Global transcriptional analysis identifies a novel role for SOX4 in tumor-induced angiogenesis
Figures
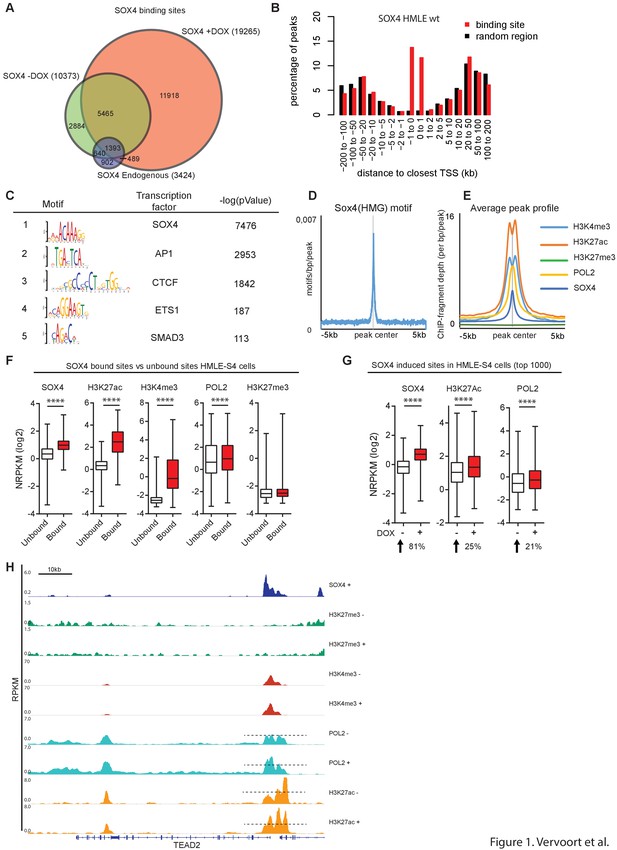
SOX4 is a transcriptional activator, preferentially binding to open/active chromatin.
(A) Venn-diagram showing the overlap of SOX4 binding sites identified by ChIP-seq in WT-HMLE and DOX-induced HMLE-S4 cells. (B). Genomic distribution of SOX4 binding sites in WT-HMLE cells with respect to annotated genes, represented as distance from the nearest TSS. Random genomic regions were used as background. (C). De novo motif analysis of SOX4-bound sites in DOX-treated HMLE-S4 cells. Selected significantly enriched motifs are represented. SOX4 was identified as the most highly enriched motif. (D) Density of the consensus SOX4 motif in SOX4 bound sites in HMLE-S4 cells 500 bp up- and downstream of the peak center. (E) Occupancy plots of SOX4, H3K27ac, H3K27me3, POL2 and H3K4me3 in the 10kb-genomic region surrounding the SOX4 peak center. (F) Changes in SOX4, H3K27ac, H3K4me3, POL2 and H3K27me3 are shown for SOX4-bound and SOX4-unbound sites (5%–95% whiskers, two-tailed Mann-Whitney U test, **** indicate p<0.0001). (G) Changes in SOX4, H3K27ac, and POL2 in HMLE-S4 for top 1000 DOX-induced sites ranked by SOX4-signal (****p<0.0001, Wilcoxon-signed-rank test) (H) Genomic tracks representing the occupancy of SOX4, H3K27me3, H3K4me3, H3K27ac and POL2 in untreated and DOX-treated HMLE-S4 cells surrounding the genomic locus of the canonical SOX4 target gene TEAD2.
-
Figure 1—source data 1
Analyses of Rapid Immunoprecipitation for Mass spectrometry on Endogenous proteins (RIME) to identify an optimaal anti-SOX4 antibody for ChIP described in Figure 1A.
- https://doi.org/10.7554/eLife.27706.004
-
Figure 1—source data 2
Overview of ChIPseq and RNAseq experiments described in Figures 1 and 2.
- https://doi.org/10.7554/eLife.27706.005
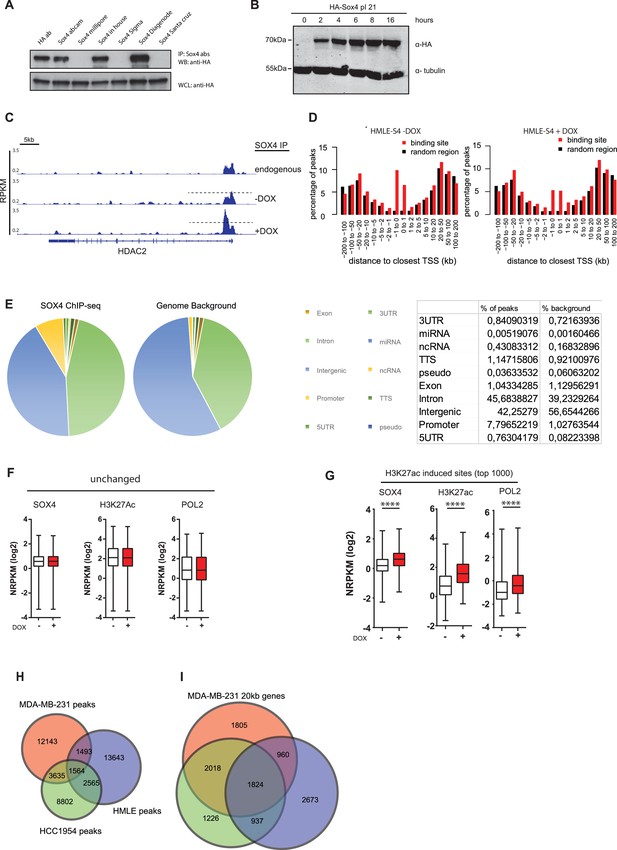
Antibody validation and ChIP-seq analysis.
(A) Commercially available and a custom-made SOX4 antibodies were tested for their ability to immunoprecipitate (IP) HA-tagged SOX4. HEK293T cells were transiently transfected with HA-tagged SOX4. Two days post transfection, cells were lysed in radio-immunoprecipitation buffer (RIPA) and IPs were formed using the indicated antibodies including an antibody against the HA-epitope. This demonstrated that the SOX4 diagenode antibody was most efficient at immunoprecipitating SOX4. (B) Validation of the successful lentiviral transduction and inducibility of the DOX-inducible pINDUCER-Sox4 construct in HMLE-cells. Transduced cells were treated with DOX (1 µg/ml) for the indicated time points after which induction of SOX4 expression was analyzed using an anti-HA antibody. (C) A representative example of SOX4 occupancy profiles derived by ChIP-seq normalized for sequencing depth (reads per kilobase per million reads sequenced; RPKM) in wt-HMLE and non-induced and induced HMLE-S4 cells. The genomic regions surrounding the HDAC2 locus is shown. (D) Histogram representing the distance of SOX4 peaks relative to the TSSs of annotated genes. (E) Genomic distribution of SOX4 peaks in DOX-treated HMLE-S4 cells over annotated regions compared to the genomic background. (F–G) Quantitative analysis of enhancer regions as defined by H3K27ac peaks. Normalized read density used to determine the sites without or with DOX-induced changes in SOX4, H3K27ac, H3K4me3, POL2 and H3K27me3 occupancy. Data are shown as boxplots with 5–95% whiskers. Statistical comparison was performed with a two-tailed Wilcoxon-signed-rank test (****p<0.0001) (H) Venn-diagram representing the overlap of SOX4 binding sites in MDA-MB-231, HCC1954 and DOX-treated HMLE-S4 cells. (I) Venn-diagram showing the overlap between genes with a SOX4 peak within 20 kb of their TSS in MDA-MB-231, HCC1954 and DOX-treated HMLE-S4 cells.
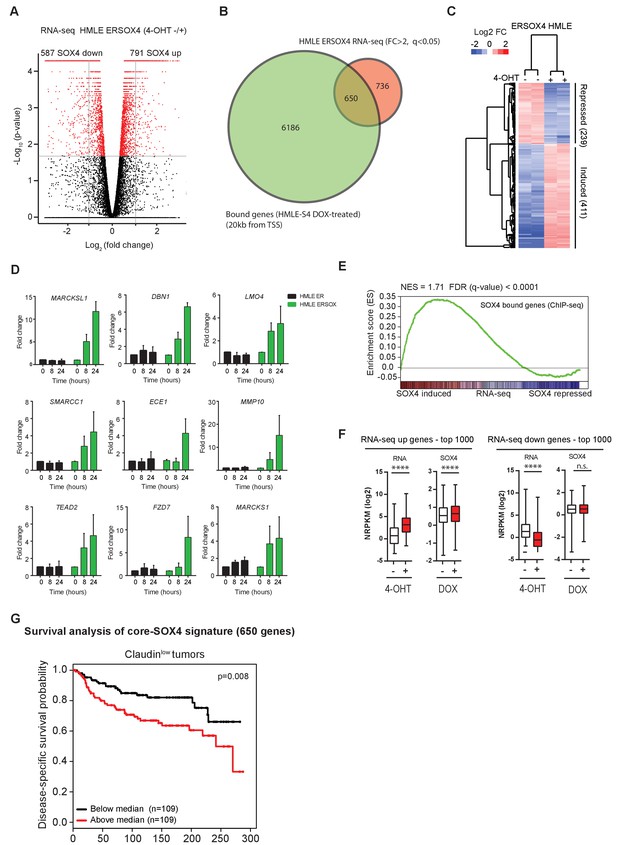
Identification of a novel core-SOX4 target gene signature with prognostic value and pro-angiogenic networks.
(A) Gene expression analysis of SOX4 induced changes in HMLE ERSOX4 cells conditionally activated by 4-OHT for 8 hr and analyzed by RNA-seq. No significantly changed genes were observed in ER-control HMLE cells (data not shown). Significantly changed genes (q-value < 0.05) are indicated in red. (B) Venn diagram showing the overlap of genes regulated by SOX4 on the RNA-level (FC > 2, q-value < 0.05) with genes possessing a SOX4 binding site within 20 kb from an annotated TSS in DOX-treated HMLE-S4 cells (650 genes). (C). Heatmap visualizing gene expression changes for core-SOX4 target genes. (D) Quantitative real-time PCR analysis of gene expression changes in 4-OHT treated ER-control and ERSOX4 HMLE cells for selected SOX4 target genes identified by RNA-seq. Data represented as mean ± SD, normalized for β2M of three independent biological replicates. (E) Gene-set enrichment analysis (GSEA) representing the enrichment of SOX4 bound sites (2 kb from TSS) in the RNA-seq expression dataset ranked by log2 fold change upon conditional activation of SOX4. (F) Quantitative analysis of changes in SOX4 occupancy in peaks associated with most highly induced or repressed genes derived from the RNA-seq dataset generated in ERSOX4 HMLE cells (****p<0.0001, Wilcoxon-signed-rank test). (G) Analysis of the prognostic value of the core-SOX4 gene expression signature (650 genes) in claudinlow breast cancer patients (METABRIC).
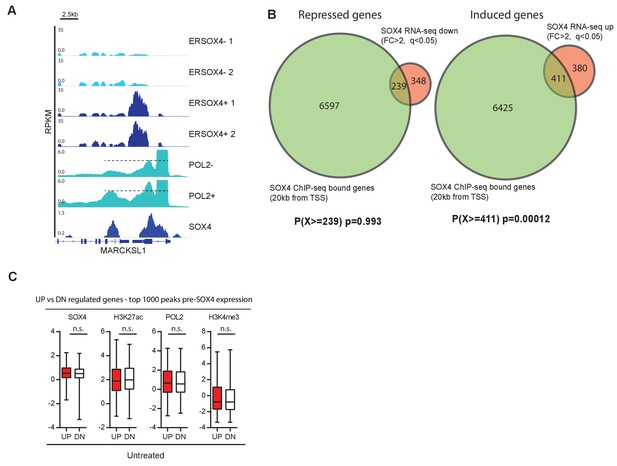
Overlap of ChIP-seq and RNA-seq datasets.
(A) Representative image of normalized RNA-seq profiles for the genomic region surrounding the MARCKSL1 gene. SOX4 binding and POL2 profiles in DOX-treated and -untreated HMLE-S4 cells for the same locus are also represented (-/+). (B) Venn-diagrams representing the overlap between SOX4- repressed (left) or SOX4-induced (right) genes with genes containing a SOX4-binding site within 20 kb of their TSS in DOX-treated HMLE-S4 cells. Hypergeometric tests were performed to test the enrichment in the gene overlap relative to chance (p-values indicate the random chance of finding an equal or greater overlap between the two datasets). (C) Quantitative analysis of top 1000 regions of open chromatin defined by H3K27ac peaks associated with the most highly induced (UP) or repressed genes (DN) (top 1000 peaks analyzed). Normalized read density was determined and the comparison of SOX4, H3K27ac, H3K4me3, and POL2 occupancy between UP and DN-associated peaks is represented by boxplots with 5–95% whiskers. Statistical comparison was performed with a two-tailed Mann-Whitney U test (****p<0.0001).
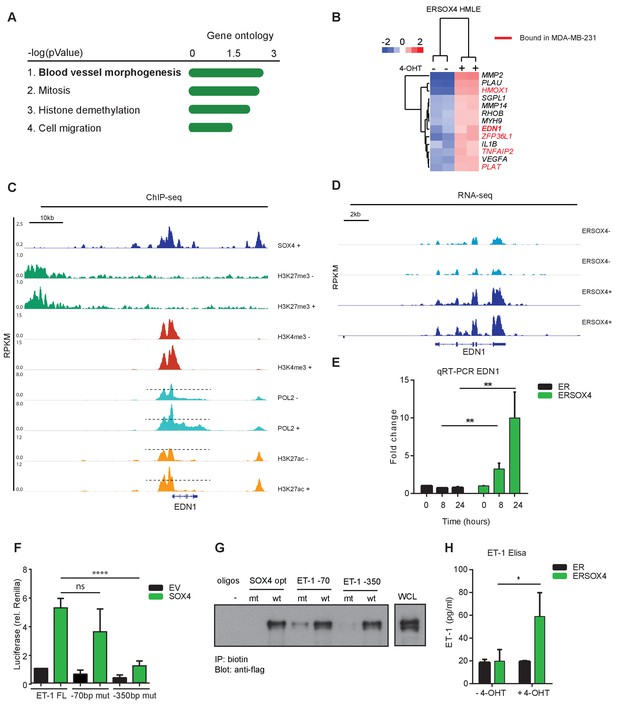
SOX4 directly regulates EDN1 mRNA expression and promotes production of the mature ET-1 peptide.
(A) Gene Ontology (GO) analysis on core-SOX4 target genes. Top terms are shown. (B) Heatmap of gene expression changes upon conditional SOX4 activation in HMLE cells for genes associated with the GO-term Blood vessel morphogenesis (HMLE regulated and bound). Genes with a SOX4-binding site within 20 kb of a TSS in MDA-MB-231 cells are annotated in red (C) Normalized ChIP-seq tracks for SOX4, H3K27me3, H3K4me3, POL2, and H3K27ac surrounding the EDN1 locus in HMLE-S4 cells. For histone marks and POL2 both DOX-treated and -untreated profiles are represented (-/+). (D) Normalized RNA-seq profile for the EDN1 gene in untreated and 4-OHT-treated ERSOX4 HMLE cells (-/+). (E) qRT-PCR analysis of EDN1 expression in 4-OHT-treated ER and ERSOX4 HMLE cells. Data represented as mean ± SD, normalized to β2M of three independent biological replicates (**p-value<0.01, Student’s t-test). (F) Luciferase assay in HEK293T cells transiently transfected with the full-length EDN-1 promoter (FL) as well as luciferase constructs in which the conserved sites have been mutated (−70 and −350). Results obtained from three independent biological replicates wherein three independent technical replicates were used per condition (ns: non-significant; ****p-value<0.001, Student’s t-test). (G) Pulldown assay in Flag-Sox4 transfected HEK293T cells using biotinylated DNA-probes. Probes matching an optimal SOX4-binding sequence, and the −70 bp and −350 bp of the EDN1 promotor sequence are indicated and mutated versions thereof (- indicates empty beads). Representative data obtained from three independent biological replicates. (H) Enzyme-linked immunosorbent assay (ELISA) analysis of ET-1 expression in the overnight conditioned media of 4-OHT treated and untreated HMLE-S4 cells. Results obtained from three independent biological replicates (*p-value<0.05, Student’s t-test).
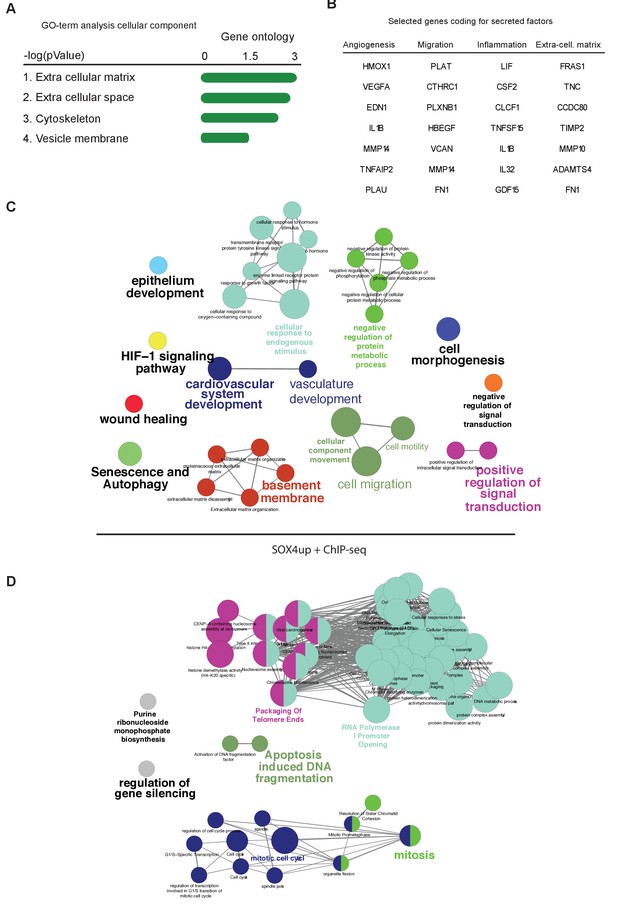
Analysis of patient survival and cellular processes associated with the core-SOX4 target gene signature.
(A) GO-term analysis for core-SOX4 target genes based on location in cell for gene-product (B) Selected genes coding for secreted factors (C–D) GO-term analysis performed in cytoscape using ClueGO of the 411 positively regulated and 239 negatively regulated genes. Visualized GO-terms are significantly enriched (p<0.05).
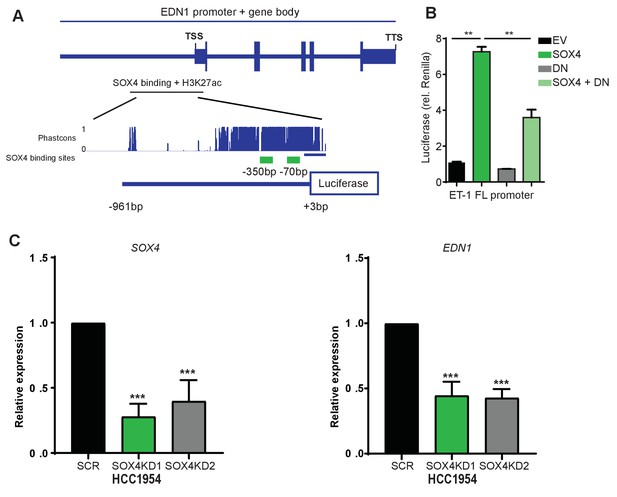
SOX4 activates the EDN1-luciferase promoter and SOX4 expression correlates with EDN1 in breast cancer patients.
(A) Schematic representation of the EDN1 promoter. The magnification shows the SOX4 and H3K27ac bound locus. Represented are the phastcon score for mammalian conservation in 46 vertebrates (upper track), with conserved SOX4-binding sites indicated underneath in green (−350 bp and −70 bp from TSS). Represented below is a schematic image of the EDN1-luciferase promoter used in this study. (B) Luciferase assay in HEK293T cells transiently transfected with the EDN1-luciferase promoter, renilla-luciferase in combinantion with either empty vector pcDNA3 (EV), flag-SOX4-pcDNA3 (SOX4), dominant negative SOX4 (DN, aa1-135), or SOX4 + DN. Data represented as mean ± S.E.M. normalized to renilla relative to EV (three independent biological replicates, statistical significance determined by ANOVA) (C) qRT-PCR quantification of SOX4 and EDN1 expression in SCR control and SOX4 KD HCC1954 cells. Data represented as mean ± SD of three independent biological replicates (***p-value<0.001, Student’s t-test).
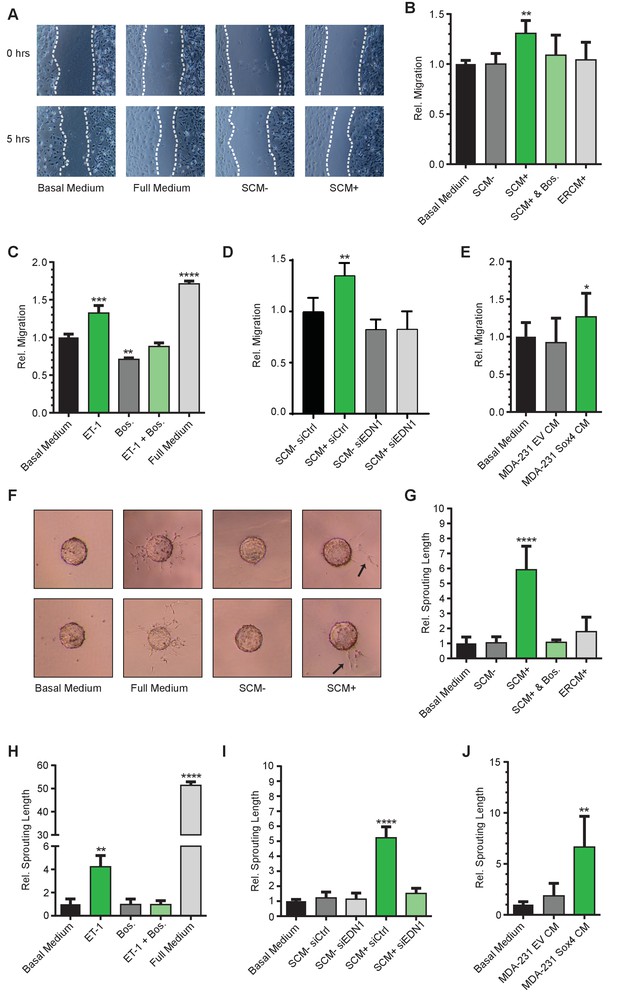
SOX4 mediated induction of ET-1 expression promotes tumor-induced angiogenesis in vitro.
(A) Representative images of wound-healing assays performed on HMEC-1 cells, treated for 5 hr with basal media, full growth media or conditioned media from HMLE-S4 cells with or without overnight treatment with 4-OHT (SCM + and SCM-, respectively). Data from three independent biological replicates (B) Quantification of wound closure relative to basal media for HMEC-1 cells treated with conditioned media; SCM-, SCM+, SCM+ with Bosentan, and conditioned media from ER-control HMLE cells treated with 4-OHT (ERCM+). Data represented as mean ± SD of three independent biological replicates. (C) Quantification of wound closure in HMEC-1 cells treated with a synthetic ET-1 peptide, the ET-1 receptor antagonist Bosentan (Bos), ET-1 + Bos and full growth media represented as relative migration compared to basal media. Data represented as mean ± SD of four independent biological replicates. (D) Quantification of the wound healing assay in presence of SCM-/+generated from siEDN1 or control siRNA treated HMLE cells. Results obtained from three independent biological replicates. (E) Quantification of the wound-healing assay in the presence of conditioned media from MDA-MB-231 cells stably expressing either an empty vector control (EV) or SOX4 construct. Results represented are relative to basal media. Data represented as mean ± SD of three independent biological replicates. (F) Representative images of sprouting assays performed with HMEC-1 cells in the presence of indicated media conditions. (G–J) Quantification of sprouting length in the indicated conditions relative to basal media. Data represented as mean ± SD of four independent biological replicates.
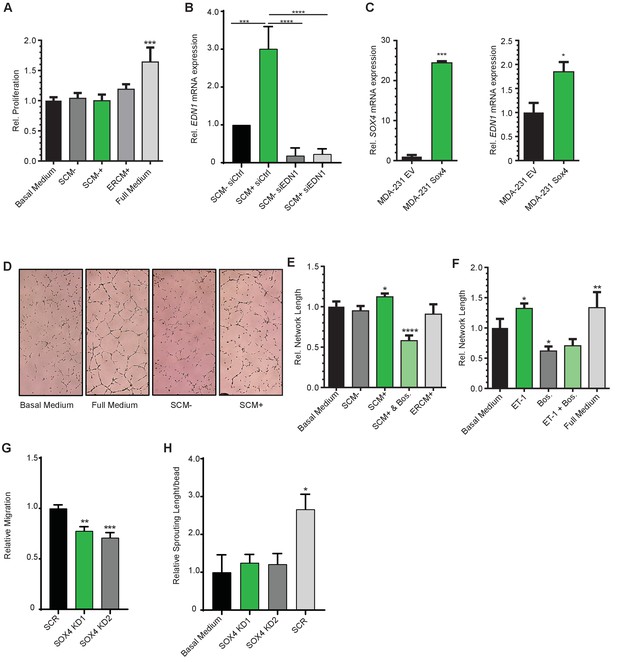
Conditioned media from conditionally activated ERSOX4 HMLE cells does not affect HMEC-1 cell proliferation.
(A) HMEC-1 cells were plated and incubated for 48 hr in the presence of basal media or indicated conditioned media, as well as full growth media. Subsequently, cells were counted and the change in numbers was represented relative to the basal control media. Data are represented as mean ± SD. (three independent biological replicates) (B) qRT-PCR quantification of EDN1 expression in HMLEs either treated with a Scrambled Control siRNA (siControl) or with a siRNA targeted against EDN1 (siEDN1). After siRNA treatment and conditional SOX4 activation cells were lysed for qRT-PCR analysis shown here, while conditioned medium was added to HMEC-1 endothelial cells. Data represented as mean ± SD of three independent biological replicates. (C) qPCR validation of SOX4 overexpression in MDA-MB-231 cells and EDN1 expression (D) Representative images of network formation assays in HMEC-1 cells plated on top of matrigel in the presence of indicated media. (E–F) Quantification of relative network length for the indicated conditions relative to basal media. Data is represented as mean ± SD (three independent biological replicates). (G) Quantification of relative migration of HMEC-1 endothelial cells in the presence of conditioned medium derived from MDA-MB-231 cells transduced with scrambled, SOX4 KD1, SOX4 KD2 shRNA. Data represented as mean ± SD of three independent biological replicates. (H) Quantification of sprouting length of HMEC-1 endothelial cells in the presence of basal medium or conditioned medium derived from MDA-MB-231 cells transduced with scrambled, SOX4 KD1, SOX4 KD2 shRNA. Data represented as mean ± SD of three independent biological replicates.
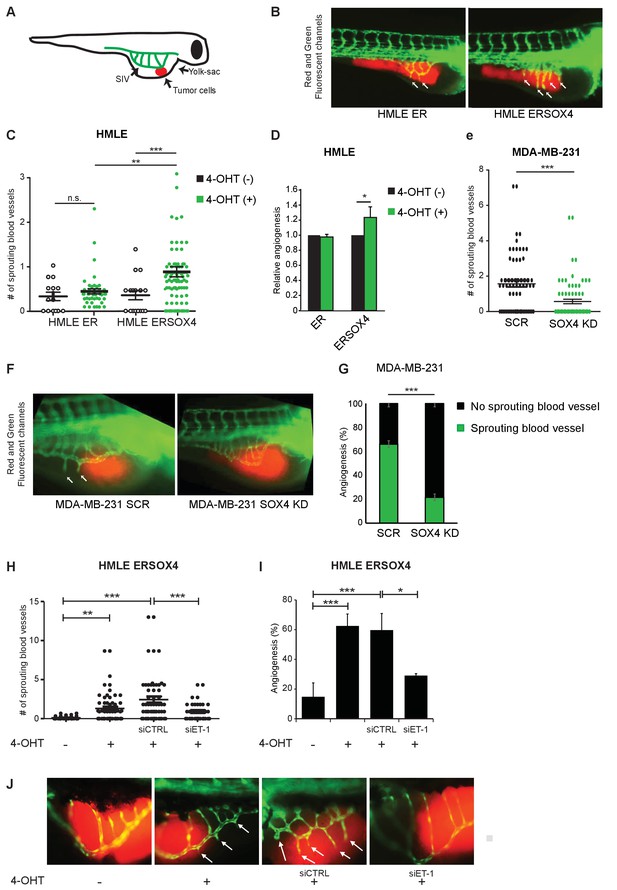
SOX4 controls tumor-induced angiogenesis in vivo in a zebrafish tumor-xenograft model.
(A) Schematic representation of the zebrafish tumor-xenograft model, with the subintestinal vessels indicated in green (SIV) and tumor cells in red. (B) Representative images of zebrafish injected with ER-control and ERSOX4 HMLE cells treated with 4-OHT. Arrows indicate newly formed blood vessels. (C) Quantification of the number of ectopic sprouts observed per fish injected with ER-control and ERSOX4 HMLE cells. Individual data points and data represented as mean ± SD of three independent biological replicates (**p-value<0.01, ***p-value<0.001; ANOVA). (D) Quantification of the relative increase in ectopic sprouting in fish injected with 4-OHT treated relative to untreated ER-control and ERSOX4 cells. Data represented as mean ± SD of three independent biological replicates (* p-value<0.05, Student’s t-test). (E) Quantification of the number of ectopic sprouts in fish injected with SCR control and SOX4 KD MDA-MB-231 cells. Results obtained from three independent biological replicates (***p-value<0.001, Student’s t-test). (F) Representative images of zebrafish injected with SCR control or SOX4 KD MDA-MB-231 cells. Arrows indicate newly formed blood vessels (G) Quantification of the number of fish injected with MDA-MB-231 cells with or without ectopic sprouting. Results obtained from three independent biological replicates (***p-value<0.001, Student’s t-test). (H) Quantification of the number of ectopic sprouts observed per fish injected with 4-OHT-treated ERSOX4 HMLE cells exposed with siRNA control or siRNA targeting ET-1. Individual data points and data represented as mean ± SD (*p-value<0.05, ANOVA). (I) Quantification of the relative increase in ectopic sprouting in fish injected with 4-OHT treated ERSOX4 HMLE cells exposed with siRNA control or siRNA targeting ET-1. Data represented as mean ± SD of three independent biological replicates (*p-value<0.05, ***p-value<0.001; ANOVA). (J) Representative images of zebrafish injected with 4-OHT-treated ERSOX4 HMLE cells exposed with siRNA control or siRNA targeting ET-1. Arrows indicate newly formed blood vessels.
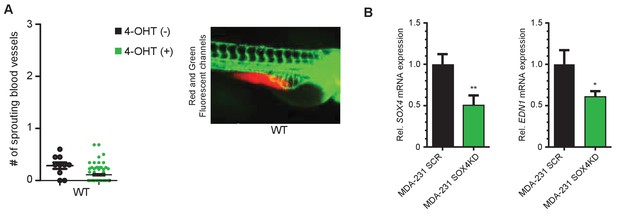
Analysis of angiogenic potential of HMLE WT cells.
SOX4 and EDN1 mRNA expression in SOX4-depleted MDA-MB-231 cells. (A) Quantification of ectopic sprouting in zebrafish tumor-xenograft model using wild-type (WT) HMLE cells in presence of absence of 4-OHT. (B) qPCR validation of SOX4 knockdown (KD) relative to SCR control in MDA-MB-231 cells with matching EDN1 expression.
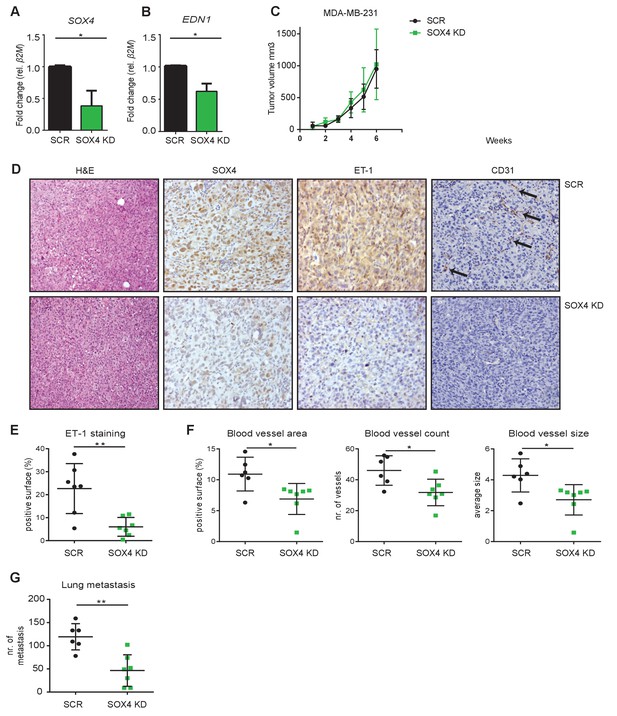
Depletion of SOX4 in MDA-MB-231 cells reduces ET-1 expression and decreases tumor vascularization and metastasis in a xenograft mouse model of breast cancer.
(A–B). qRT-PCR quantification of SOX4 and EDN1 expression in luciferase-marked SCR control and SOX4 KD MDA-MB-231 cells. Data represented as mean ± SD of three independent biological replicates (*p-value<0.05, Student’s t-test). (C) Growth curve of SOX4 KD and SCR control MDA-MB-231 tumors. Data represented as mean ± SD of three independent biological replicates (D) IHC analysis of scrambled control and SOX4 KD MDA-MB-231 primary tumors. Representative Hematoxylin and eosin stainings (H and E) and IHC stainings for SOX4, ET-1 and CD31 (PECAM-1) are shown. Arrows indicate blood vessels. (E) Quantification of ET-1 staining. Indicated is the total surface passing the filter threshold. Data represented as mean ± SD (**p-value<0.01, student t-test). (F) Quantitative analysis of the endothelial cell marker CD31 in SOX4 KD and control tumors. Images were analyzed by ImageJ software and total vessel area, blood vessel count and blood vessel size are indicated (*p-value<0.05, t-test). (G) Quantitative analysis of the number of visible lung metastases in SOX4 KD and SCR control MDA-MB-231 tumors. Data represented as mean ± SD (**p-value<0.01, t-test).
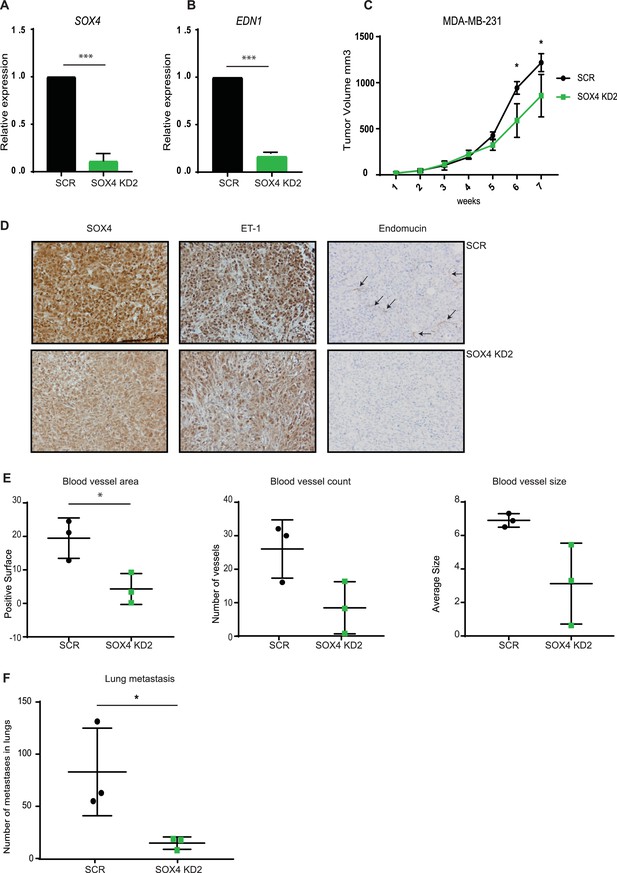
Depletion of SOX4 using a second ShRNA in MDA-MB-231 cells reduces Endothelin-1 expression and decreases tumor vascularization and metastasis in a xenograft mouse model of breast cancer.
(A–B). qRT-PCR quantification of SOX4 and EDN1 expression in luciferase-marked SCR control and SOX4 KD2 MDA-MB-231 cells. Data represented as mean ± SD of three independent biological replicates (*p-value<0.05, Student’s t-test). (C) Growth curve of SOX4 KD2 and SCR control MDA-MB-231 tumors. Data represented as mean ± SD of three independent biological replicates (D) IHC analysis of scrambled control and SOX4 KD MDA-MB-231 primary tumors. Representative IHC stainings for SOX4, ET-1 and Endomucin are shown. Arrows indicate blood vessels. (E) Quantitative analysis of the endothelial cell marker Endomucin in SOX4 KD2 and control tumors. Images were analyzed by ImageJ software and total vessel area, blood vessel count and blood vessel size are indicated (*p-value<0.05, t-test). (F) Quantitative analysis of the number of visible lung metastases in SOX4 KD2 and SCR control MDA-MB-231 tumors. Data represented as mean ± SD (*p-value<0.05, t-test).
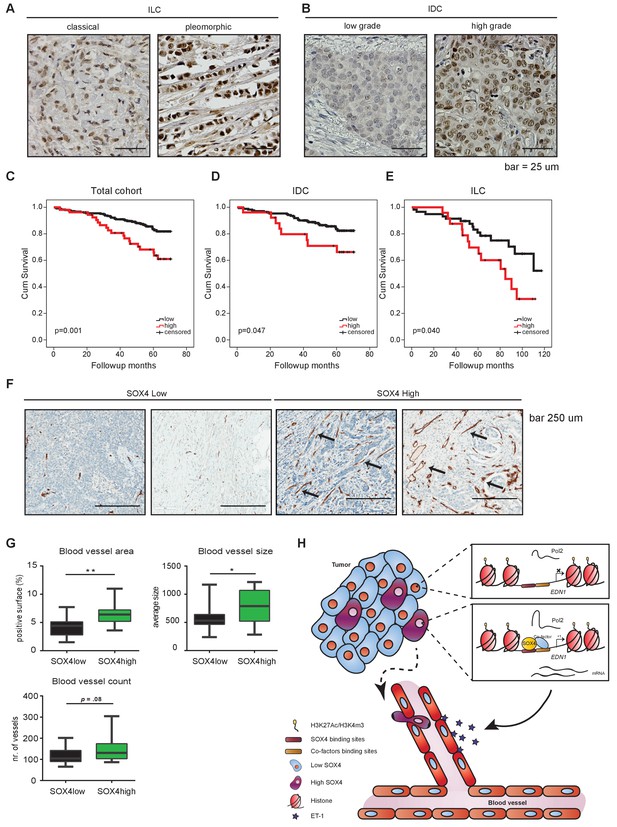
Immunohistochemical analysis of SOX4 expression in patients with human breast cancer reveals a positive correlation with decreased survival, increased metastasis and elevated blood vessel density.
(A–B) Representative images of SOX4 expression in invasive lobular carcinoma (ILC, classical and pleomorphic) and invasive ductal carcinoma (IDC, low-grade tumor and high-grade tumor) cases in the tissue microarray (TMA) cohort. (C–E) Kaplan-Meier curves for cumulative (cum) survival for patients with high or low nuclear SOX4 expression for the total cohort, ILC and IDC breast cancers. Indicated p-values were calculated using the Log-rank test. (F) Representative images of CD34 staining in SOX4HI and SOX4LO tumors as identified previously in TMA analysis. Arrows indicate blood vessels. (G) Quantitative analysis of CD34 staining in 17 SOX4HI and 17 SOX4LO ductal carcinomas. (Student t-test, *p-value<0.05). (H) Schematic model for the pro-angiogenic function of SOX4 during breast cancer progression. The SOX4 transcription factor binds to regions of active/open chromatin and subsequently acts as positive regulator of many genes involved in tumorigenesis, such as EDN1. Upregulation of ET-1 contributes to the increase of neovascularization, which ultimately may facilitate tumor cell intravasation and growth factor accessibility.
-
Figure 7—source data 1
Correlation of nuclear SOX4 expression with clinicopathological and molecular features of invasive breast cancer.
- https://doi.org/10.7554/eLife.27706.020
-
Figure 7—source data 2
Correlation of nuclear SOX4 expression with clinicopathological features of IDC and ILC.
- https://doi.org/10.7554/eLife.27706.021
-
Figure 7—source data 3
Clinicopathological characteristics of 452 breast cancer patients studied for the expression of SOX4.
- https://doi.org/10.7554/eLife.27706.022
-
Figure 7—source data 4
Clinicopathological characteristics of 27 patients with metastatic breast cancer studied for the expression of SOX4.
- https://doi.org/10.7554/eLife.27706.023
-
Figure 7—source data 5
Correlation of nuclear SOX4 expression with metastasis formation.
- https://doi.org/10.7554/eLife.27706.024
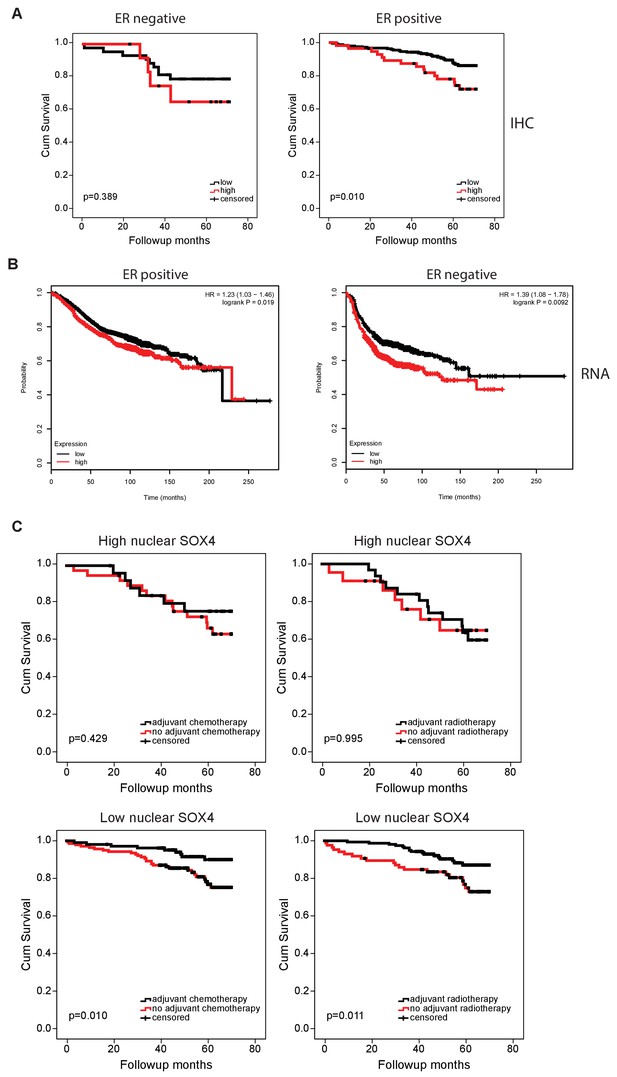
SOX4 mRNA expression correlates with decreased patient survival in both ER positive and negative breast cancers.
(A) Analysis of patient survival according to ER-status and stratified into SOX4HIand SOX4LO tumors (B) Correlation between SOX4 mRNA expression and patient survival was analyzed using the KMPlot.com platform. The best probe-set was selected and the optimal cut-off was automatically determined. Tumors were separated into ER negative and ER positive prior to analysis. (C) Analysis of SOX4HIand SOX4LO tumors. Patient survival data stratified according the adjuvant radio- and chemotherapy treatments status.
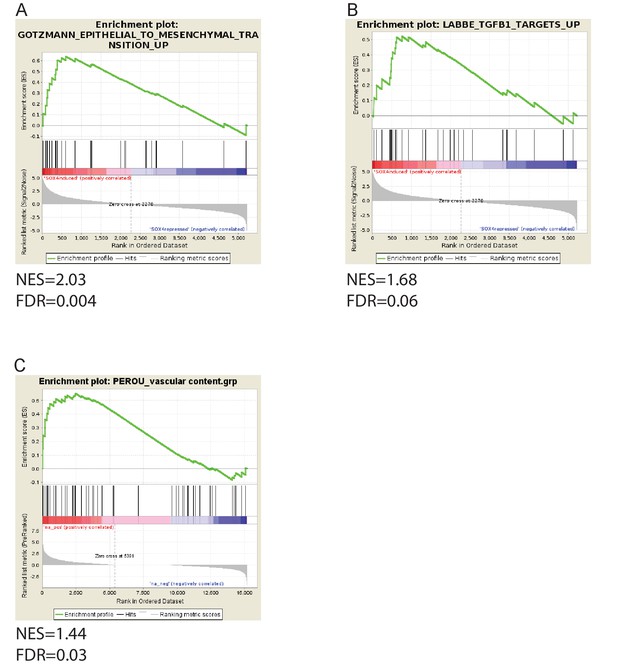
Gene set enrichment analysis on RNA-seq data derived from conditional activation of SOX4 in HMLE cells.
(A) Enrichment analysis of TGF-β signature (B) Enrichment analysis of EMT signature (C) Enrichment analysis of vascular content signature.
Additional files
-
Transparent reporting form
- https://doi.org/10.7554/eLife.27706.027