Influenza virus recruits host protein kinase C to control assembly and activity of its replication machinery
Figures
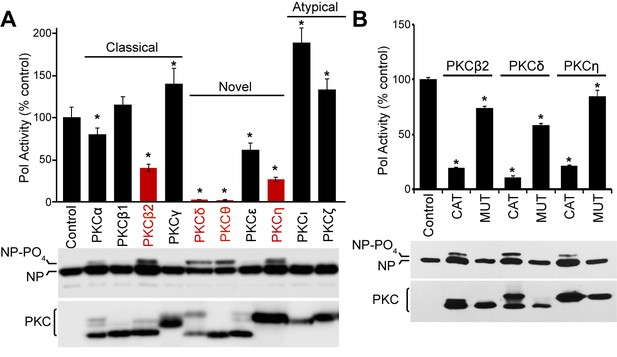
Constitutively active PKC phosphorylates NP leading to impaired influenza virus polymerase activity.
(A) Expression of constitutively active PKC impairs influenza virus polymerase activity. Polymerase activity assays were performed in 293T cells in the presence or absence of the catalytic domains from classical, novel or atypical PKC isoforms. Data were averaged and normalized to the empty vector control. NP and PKC were detected by western blotting whole cell lysate. A hyper-phosphorylated form of NP was detected in some conditions. (n=3 ± standard deviation, *p<0.05 one-way ANOVA when compared to the empty vector control). (B) Polymerase activity assays were performed in the presence of PKC catalytic domains, catalytically inactive mutants, or empty vector controls. Polymerase activity and protein expression were analyzed as in (A).
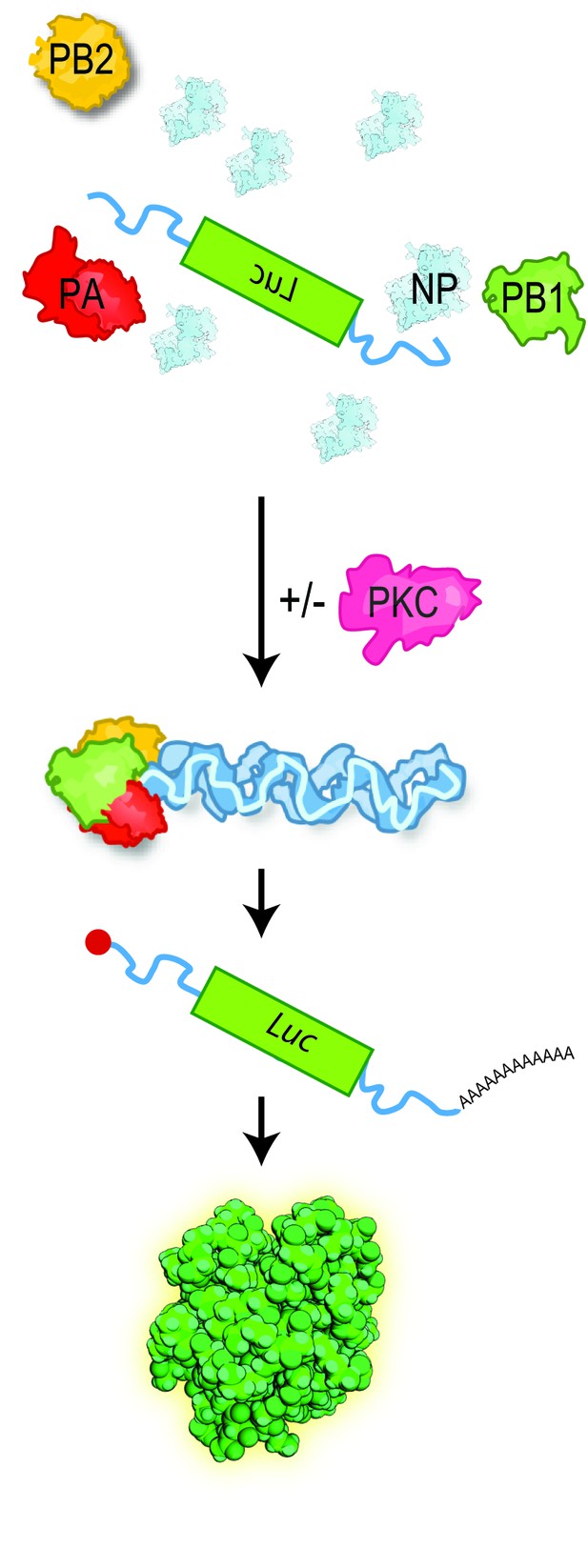
Polymerase activity assays.
Cells are transfected with plasmids expressing a viral genome segment encoding luciferase, NP, and polymerase proteins PB1, PB2, and PA in the presence or absence of PKC. Luciferase activity output is measured as a proxy for RNP assembly and polymerase activity.
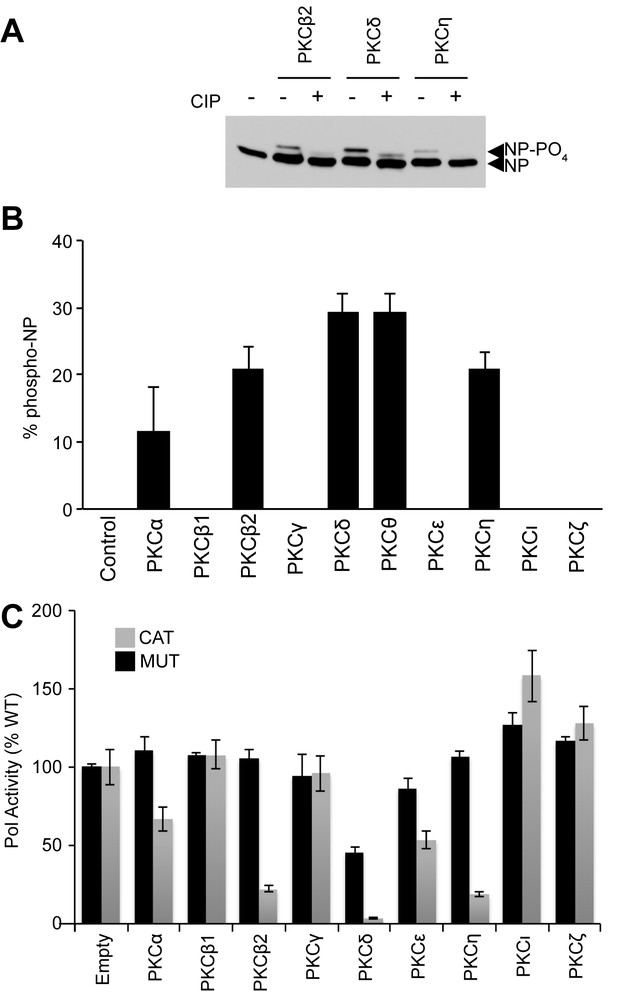
PKCs hyper-phosphorylate NP and only catalytically active enzymes inhibit viral polymerase activity.
(A) Whole cell lysates co-expressing NP with the indicated PKC catalytic fragments were treated with calf intestine alkaline phosphatase (CIP) or left untreated. Reaction products were analyzed by western blot with anti-NP antibody. (B) Quantification of NP phosphorylation in three separate replicates of the assay in Figure 1A (mean ±standard deviation). (C) Polymerase activity assays were performed in 293T cells co-expressing either PKC catalytic fragments (CAT) or mutants lacking catalytic activity (MUT) (n=3 ± standard deviation).
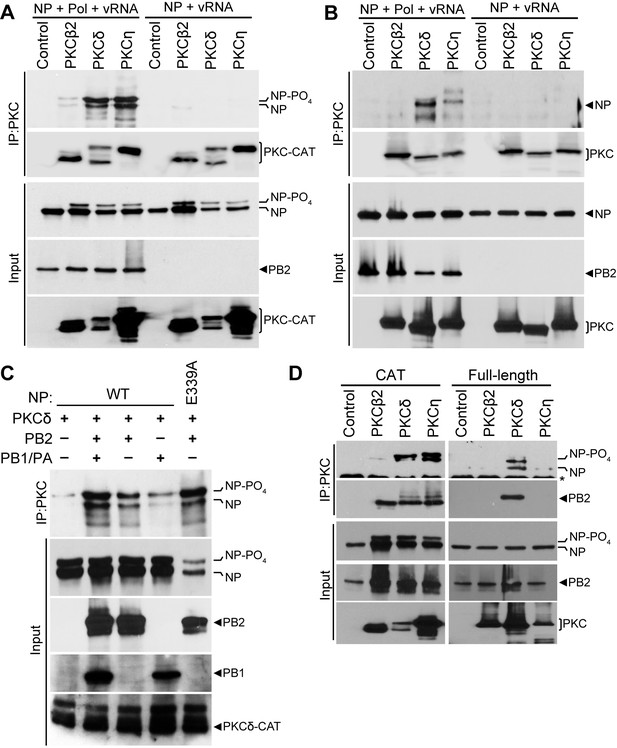
The polymerase subunit PB2 bridges stable interactions between PKCδand NP.
(A) Catalytic fragments or (B) full-length PKC isoforms were co-expressed with NP and vRNA in the presence or absence of the viral polymerase (Pol). PKC was immunoprecipitated from cell lysates and precipitated PKC and co-precipitated NP were detected by western blot. Input proteins were detected by blotting whole cell lysates for NP, PB2 and PKC. (C) To determine which polymerase subunits facilitate PKC binding, interaction assays were performed as above on cells expressing PKCδ, WT or oligomerization-defective NP (E339A), and the indicated combinations of polymerase proteins. (D) The minimal components needed for complex formation were tested by immunoprecipitating catalytic fragments or full-length PKCs from lysates where NP and PB2 were co-expressed. Immunoprecipitated and input proteins were detected by western blot.
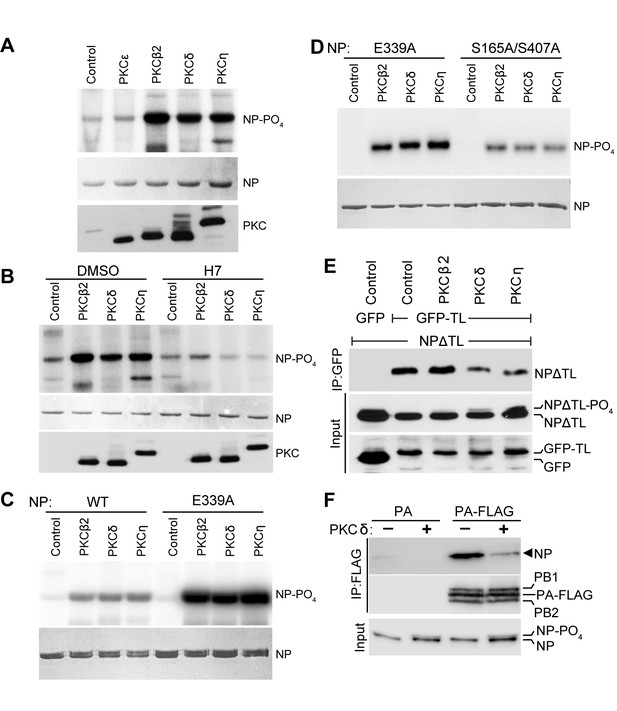
PKC regulates NP oligomerization by phosphorylating residues at the homotypic interface.
(A) Purified PKC phosphorylates NP in vitro. Catalytic fragments of PKC were immunoprecipitated from 293T cells and used for in vitro kinase assays with recombinant NP. Immunoprecipitates from cells transfected with empty vector were used as a negative control. Kinase reactions were resolved by SDS-PAGE followed by Coomassie staining to monitor NP levels and autoradiography to detect phosphorylation. PKC levels were measured by western blotting a portion of the immunoprecipitate. (B) In vitro kinase assays were performed with purified PKC and NP supplemented with the PKC inhibitor H7 or the vehicle control. Reaction products were analyzed as described in (A). (C–D) In vitro kinase assays were performed to identify NP residues phosphorylated by PKC, using (C) WT and oligomerization-defective NP (E339A) as substrate or (D) oligomerization-defective NP (E339A) and a double-alanine mutant of NP (S165A/S407A) as substrate. (E) PKC activity reduces NP tail loop:groove interactions. An NP deletion mutant lacking the tail loop (NPΔTL) and a tail loop fused to eGFP (eGFP-TL) were co-expressed in 293T cells in the presence or absence of exogenous PKCδ. NP tail loop:groove interactions were monitored by immunoprecipitating eGFP-TL and measuring co-precipitating NPΔTL by western blot. Expression levels of interacting partners were analyzed by blotting total proteins. (F) PKCδ activity impairs RNP assembly. RNPs were reconstituted in cells expressing a vRNA, PB1, PB2, NP and PA or PA-FLAG in the presence or absence of exogenous PKCδ. RNPs were captured by FLAG immunoprecipitation. Co-precipitating and input proteins were detected by blotting NP or the polymerase proteins.
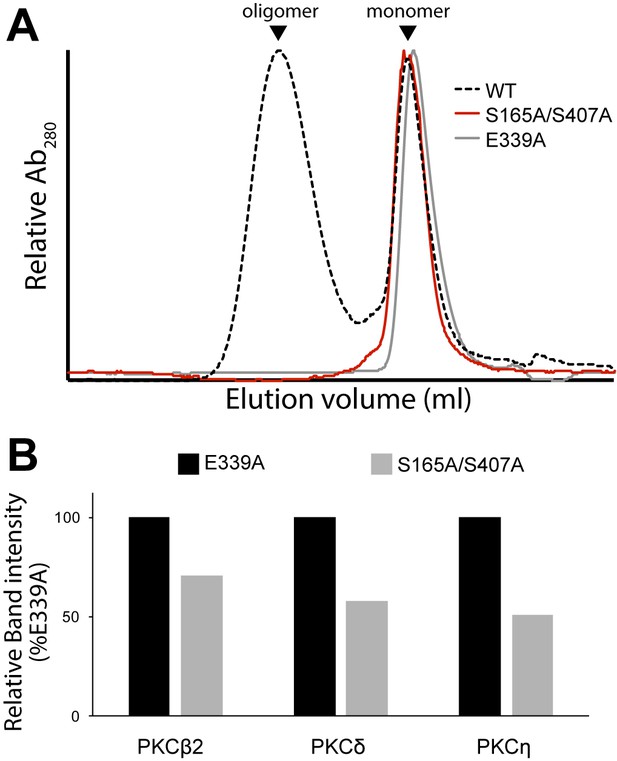
PKC phosphorylates NP S165 and S407.
(A) Size exclusion chromatography of recombinant NP shows purified NP mutants exist as a homogenous monomeric population compared to WT NP that exists as a mixture of monomer and multimer. (B) Quantification of in vitro kinase assay products reported in Figure 2B shows mutation of NP S165 and S407 reduced phosphorylation by PKC. Data are normalized to NP E339A phosphorylation levels.
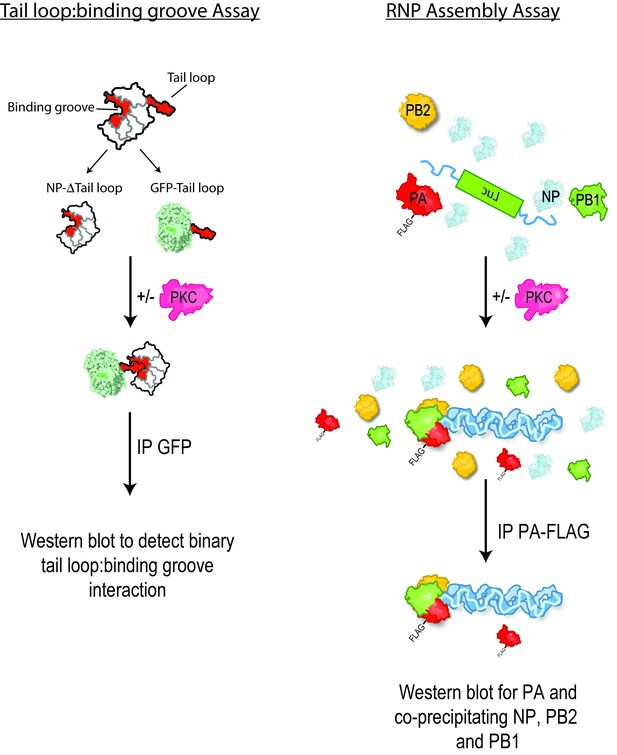
Protein interaction assays to measure NP:NP association and RNP assembly.
Schematic representation of experimental designs to measure binary NP:NP interactions via a tail loop:binding groove assay (left) and NP oligomerization via an RNP assembly assay (right).
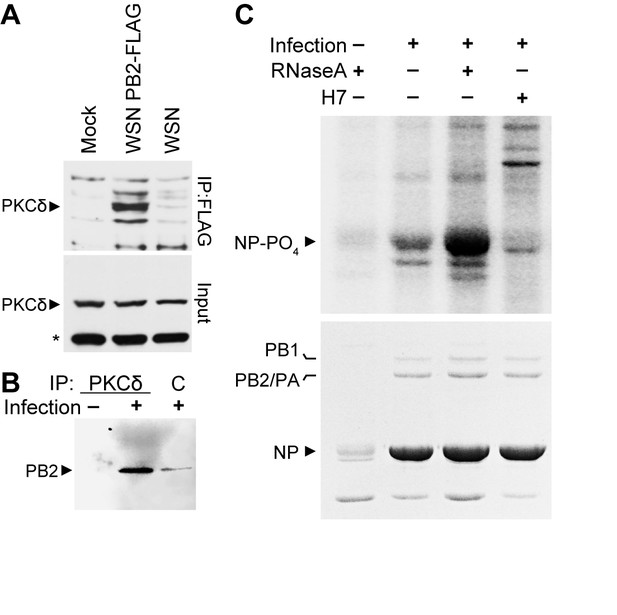
Polymerase-associated PKCδ directs NP phosphorylation during infection.
(A) A549 cells were infected with WSN, WSN PB2-FLAG, or mock treated. Cell lysates were subjected to anti-FLAG immunoprecipitation and blotted for PKCδ. Whole cell lysate were also probed for PKCδ. *=nonspecific cellular protein. (B) Infected or mock-treated A549 cell lysates were immunoprecipitated with anti-PKCδ antibodies or a non-specific control and analyzed by blotting for PB2. (C) PB2-FLAG was immunoprecipitated from infected A549 cells. Immunoprecipitates were used as a source of both kinase activity and substrate for in vitro kinase assays. Where indicated, immunoprecipitated complexes were treated with RNaseA or H7. Reactions were analyzed by SDS-PAGE followed by Coomassie staining and autoradiography. IgG was detected by Coomassie staining immunoprecipitated samples, including heavy chain in the uninfected control which co-migrates with NP.
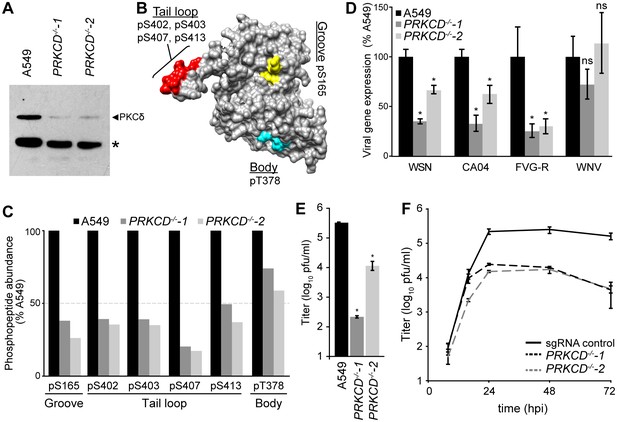
Knockout of PKCδ impairs NP phosphorylation and virus replication.
(A) Ablation of PKCδ expression in PRKCD-knockout A549 cells was confirmed by western blotting cell lysates from WT and two clonal knockout cell lines. Residual signal is due to low-level antibody cross-reactivity to PKCβ2 present in these cells (Figure 5—figure supplement 1C). *=nonspecific cellular protein. (B) Structure of NP (PDB: 2IQH) showing spatial organization of the PKCδ target sites identified by mass spectrometry. (C) Relative quantification of NP phosphorylation. Elution profiles of phosphopeptides identified by mass spectrometry were integrated to calculate the relative abundance of phosphorylation sites present in the groove (S165), tail loop (S402, S403, S407, S413), and body (T378) regions of the influenza nucleoprotein. (D–F) Loss of PKCδ impairs viral gene expression and replication. (D) Viral gene expression was measured in WT or PKCδ-knockout A549 cells infected with the indicated influenza reporter viruses or a West Nile virus (WNV) replicon. (E) Single-cycle virus replication measured 8 hpi in WT or PKCδ-knockout A549 cells infected with WSN virus. (F) Multi-cycle replication was measured in sgRNA control A549 cells or PKCδ-knockout cells. For all infections, data are shown as mean of n=3 ± standard deviation. *=p< 0.05 one-way ANOVA when compared to WT control. ns = not significant.
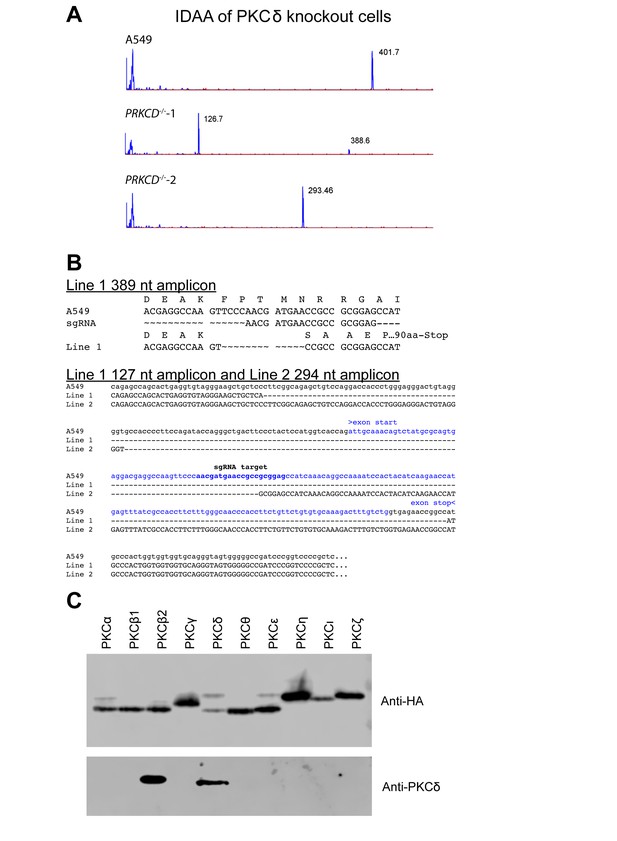
Identification of PRKCD−/− cells by Indel Detection by Amplicon Analysis (IDAA).
(A) IDAA performed on genomic DNA isolated from PRKCD−/−-1 and PRKCD−/−-2 clonal cell lines identified two amplicons containing deletions for line 1 and only one amplicon for line 2. Calculated size (nt) is indicated for each peak. (B) Mutations at each locus were defined by sequencing of the IDAA amplicon and alignment to the sequence obtained from WT A549 cells. (C) The PKCδ antibody cross-reacts with PKCβ2. Constitutively active PKCs were over-expressed as HA-tagged proteins and blotted with antibodies directed against HA or PKCδ. The PKCδ antibody (Santa Cruz sc-937 C20) detects both PKCδ and PKCβ2.
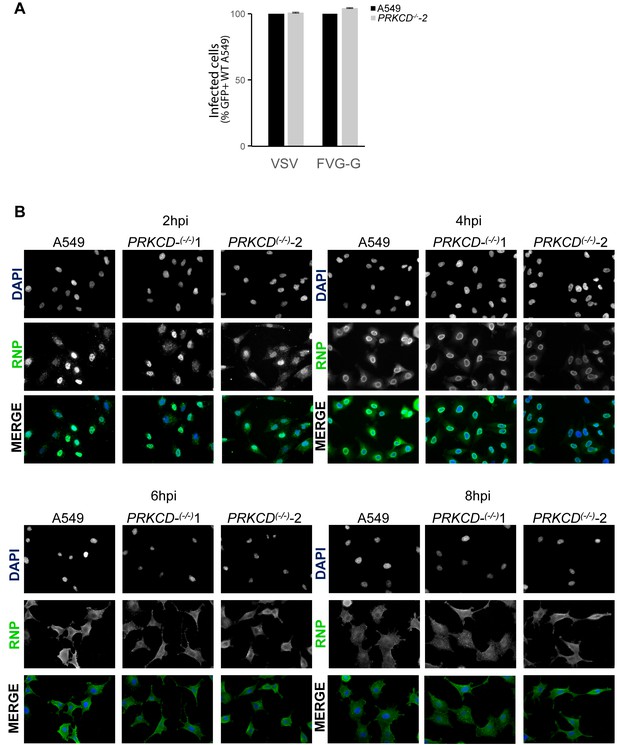
PKCδ is not required for entry via VSV-G or nucleo-cytoplasmic shuttling of NP.
(A) WT or PKCδ-knockout A549 cells were infected with GFP-expressing vesicular stomatitis virus (VSV) or a VSV-G pseudotyped influenza virus. The number of infected (GFP+) cells was quantified by flow cytometry and normalized to WT A549 cells. Data are averages from three independent experiments ± sd. (B) WT or PKCδ-knockout A549 cells were infected with WSN, fixed at the indicated time points, and NP localization was detected by immunofluorescence assay. Nuclei were counter-stained with DAPI.
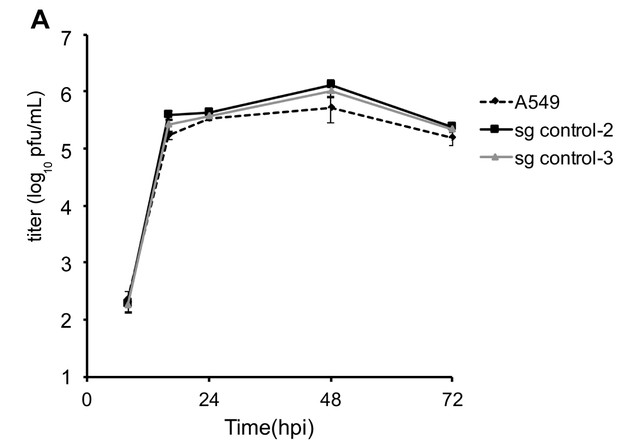
Influenza virus replication is unchanged in clonal lines encoding a non-targeting CRISPR-Cas system.
Two clonal cell lines were obtained expressing Cas9 and a non-targeting sgRNA. Multicycle replication kinetics were examined in WT or sg RNA control A549 cell lines by infecting cells with WSN at an MOI of 0.01. Viral titers were determined at the indicated time points by plaque assay (n=3 independent infections ± SD).
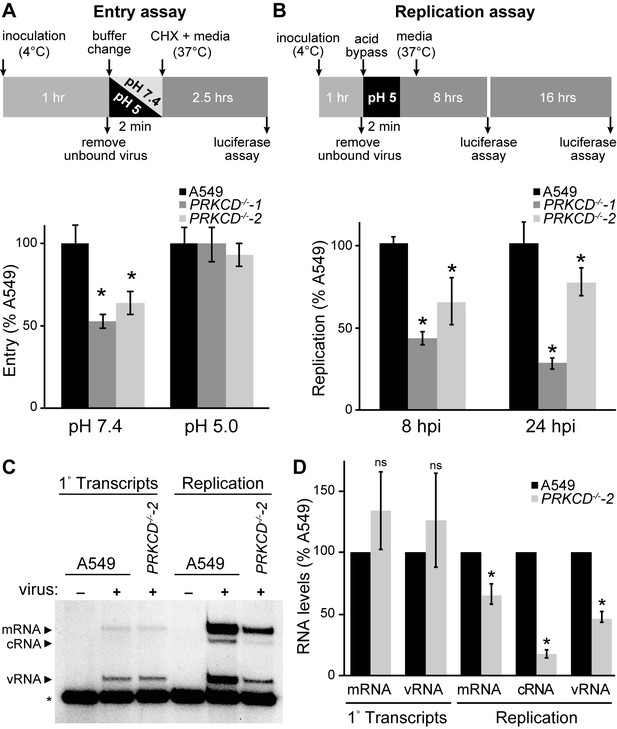
Loss of PKCδ specifically inhibits the transition to genome replication.
(A) PKCδ plays a role in entry. The experimental diagram shows attachment of bioluminescent influenza virions (PASN) to cells at 4˚C followed by normal receptor-mediated endocytosis (pH 7.4) or ‘acid bypass’ at the plasma membrane by exposure to low pH buffer (pH 5). Following entry, cells were treated with cycloheximide for 2.5 hr and entry was quantified. Data were normalized to WT cells for each condition. (B) To eliminate the effects of PKCδ on viral entry, infections with the transcription-dependent reporter virus (PASTN) were initiated by acid bypass as diagramed. Replication was quantified at 8 and 24 hpi. Data were normalized to WT cells for each condition. (C) and (D) Loss of PKCδ impairs replication but not primary transcription. (C) Primary transcription from incoming RNPs and replication by de novo-assembled RNPs were measured by primer extension assays in cells infected via acid bypass. To measure only primary transcription, cells were treated with cycloheximide (left). A non-specific product (*) served as a loading control. (D) Quantification of mRNA, cRNA and vRNA bands detected during primary transcription or replication in three independent primer extension experiments, normalized to WT A549 cells. For all experiments, data are mean of n=3± standard deviation. *p<0.05 one-way ANOVA when compared to WT A549 cells. ns = not significant.
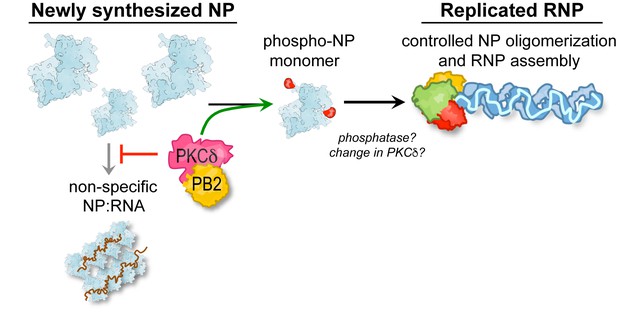
A model for PKC phospho-regulation of NP oligomerization and RNP assembly.
PKCδ binds to the polymerase subunit PB2 to phosphorylate newly synthesized NP and negatively regulate its homo-oligomerization. This process is proposed to establish a pool of monomeric NP that is competent to specifically assemble with genomic RNA into viral RNPs, while also reducing premature non-specific assembly of NP:RNA aggregates. Our model further raises the possibility that additional factors ultimately license NP oligomerization and help potentiate successful genome replication.
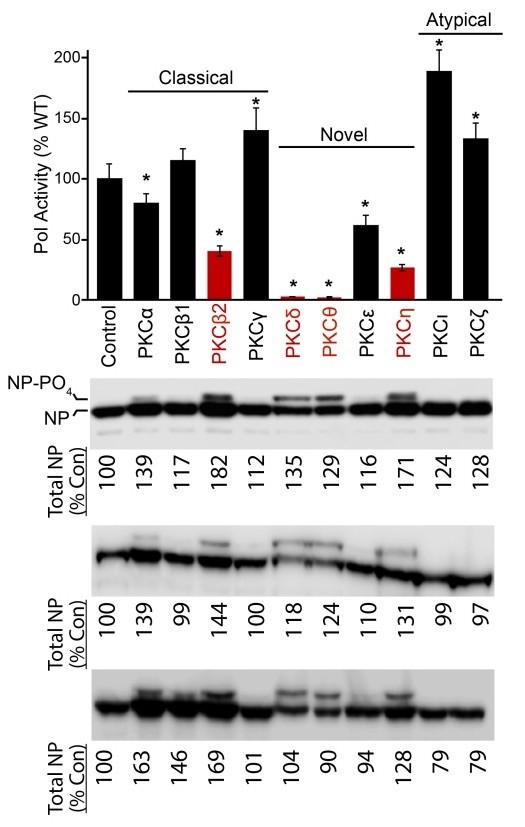
NP levels were quantified for data presented in Figure 1A.

PB2 interacts with PKCδ in the absence of NP. PKCδ and PB2 variants were expressed in 239T cells.
Tables
PKCδ is activated during influenza virus infection.
https://doi.org/10.7554/eLife.26910.011Position | Phospho (STY) Probabilities | Position in peptide | Charge | Mass error [ppm] |
---|---|---|---|---|
S302 | RS(0.52)DS(0.378)AS(0.097)S(0.005)EPVGIYQGFEK | 2 | 3 | 0.87569 |
S304 | RS(0.003)DS(0.995)AS(0.002)SEPVGIYQGFEK | 4 | 2 | 0.51199 |
-
Phosphoproteomic analysis was performed by mass spectrometry on lysates prepared from A549 cells at 6 hr post-inoculation. Phosphopeptides corresponding to PKCδ autocatalytic modifications at residues S302 and S304 were identified. Localization probabilities for each phosphosite are shown in parentheses.
Domain boundaries and mutations for PKC variants.
https://doi.org/10.7554/eLife.26910.018PKC isoform | CAT domain (aa) | DN mutation |
---|---|---|
PKCα | 326–672 | K368R |
PKCβ1 | 329–671 | K371R |
PKCβ2 | 329–673 | K371R |
PKCδ | 338–697 | K380R |
PKCε | 334–674 | K376R |
PKCη | 342–683 | K384R |
PKCι | 239–592 | K281M |
PKCζ | 232–587 | K273M |
-
Boundaries and mutations for PKC expression constructs: PKC catalytic fragments (CAT) are defined by the first and last amino acid residues in the expression construct. Dominant negative (DN) mutations were created by introducing the indicated mutation.