atp5f1 | B1 | |
Atp5g1 | C1 | ||
Atp5g3 | C3 | ||
Atp5h | D | ||
Atp5j | F6 | ||
Atp5l | G | ||
Cytochrome c oxidase | Cox5a | 5A | |
Cox6c | 6C | ||
Cox7b | 7B | ||
Cox7c | 7C | ||
Cox8a | 8A | ||
Ubiquinol-cytochrome c reductase | Uqcr10 | Subunit 9 | |
Uqcr11 | Subunit 10 | ||
Uqcrb | Subunit 7 | ||
Uqcrfs1 | Subunit Rieske | ||
Uqcrh | Subunit 6 | ||
RRC | Na+/K+ ATPase | Atp1b3 | Na+/K+ ATPase subunit β3 |
Ca2+ channel | Cacna1g | Cav3.1 | |
Cation channel | Trpm3 | TRPM3 | |
Cl- channel | Fxyd3 | FXYD3 (MAT-8) | |
Volume-sensitive anion channel | Lrrc8c | LRRC8C | |
Gap junction | Gja1 | GJA1 | |
Na+/amino acid sympoter | Slc1a3 | EAAT1 | |
H+/amino acid symporter | Slc36a3 | PAT3 |
Additional files
-
Source Code 1
R script for analysis and visualization
- https://doi.org/10.7554/eLife.26851.027
-
Transparent reporting form
- https://doi.org/10.7554/eLife.26851.028