Sculpting ion channel functional expression with engineered ubiquitin ligases
Figures
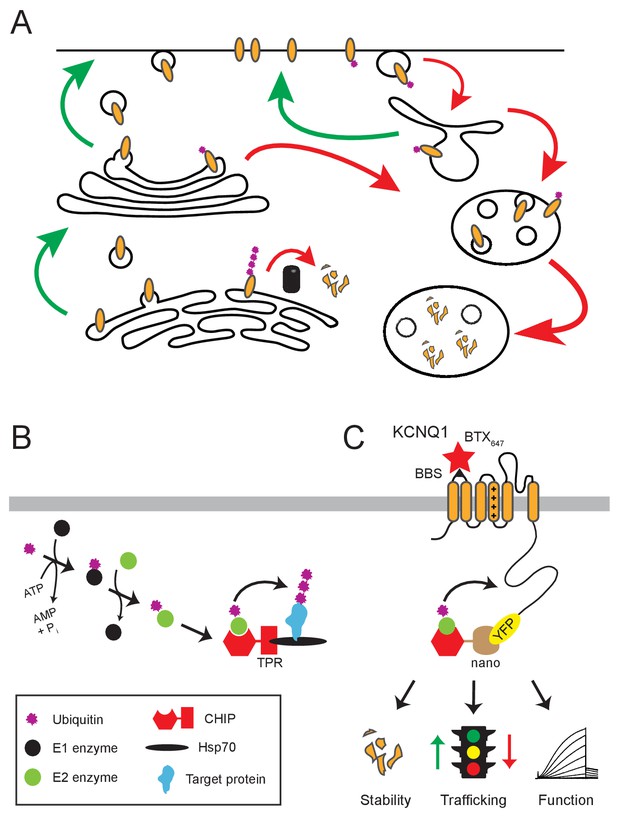
Role of ubiquitin in the lifecycle of membrane proteins.
(A) Dynamic trafficking of membrane proteins among subcellular compartments. Degradation can take place from the ER via the proteasome, or through downstream endocytic compartments via the lysosome. Ubiquitin (purple) is a molecular signal important for mediating multiple steps in membrane protein trafficking, function, and degradation. Forward trafficking (green) and reverse trafficking (red) processes are represented. (B) Enzymatic cascade of ubiquitination, including the ATP-dependent activation of ubiquitin (E1), ubiquitin conjugation (E2), and ultimate ubiquitin transfer to target substrate (E3). CHIP is an E3 ligase, recognizing Hsp70-bound substrates via the TPR binding domain and catalyzing their ubiquitination via the U-box domain (hexagon). (C) Schematic for engineering an E3 ubiquitin ligase and potential outcomes on an ion channel substrate. The substrate-binding TPR domain of CHIP is replaced with GFP-binder, vhh4 nanobody, creating nanoCHIP which has novel selectivity towards YFP-tagged Q1 subunits. The bungarotoxin binding site (BBS) epitope (S1–S2) allows for selective labeling of surface Q1 subunits, YFP signal represents total Q1 expression. This experimental paradigm enables robust analysis of Q1 stability, trafficking, and function.
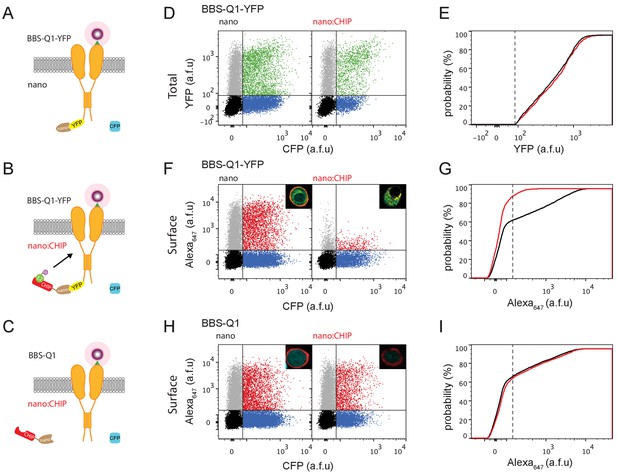
nanoCHIP selectively abolishes Q1 surface expression.
(A–C) Cartoons of experimental strategies. BBS-Q1-YFP was co-transfected with either nanobody alone (A) or with nanoCHIP (B). Untagged BBS-Q1 co-expressed with nanoCHIP was used as a control to test for specificity of the approach (C). (D) Flow cytometry analyses of total Q1 expression (YFP fluorescence) in cells expressing BBS-Q1-YFP + nanobody (left, control) or BBS-Q1-YFP + nanoCHIP (right).~50,000 cells are represented in dot plots here and throughout. Vertical and horizontal lines represent thresholds for CFP and YFP-positive cells, respectively, based on analyses of single color controls. Represented are CFP-positive cells with YFP signal above (green dots) or below threshold (blue dots); YFP-positive cells with CFP signal below threshold (gray dots); and untransfected cells (black dots). (E) Cumulative distribution histograms of YFP fluorescence for BBS-Q1-YFP co-expressed with either nanobody (black line) or nanoCHIP (red line). Plot generated from population of YFP- and CFP-positive cells. Dotted line is threshold value for YFP signal. (F) Flow cytometry analyses of surface Q1 channels (Alexa647 fluorescence) in cells expressing BBS-Q1-YFP + nanobody (left, control) or BBS-Q1-YFP + nanoCHIP (right). Representative confocal images are inset. (G) Cumulative distribution histograms of Alexa647 fluorescence for BBS-Q1-YFP co-expressed with either nanobody (black line) or nanoCHIP (red line). Plot generated from population of CFP-positive cells. Dotted line is threshold value for Alexa647 signal. (H,I) Flow cytometry analyses of surface Q1 channels in cells expressing BBS-Q1 with either nanobody alone or with nanoCHIP. Same format as (F,G).
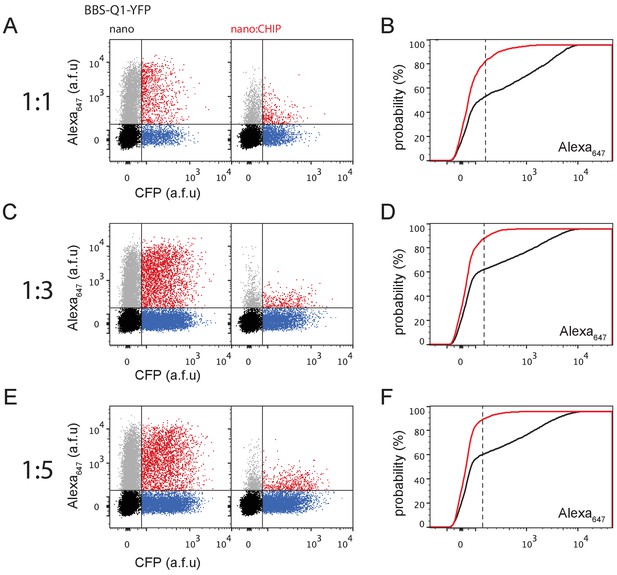
Titration of nanoCHIP expression.
(A,C,E) Flow cytometry analyses of surface Q1 channels (Alexa647 fluorescence) in cells expressing BBS-Q1-YFP + nanobody (left, control) or BBS-Q1-YFP + nanoCHIP (right), using 1:1 (A), 1:3 (C), and 1:5 (E) transfection ratios of BBS-Q1-YFP:nanoCHIP plasmids. (B,D,F) Cumulative distribution histograms of Alexa647 fluorescence for BBS-Q1-YFP co-expressed with either nanobody (black line) or nanoCHIP (red line).
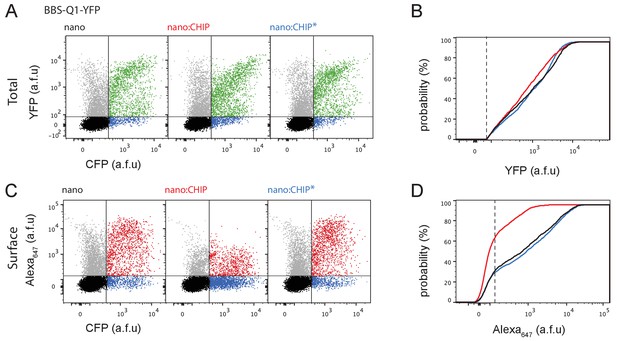
Catalytically inactive nanoCHIP* has no effect on Q1 surface expression.
(A) Flow cytometry analyses of total Q1 expression (YFP fluorescence) in cells expressing BBS-Q1-YFP + nanobody (left, control), BBS-Q1-YFP + nanoCHIP (center), and BBS-Q1-YFP + nanoCHIP* [Δ128–229] (right). (B) Cumulative distribution histograms of YFP fluorescence for BBS-Q1-YFP co-expressed with either nanobody alone (black line), nanoCHIP (red line), and nanoCHIP* [Δ128–229] (blue line). (C) Flow cytometry analyses of surface Q1 channels (Alexa647 fluorescence) in cells expressing BBS-Q1-YFP + nanobody (left, control), BBS-Q1-YFP + nanoCHIP (center), and BBS-Q1-YFP + nanoCHIP* [Δ128–229] (right). (D) Cumulative distribution histograms of Alexa647 fluorescence for BBS-Q1-YFP co-expressed with either nanobody alone (black line), nanoCHIP (red line), and nanoCHIP* [Δ128–229] (blue line).
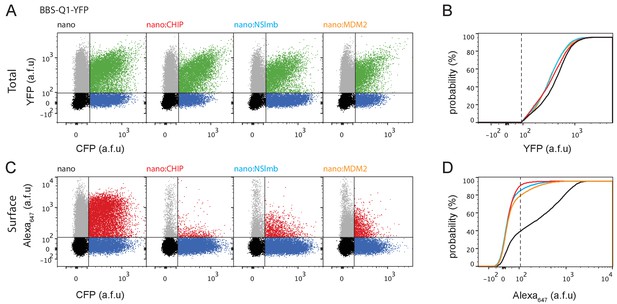
Screening the effects of different engineered E3 ligases.
(A) Flow cytometry analyses of total Q1 expression (YFP fluorescence) in cells expressing BBS-Q1-YFP + nanobody (left, control) or BBS-Q1-YFP + nanoCHIP, nanoNSlmb (termed deGradFP), or nanoMDM2. (B) Cumulative distribution histograms of YFP fluorescence for BBS-Q1-YFP co-expressed with either nanobody (black line) or nanoCHIP (red line), nanoNSlmb (blue line), or nanoMDM2 (orange line). (C) Flow cytometry analyses of surface Q1 channels (Alexa647 fluorescence) in cells expressing BBS-Q1-YFP + nanobody (left, control) or BBS-Q1-YFP + nanoCHIP, nanoNSlmb, or nanoMDM2. (D) Cumulative distribution histograms of Alexa647 fluorescence for BBS-Q1-YFP co-expressed with either nanobody (black line) or nanoCHIP (red line), nanoNSlmb (blue line), or nanoMDM2 (orange line).
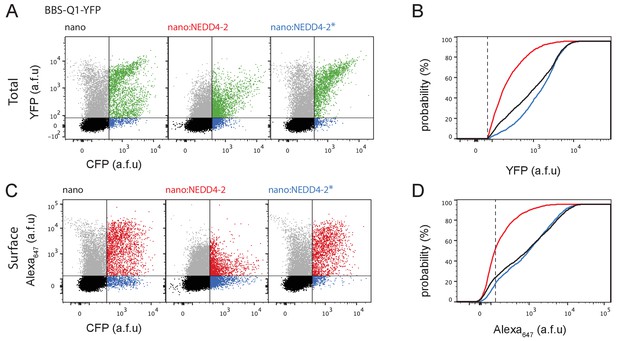
Engineered nanoNEDD4-2 decreases both Q1 surface density and total protein expression.
(A) Flow cytometry analyses of total Q1 expression (YFP fluorescence) in cells expressing BBS-Q1-YFP + nanobody (left, control), BBS-Q1-YFP + nanoNEDD4-2 (center), and BBS-Q1-YFP + nanoNEDD4-2* [C942S] (right). (B) Cumulative distribution histograms of YFP fluorescence for BBS-Q1-YFP co-expressed with either nanobody alone (black line), nanoNEDD4-2 (red line), and nanoNEDD4-2* [C942S] (blue line). (C) Flow cytometry analyses of surface Q1 channels (Alexa647 fluorescence) in cells expressing BBS-Q1-YFP + nanobody (left, control), BBS-Q1-YFP + nanoNEDD4-2 (center), and BBS-Q1-YFP + nanoNEDD4-2* [C942S] (right). (D) Cumulative distribution histograms of Alexa647 fluorescence for BBS-Q1-YFP co-expressed with either nanobody alone (black line), nanoNEDD4-2 (red line), and nanoNEDD4-2* [C942S] (blue line).
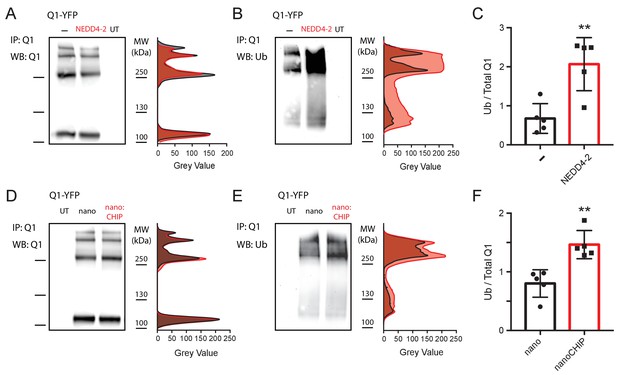
nanoCHIP increases Q1 ubiquitination.
(A) Left, Q1 pulldowns probed with anti-Q1 antibody from HEK293 cells expressing Q1-YFP ± NEDD4-2, and untransfected controls (UT). Right, densitometric analyses of anti-Q1 Western blot bands. (B) Left, Anti-ubiquitin labeling of the stripped Western blot from (A). Right, densitometric analyses of anti-ubiquitin Western blot bands. (C) Relative Q1 ubiquitination computed by ratio of anti-ubiquitin to anti-Q1 signal intensity. (D–F) Anti-Q1 and anti-ubiquitin Western blot signals from HEK293 cells expressing Q1-YFP with either nanobody alone or nanoCHIP. Same format as (A–C). **p<0.01, Student’s t test.
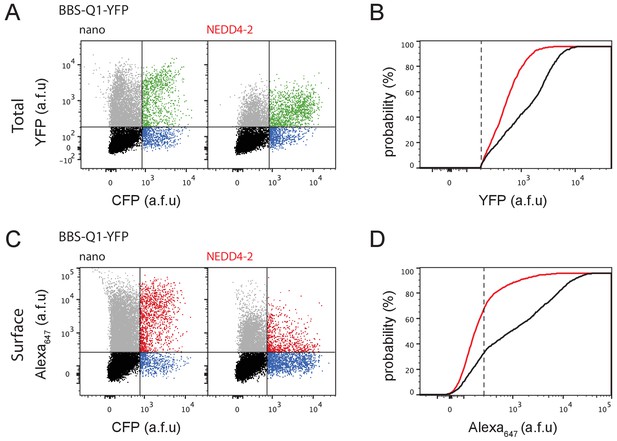
Full-length NEDD4-2 diminishes both Q1 surface density and total protein expression.
(A) Flow cytometry analyses of total Q1 expression (YFP fluorescence) in cells expressing BBS-Q1-YFP + nanobody (left, control) or BBS-Q1-YFP + NEDD4-2 (right). (B) Cumulative distribution histograms of YFP fluorescence for BBS-Q1-YFP co-expressed with either nanobody alone (black line) or NEDD4-2 (red line). (C) Flow cytometry analyses of surface Q1 channels (Alexa647 fluorescence) in cells expressing BBS-Q1-YFP + nanobody (left, control) or BBS-Q1-YFP + NEDD4-2 (right). (D) Cumulative distribution histograms of Alexa647 fluorescence for BBS-Q1-YFP co-expressed with either nanobody alone (black line) or NEDD4-2 (red line).
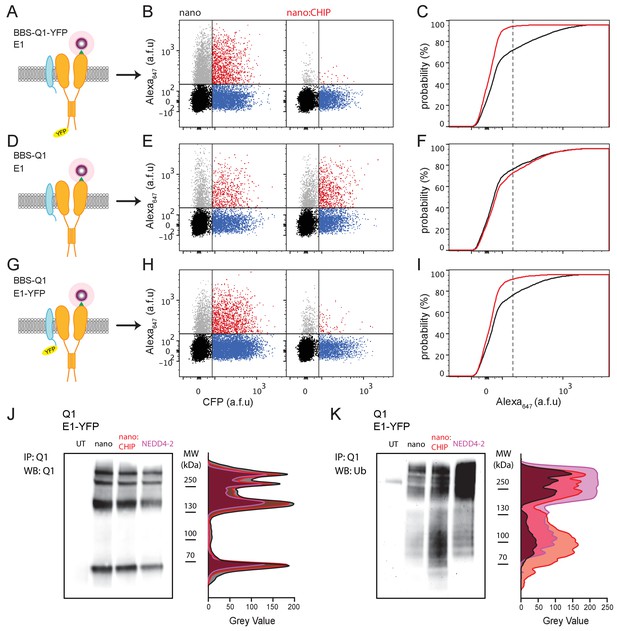
nanoCHIP regulation of Q1/KCNE1 complex surface density.
(A) Cartoon of BBS-Q1-YFP + KCNE1. (A) Flow cytometry analyses of surface Q1 channels (Alexa647 fluorescence) in HEK293 cells expressing BBS-Q1-YFP + KCNE1 with either nanobody (left, control) or nanoCHIP (right). (C) Cumulative distribution histograms of Alexa647 fluorescence for BBS-Q1-YFP co-expressed with either nanobody (black line) or nanoCHIP (red line). (D–F) Schematic, flow cytometry analyses, and cumulative distribution histograms from cells expressing BBS-Q1 + KCNE1 with either nanobody alone or nanoCHIP. Same format as (A–C). (G–I) Schematic, flow cytometry analyses, and cumulative distribution histograms from cells expressing BBS-Q1 + KCNE1-YFP with either nanobody alone or nanoCHIP. Same format as (A–C). (J) Left, Q1 pulldowns probed with anti-Q1 antibody from HEK293 cells expressing Q1 + KCNE1-YFP with nanobody alone, nanoCHIP, or NEDD4-2. UT, untransfected cells. Right, densitometric analyses of anti-Q1 Western blot bands for the different conditions. (K) Left, Anti-ubiquitin labeling of the stripped Western blot from (J). Right, densitometric analyses of anti-ubiquitin Western blot bands.
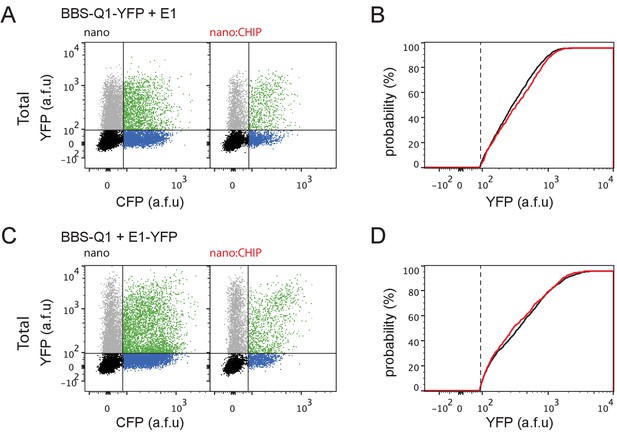
nanoCHIP has no effect on Q1/E1 total protein expression.
(A) Flow cytometry analyses of total Q1 expression (YFP fluorescence) in cells expressing BBS-Q1-YFP + KCNE1 with either nanobody (left, control) or nanoCHIP (right). (B) Cumulative distribution histograms of YFP fluorescence for BBS-Q1-YFP + KCNE1 co-expressed with either nanobody (black line) or nanoCHIP (red line). (C,D) Flow cytometry analyses, and cumulative distribution histograms from cells expressing BBS-Q1 + KCNE1-YFP with either nanobody alone or nanoCHIP. Same format as (A,B).
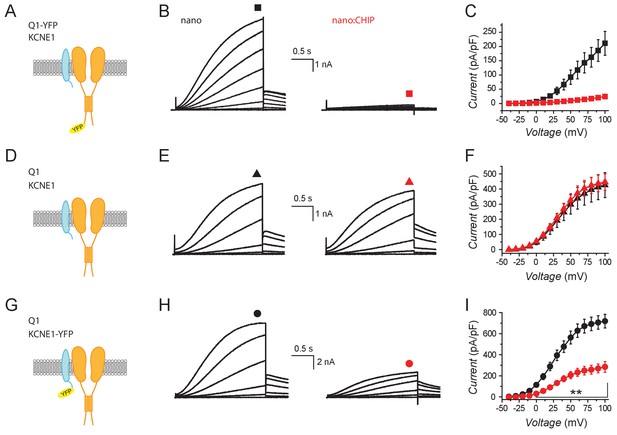
Functional knockdown of reconstituted Iks by nanoCHIP.
(A) Schematic, Q1-YFP + KCNE1. (B) Exemplar family of IKs reconsitituted in CHO cells expressing Q1-YFP + KCNE1 with either nanobody alone (left) or nanoCHIP (right). (C) Population I-V curves for nano (■, n = 5) and nanoCHIP (
, n = 5). (D–F) Schematic, exemplar currents and population I-V curves for CHO cells expressing Q1 + KCNE1 with either nanobody (▲, n = 14) or nanoCHIP ( , n = 12). Same format as (A–C). (G–I) Schematic, exemplar currents and population I-V curves for CHO cells expressing Q1 + KCNE1-YFP with either nanobody (●, n = 13) or nanoCHIP ( , n = 8). Same format as (A–C). **p<0.01, Student's t test.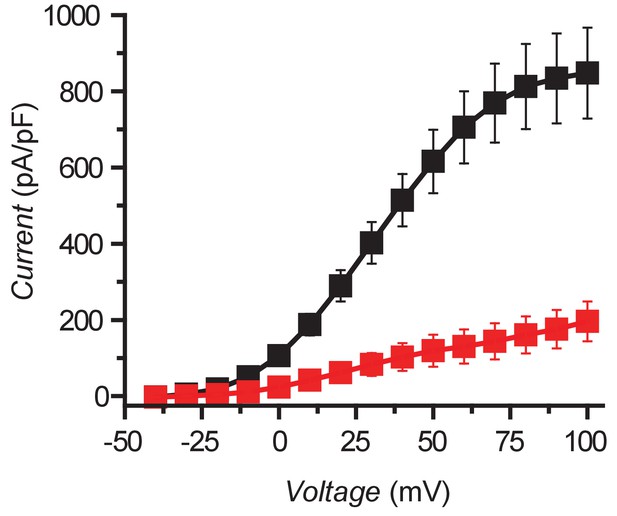
Functional knockdown of Iks reconstituted in HEK293 cells by nanoCHIP.
Population I-V curves from HEK293 cells expressing Q1-YFP + KCNE1 with either nanobody alone (■, n = 9) or nanoCHIP (
, n = 5).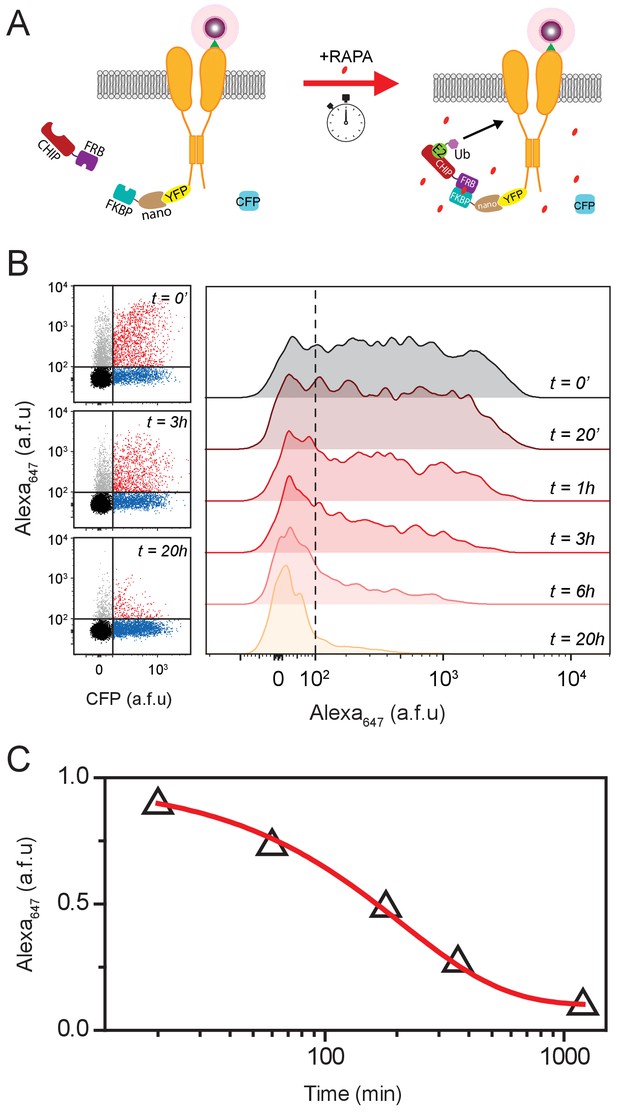
A small-molecule-inducible system for temporal control of Q1 surface expression.
(A) Cartoon showing FKBP/FRB heterodimerization strategy for rapamycin-induced recruitment of engineered E3 ligase (iN-CHIP) to Q1-YFP. (B) Representative flow cytometry dot plots (left) and histograms (right) showing evolution of surface Q1 channels in cells expressing BBS-Q1-YFP + FRB-CHIP + FKBP-nano at varying time intervals after rapamycin induction. (C) Plot of normalized mean Q1 surface density (Alexa647 fluorescence) as a function of time after rapamycin induction (Δ, n = 1355–1523 cells; N = 3). Smooth curve is an exponential decay function fit to the data: , with A = 0.87 ± 0.03, y0 = 0.10 ± 0.02, τ = 208.8 ± 17.4 mins.
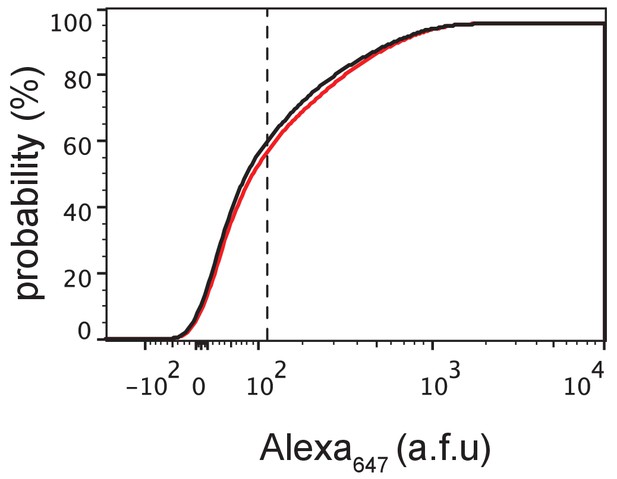
. iN-CHIP does not modulate surface expression of untagged BBS-Q1.
Cumulative distribution histograms of Alexa647 fluorescence in cells expressing BBS-Q1 + FKBP-nano + FRB-CHIP after addition of DMSO (black) or rapamycin (red) for 20 hr.
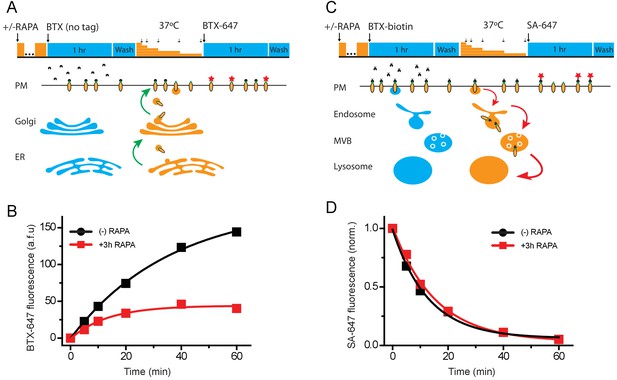
iN-CHIP selectively impairs Q1-YFP forward trafficking.
(A) Schematic showing optical pulse-chase assay for measuring BBS-Q1-YFP forward trafficking. Cells expressing BBS-Q1-YFP + FRB-CHIP + FKBP-nano were induced with rapamycin and initial surface channels blocked by incubation with untagged α-bungarotoxin (BTX) at 4°C. Cells were washed and placed back at 37°C for varying time intervals (5, 10, 20, 40, 60 min) to resume delivery of new channels to the surface membrane. Newly delivered channels were labeled with Alexa Fluor 647 conjugated BTX (BTX647) at 4°C and analyzed using flow cytometry. (B) Time evolution of BBS-Q1-YFP delivery to the surface without (●, n = 2878–3905 cells; N = 2) or with (
, n = 2990–3469 cells; N = 2) rapamycin induction. Smooth curves are fits of an exponential growth function to the data: . For ●; A = −45.1 ± 3.8, y0 = 44.1 ± 3.0, τ = 13.4 ± 3.0 mins. For ; A = −180.5 ± 7.0, y0 = 179.9 ± 7.7, τ = 36.1 ± 3.2 mins. (C) Schematic showing optical assay for measuring BBS-Q1-YFP internalization. Cells expressing BBS-Q1-YFP + FRB-CHIP + FKBP-nano were induced with rapamycin and initial surface channels labeled with biotin-conjugated BTX (BTX-biotin) at 4°C. Cells were washed and incubated at 37°C for varying time intervals (5, 10, 20, 40, 60 min) to allow for internalization of surface channels. The remaining surface channels were labeled with Alexa Fluor 647-conjugated streptavidin (SA-647) at 4°C. (D) Time evolution of loss of surface BBS-Q1-YFP channels without (●, n = 3430–4919 cells; N = 2) or with ( , n = 3336–4744 cells; N = 2) rapamycin induction. Smooth curves are fits of an exponential decay function to the data: . For ●; A = 0.93 ± 0.03, y0 = 0.06 ± 0.02, τ = 12.8 ± 1.1 mins. For ; A = 0.98 ± 0.03, y0 = 0.03 ± 0.03, τ = 15.3 ± 1.3 mins.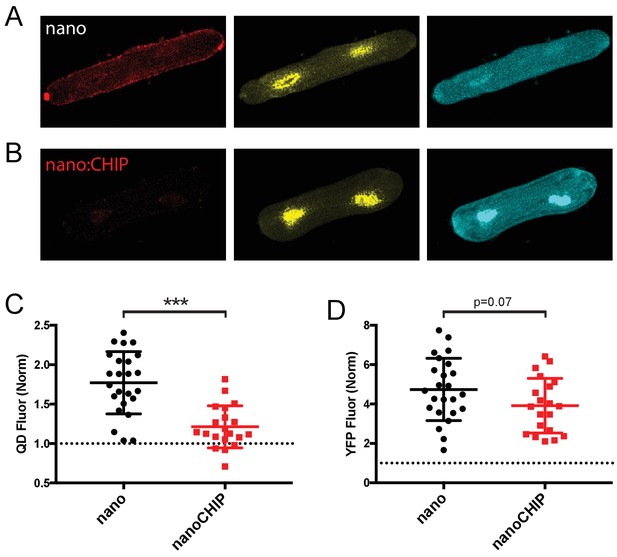
nanoCHIP eliminates Q1 surface expression in cardiomyocytes.
(A) Exemplar adult rat cardiomyocyte expressing BBS-Q1-YFP + nanobody-P2a-CFP. Fluorescence shows surface Q1 (QD655 signal, left), total Q1 (YFP signal, middle) and marker for nanobody expression (CFP signal, right). (B) Exemplar cardiomyocyte co-expressing BBS-Q1-YFP and nanoCHIP. Same format as (A). (C) Comparison of surface Q1 channels in cardiomyocytes co-expressing either nanobody (●, n = 24) or nanoCHIP (
, n = 20). ***p<0.0001, Student’s unpaired t test. (D) Comparison of total Q1 channels in cardiomyocytes co-expressing either nanobody (●, n = 24) or nanoCHIP ( , n = 20).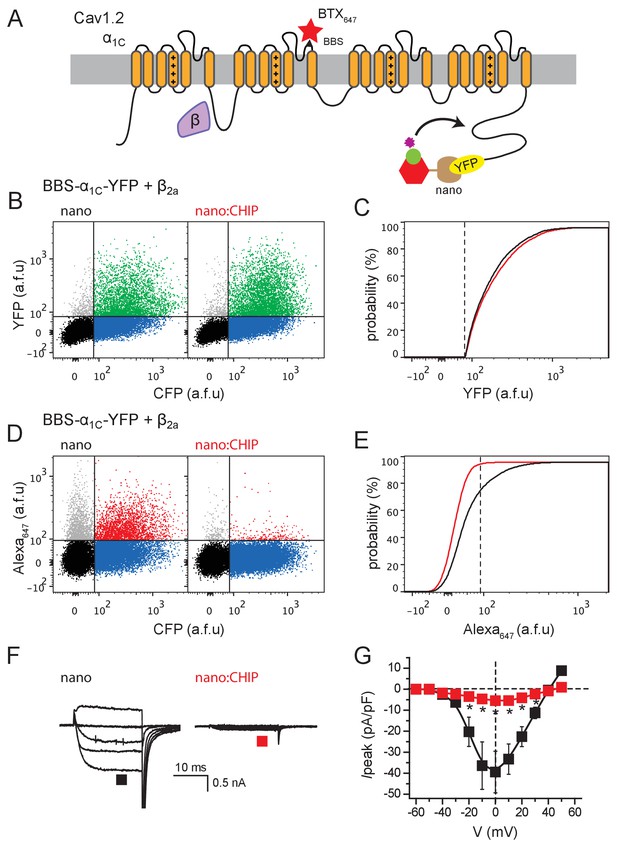
Functional knockdown of recombinant Cav1.2 channels by nanoCHIP.
(A) Schematic of CaV1.2 pore-forming α1C and auxiliary β subunit complex. An extracellular BBS epitope tag placed on the domain II S5-S6 loop permits detection of surface channels using Alex Fluor647-conjugated bungarotoxin. A YFP tag on α1C C-terminus reports on the total expression and serves as a docking site for nanoCHIP. (B) Flow cytometry analyses of total α1C expression (YFP fluorescence) in cells expressing BBS-α1C-YFP + β2a together with either nanobody (left, control) or nanoCHIP (right). (C) Cumulative distribution histograms of YFP fluorescence for BBS-α1C-YFP + β2a co-expressed with either nanobody (black line) or nanoCHIP (red line). (D) Flow cytometry analyses of surface α1C (Alexa647 fluorescence) in cells expressing BBS-α1C-YFP + β2a + nanobody (left, control) or BBS-α1C-YFP + β2a + nanoCHIP (right). (E) Cumulative distribution histograms of Alexa647 fluorescence for BBS-α1C-YFP + β2a co-expressed with either nanobody (black line) or nanoCHIP (red line). (F) Exemplar Ba2+ currents from CaV1.2 channels reconstituted in HEK293 cells expressing α1C-YFP + β2a with either nanobody alone (left) or nanoCHIP (right). (G) Population I-V curves for α1C-YFP + β2a reconstituted with either nano (■, n = 10) or nanoCHIP (
, n = 14). *p<0.05, Student's unpaired t test.Tables
Reagent type (species) or resource | Designation | Source or reference | Identifiers | Additional information |
---|---|---|---|---|
gene (human) | KCNQ1 | NM_000218 | ||
gene (rabbit) | Cav1.2 | NM_001136522 | ||
strain, strain background (E. coli) | XL10-Gold | Agilent | ||
cell line (human) | HEK293 | other | RRID:CVCL_0045 | Laboratory of Robert Kass |
cell line (human) | CHO | ATCC | RRID:CVCL_0214 | CHO-K1, ATCC, CCL-61 |
recombinant DNA reagent | nano-P2A-CFP | this paper | Made from GFP-nanobody (vhhGFP4) (Kubala et al., 2010); see molecular biology and cloning | |
recombinant DNA reagent | nanoCHIP-P2A-CFP | this paper | Made by gene synthesis (Genewiz); see molecular biology and cloning | |
recombinant DNA reagent | nanoCHIP*-P2A-CFP | this paper | Made by PCR; see molecular biology and cloning | |
recombinant DNA reagent | nanoNSlmb-P2A-CFP | this paper; PMID: 22157958 | Made from pcDNA3_NSlmb-vhhGFP4 (Addgene #35579) (Caussinus et al., 2011); see molecular biology and cloning | |
recombinant DNA reagent | nanoMDM2-P2A-CFP | this paper | Made from pcDNA3 MDM2 WT (Addgene #16233) (Zhou et al., 2001); see molecular biology and cloning | |
recombinant DNA reagent | CFP-P2A-nanoNEDD4-2 | this paper | Made from PCI_NEDD4L (Addgene #27000) (Gao et al., 2009); see molecular biology and cloning | |
recombinant DNA reagent | CFP-P2A-nanoNEDD4-2* | this paper | Made by site-directed mutagenesis of above; see molecular biology and cloning | |
recombinant DNA reagent | CFP-P2A-NEDD4-2 | this paper | Made from PCI_NEDD4L (Addgene #27000) (Gao et al., 2009); see molecular biology and cloning | |
recombinant DNA reagent | iN-CHIP | this paper | FRB:CHIP-P2A-CFP-P2A-FKBP:nano; see molecular biology and cloning | |
recombinant DNA reagent | BBS-KCNQ1-YFP | PMID: 25344363 | ||
recombinant DNA reagent | BBS-KCNQ1 | this paper | ||
recombinant DNA reagent | KCNQ1-YFP | PMID: 25344363 | ||
recombinant DNA reagent | KCNQ1 | PMID: 25344363 | ||
recombinant DNA reagent | KCNE1-YFP | PMID: 25344363 | ||
recombinant DNA reagent | KCNE1 | PMID: 25344363 | ||
recombinant DNA reagent | BBS-a1C-YFP | PMID: 20308247; PMID: 24003157 | ||
recombinant DNA reagent | a1C-YFP | PMID: 20308247 | ||
recombinant DNA reagent | B2a | PMID: 20308247 | ||
biological sample (R. norvegicus) | Adult Heart Ventricular Cells | PMID: 19532115 | ||
antibody | Anti-Q1 antibody, APC-022 | Alomone | RRID:AB_2040099 | 1:1000 |
antibody | Anti-Ubiquitin, VU1 | LifeSensors | 1:500 | |
peptide, recombinant protein | Protein A/G Sepharose beads | Rockland | ||
peptide, recombinant protein | a-bungarotoxin, Alexa Fluor 647 conjugate | Life Technologies | ||
peptide, recombinant protein | a-bungarotoxin, Biotin-XX conjugate | Life Technologies | ||
peptide, recombinant protein | a-bungarotoxin | Life Technologies | ||
peptide, recombinant protein | Streptavidin, Alexa Fluor 647 conjugate | Life Technologies | ||
peptide, recombinant protein | Streptavidin, Qdot 655 conjugate | Life Technologies | ||
commercial assay or kit | AdEasy Adenoviral Vector Systems | Stratagene | ||
commercial assay or kit | X-tremeGENE HP DNA Transfection Reagent | Roche | ||
commercial assay or kit | QuikChange Lightning Site-Directed Mutagenesis Kit | Stratagene | ||
chemical compound, drug | Rapamycin | Sigma | ||
software, algorithm | FlowJo | RRID:SCR_008520 | ||
software, algorithm | PulseFit | HEKA | ||
software, algorithm | Origin | RRID:SCR_014212 | ||
software, algorithm | Graphpad Prism | RRID:SCR_002798 |
Additional files
-
Transparent reporting form
- https://doi.org/10.7554/eLife.29744.020