Circular oligomerization is an intrinsic property of synaptotagmin
Figures
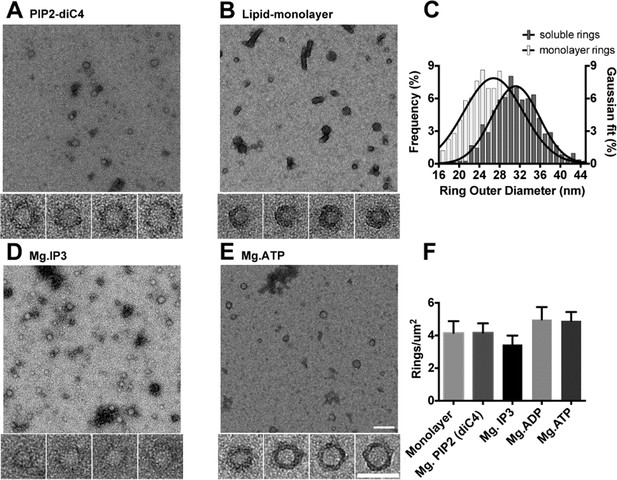
The C2AB domains of Syt1 oligomerize into ring-like structures in solution when triggered with naturally occurring polyphosphates and Mg2+.
Negative staining electron microscopy analysis shows that Syt1C2AB forms ring-like structures in buffer solution containing soluble PIP2 (PIP2-diC4) and 1 mM free Mg2+ (A), and these rings are very similar to those observed on monolayers containing 40% DOPS (B). (C) The average diameter of the Syt1C2AB soluble ring-oligomers are slightly but not significantly larger (32 ± 3 nm) compared to the rings (28 ± 2 nm) formed on lipid monolayer. These oligomeric rings were not unique to PIP2-diC4 and other polyvalent anions, like IP3 (D) and ATP (E) also induced the oligomerization of Syt1C2AB. The prevalence and stability of the Syt1C2AB were similar for all the polyvalent anions tested (F). The scale bar represents 100 nm in the main figure and 50 nm for the insets (Panels A, B, D, E). Average values and standard deviations from a minimum of 3 independent experiments are shown.
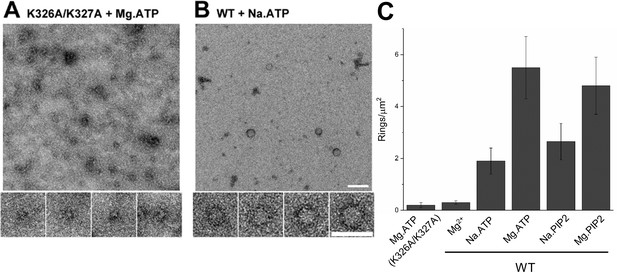
(A) Molecular interaction of polyvalent anion with the C2B polybasic is strictly required for the oligomer formation. Negative stain EM analysis shows that disrupting the polylysine motif on the C2B domain (K326A/K327A) prevents the oligomer formation even in the presence of polyphosphates and Mg2+. (B and C) Mg2+ is a critical co-factor that stabilizes the ring oligomers triggered by polyphosphates (ATP or PIP2) as complete removal of Mg2+ (Na.ATP or Na.PIP2) results in reduction in the density of the ring oligomers.
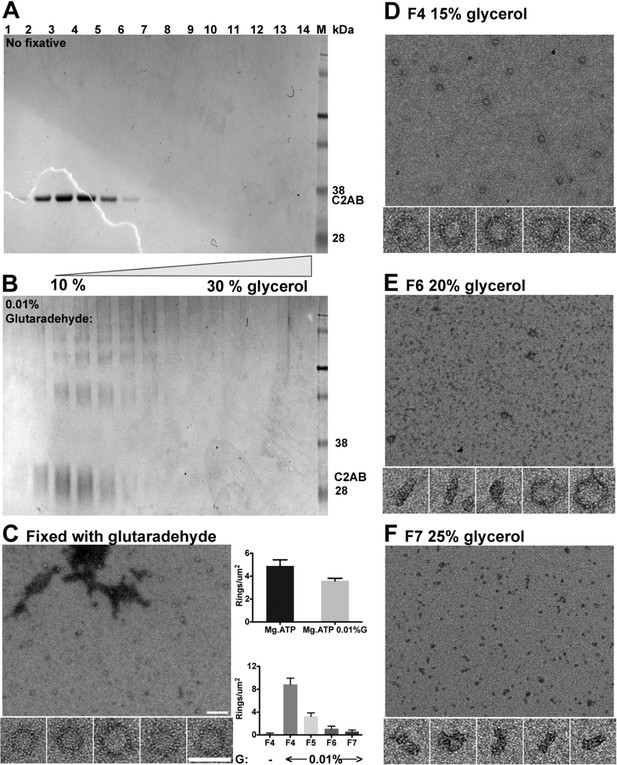
Glycerol density gradient was used to purify the soluble Syt1C2AB ring oligomers.
The Syt1C2AB oligomers were triggered with 1 mM ATP and Mg2+. The ring oligomers are not stable during purification and thus, and no oligomers were isolated under native conditions (A). However, the ring oligomers are stabilized with a small amount of fixative (0.01% glutaraldehyde) and can be isolated at ~15% glycerol gradient as visualized by negative stain EM (C–F).
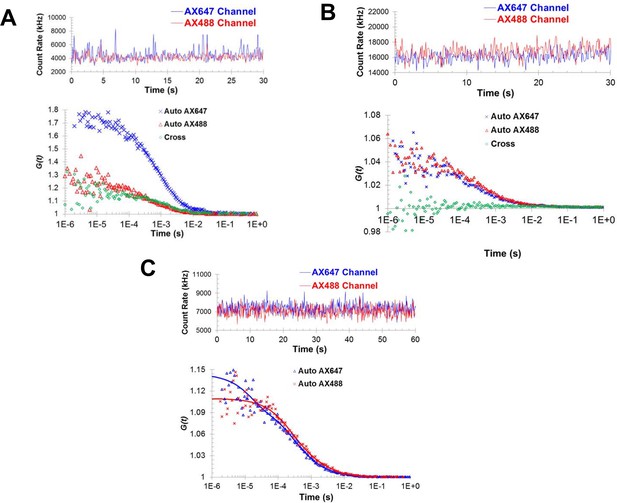
Fluorescence correlation spectroscopy confirms the formation of Syt1C2AB oligomers in solution.
Fluorescence cross-correlation spectroscopy of the Syt1C2AB oligomerization reaction was measured using Alexa488 and Alexa647 labeled Syt1C2AB, mixed with unlabeled Syt1C2AB protein either in the presence (A) or absence (B) of 1 mM ATP and Mg2+. The upper panels represent the count rates and the lower panels represent the auto-correlation (blue and red markers for Alexa647 and Alexa488) and cross correlation (green markers) functions. The data were collected for a range of fluorophores concentration (~30–120 nM) and we observe no cross-correlation even at higher label concentration in the absence of Mg2+/ATP (B) in contrast to distinct cross-correlation in the presence of Mg2+/ATP (A) despite the lower label concentration. This shows that these structures are not random association of Syt1C2AB molecules, but are ATP-derived oligomers of Syt1C2AB. (C) Fluorescence auto correlation spectroscopy of the oligomerization reaction was carried out using dilute concentration of the labeled Syt1C2AB (~25 nM each of Alexa488 and Alexa647) mixed with unlabeled Syt1C2AB in the presence of ATP and Mg2+ The auto correlation function was best fitted with the two-component translational diffusion model with triplet state (blue and red solid lines for each dye), confirming the presence of the oligomeric component. Representative traces are shown and the calculated parameters from a minimum of three independent trials are shown in Table 1.
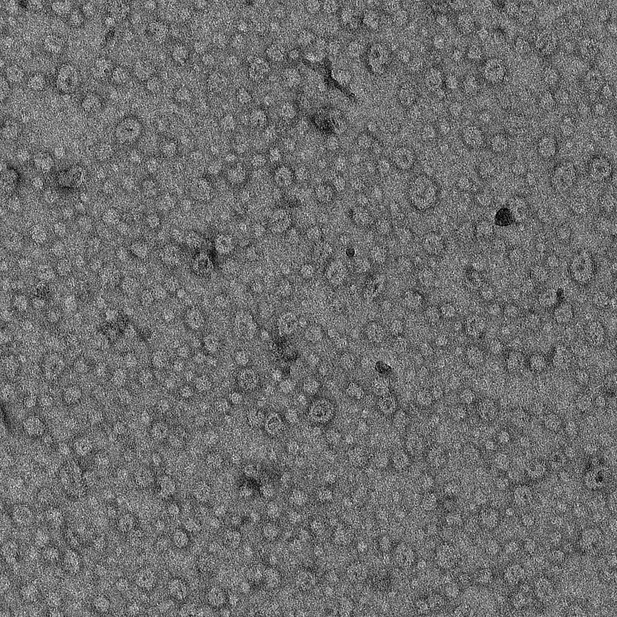
Negative stain EM analysis shows that the fluorescent label (Alexa488) introduced at position 269 does not affect Syt1C2AB ability to form ring oligomers on lipid monolayers containing 40% DOPS.
https://doi.org/10.7554/eLife.27441.006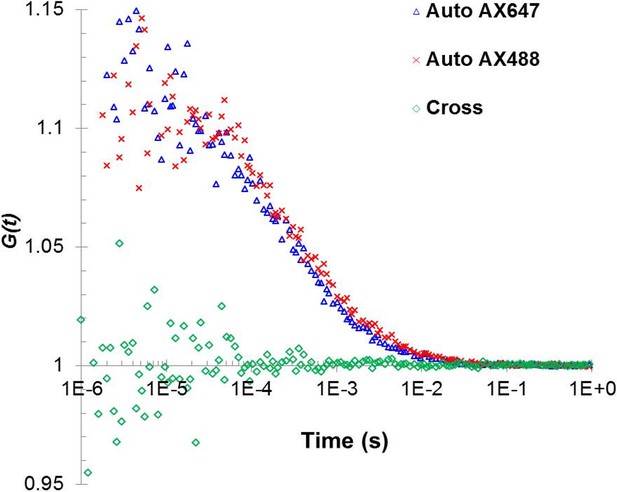
Fluorescent cross-correlation analysis confirms that under the low concentration of Alexa488 and Alexa647 used in the autocorrelation analysis, the two fluorophores are not located in the same oligomer and thus, could be used independently to cross-verify the analysis.
https://doi.org/10.7554/eLife.27441.007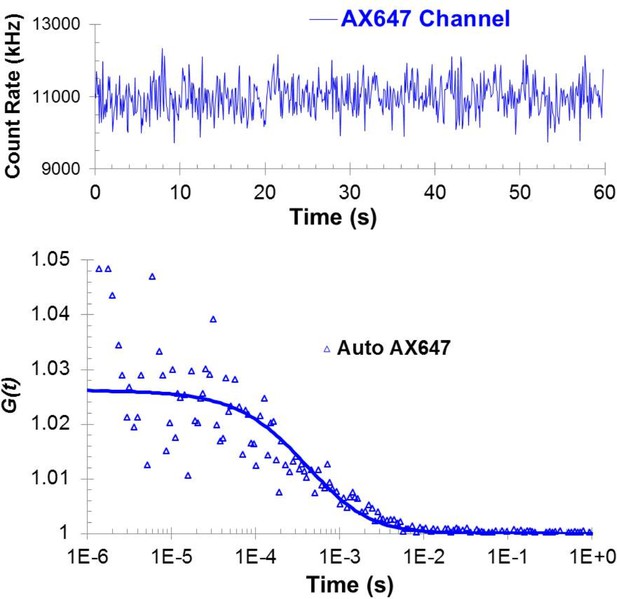
Autocorrelation analysis of the oligomerization reaction of C2B polylysine motif mutant was carried out using the mixture of wild type Syt1C2AB -Alexa647 and unlabeled Syt1 C2AB K326A/K327A.
The data show the absence of oligomers even in the presence of ATP/Mg2+ and can be best fitted using a single component model, with properties of the Syt1C2AB monomer.
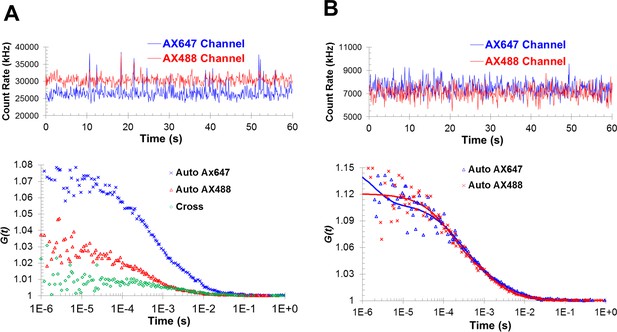
Fluorescence cross-correlation and autocorrelation analysis shows the formation of Syt1C2AB oligomers with PIP2-diC4 and Mg2+.
(A) The cross-correlation is larger than one at short time intervals, suggesting the co-migration of the two fluorophores. (B) The formation of oligomers is also reflected in the best fits of the autocorrelation functions by two-component diffusion model, similar to those observed with ATP/Mg2+.
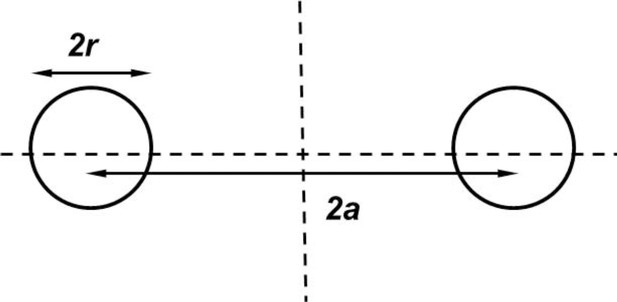
Geometry of the torus model (Thaokar, 2008) used to estimate the hydrodynamic radius of the Syt1C2AB oligomers.
The torus model was as best approximate considering the ring-like oligomeric structure observed in the EM analysis.
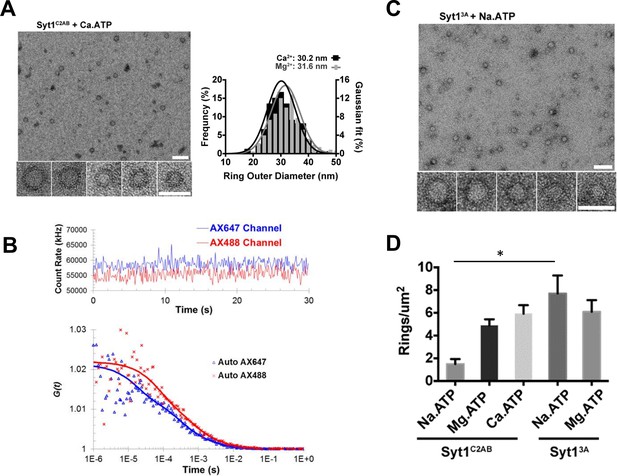
Soluble Syt1C2AB oligomers are insensitive to Ca2+.
(A) Negative stain analysis shows that the Syt1C2AB assembles into ring-like oligomers in the presence of Ca2+ and ATP and these ring oligomers are similar in size and frequency to the rings assembled with Mg2+/ATP. (B) Fluorescence autocorrelation analysis corroborates the insensitivity of the ring oligomers to Ca2+ as the auto correlation function of Syt1C2AB incubated with Ca2+ and ATP is best described with the two-component translational diffusion model with triplet state revealing the presence of the oligomeric component. (C) Neutralizing the Ca2+ coordinating aspartic acids in the C2B domain stabilizes the soluble ring oligomers even in absence of Mg2+ ions clarifying the molecular role of Mg2+ in the soluble Syt1C2AB ring assembly. The stability of the ring oligomers under various conditions was quantified by density of the rings on the EM grid (D). Average values and deviations from a minimum of 3 independent experiments are shown.
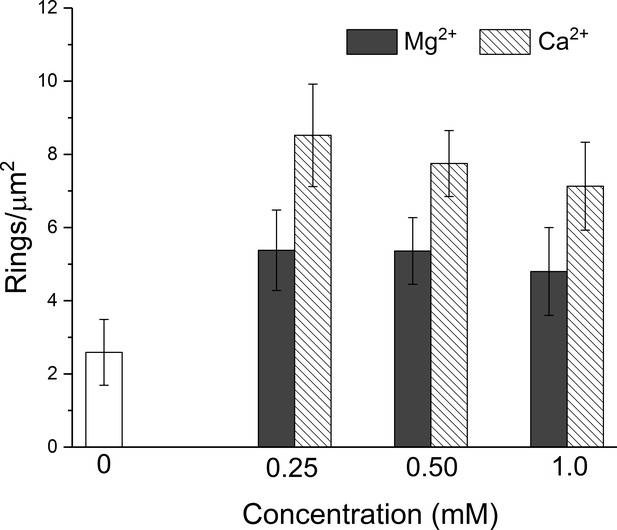
Negative stain EM analysis shows that both Mg2+ and Ca2+ act in a similar manner to stabilize the soluble Syt1C2AB ring-like oligomers.
Both divalent cations increase the density of the ring oligomers observed over a range of concentration (0.25–1 mM) tested, but Ca2+ is more efficient than Mg2+.
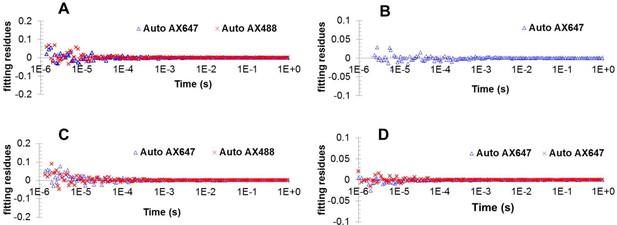
Fitting residues from fitting autocorrelation function for data shown in Figure 3C.
(A) Figure 3—figure supplement 2 (B); Figure 3—figure supplement 4B (C) and Figure 4B (D).
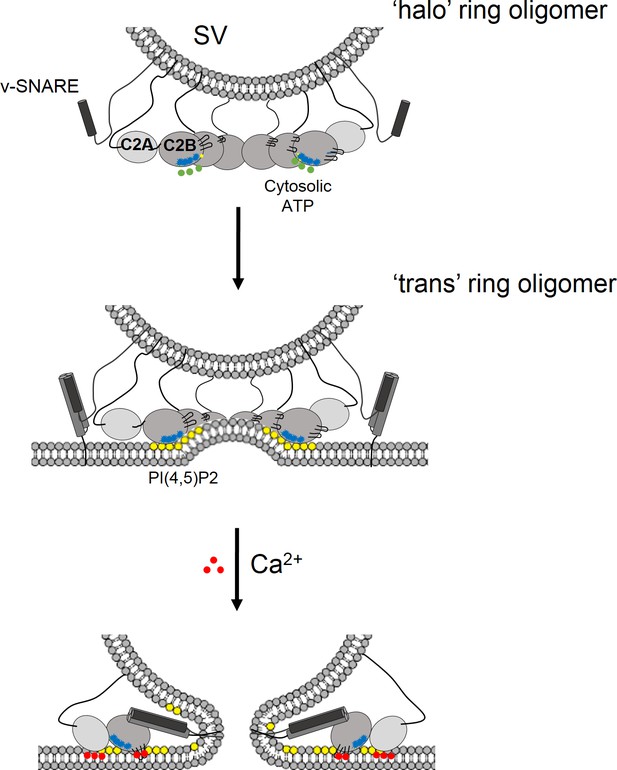
Model for the regulation of neurotransmitter release by the Syt1 ring oligomers.
The Syt1 ring oligomer formation may be triggered prior to vesicle docking that is halo-like structure by cytosolic ATP (green dots) binding to the Syt1 C2B polylysine motif (blue dots). The ring oligomers are then transferred to the site of docking wherein the ATP interaction is replaced with the PIP2 clusters (yellow dots) on the plasma membrane. This serves to enhance the docking ability of the Syt1 and position the ring at the docking site prior to the engagement of the SNARE proteins. The dimensions of the Syt1 ring, would permit the assembly of N-terminal domain of SNARE, but impede the complete zippering either passively as a spacer/washer or actively by restraining the SNARE assembly via specific interaction with t-SNAREs. Upon binding Ca2+ (red dots), the Ca2+ loops that is at the oligomeric interface, re-orients and inserts into the membrane. This disrupts the Syt1 ring oligomer and removing the impediment for fusion. In this fashion, the Syt1 ring oligomers could synchronize the release neurotransmitters to the influx of Ca2+ following the action potential.
Tables
Fractions and molecular properties of diffusional particles of Syt1C2AB generated various conditions as measured by fluorescence correlation spectroscopy.
Averages and standard errors from three independent trials are shown.
Proteins | Total protein conc. (nM) | Mg2+ + polyphosphate | Readout channel | Component 1 | Component 2 | ||||
---|---|---|---|---|---|---|---|---|---|
Fraction (%) | Diffusion time (µs) | Diffusion coefficient (μm2/s) | Fraction (%) | Diffusion time (µs) | Diffusion coefficient (μm2/s) | ||||
Syt1-Alexa488 | ~30 | No | Alexa488 | 100 | 620 ± 35 | 70 ± 4 | n/a | n/a | n/a |
Syt1-Alexa647 | ~30 | No | Alexa647 | 100 | 616 ± 22 | 70 ± 3 | n/a | n/a | n/a |
Syt1-Alexa488 + Syt1-Alexa647 + unlabeled Syt1 | ~5000 | No | Alexa488 | 100 | 583 ± 20 | 74 ± 8 | n/a | n/a | n/a |
Alexa647 | 100 | 577 ± 20 | 75 ± 8 | n/a | n/a | n/a | |||
Syt1-Alexa488 + Syt1-Alexa647 + unlabeled Syt1 | ~5000 | Yes | Alexa488 | 77 ± 2 | 527 ± 20 | 82 ± 4 | 23 ± 2 | 4168 ± 194 | 10 ± 1 |
Alexa647 | 76 ± 2 | 567 ± 26 | 77 ± 4 | 24 ± 2 | 3862 ± 196 | 11 ± 1 |
Additional files
-
Transparent reporting form
- https://doi.org/10.7554/eLife.27441.016