Neurexin and Neuroligin-based adhesion complexes drive axonal arborisation growth independent of synaptic activity
Figures
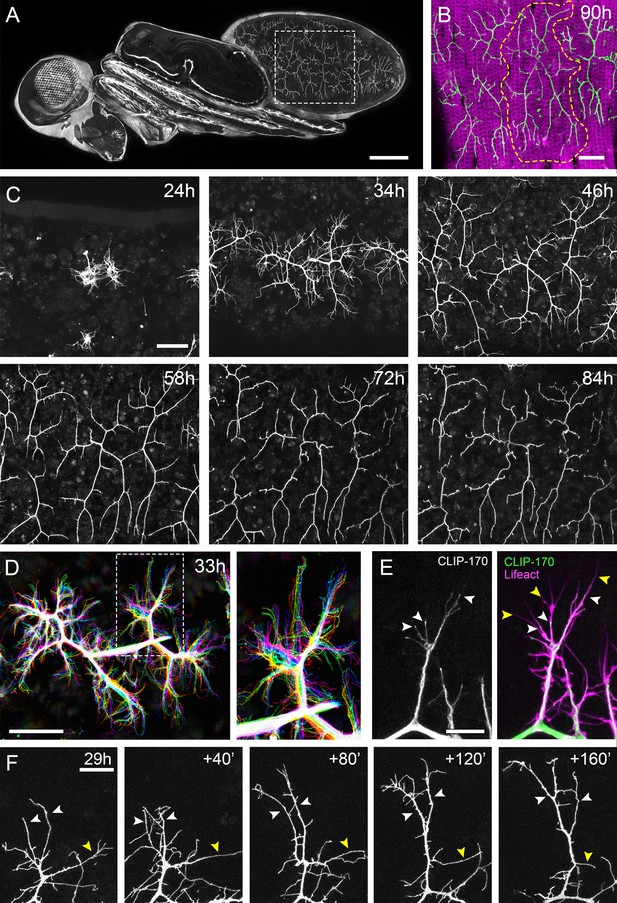
The pleural motoneurons show dynamic growth during development.
(A) A dorso-lateral view of a pupa at 90 hr APF expressing UAS-myr::GFP in the pattern of the glutamatergic driver OK371-GAL4. Dashed lines demarcate arborisations innervating segments A3 and 4. (B) Innervation of the pleural muscles in segments A3 and 4 at 90 hr APF. Muscles labelled with mCD8::ChRFP (Mef2-GAL4; magenta; Ranganayakulu et al., 1996) and motoneurons labelled with myr::GFP (VGlut-LexA; Diao et al., 2015; green). Dashed lines demarcate the anterior arborisation in 4A (A4A). (C) A time series follows the growth of arborisations in segment A3 from 24 hr to 84 hr APF in 10–14 hr intervals (VGlut-LexA > myr::GFP). (D) A 10 min time-lapse (temporally colour coded every 2 min) reveals the dynamic growth of a pair of arborisations in segment A3 at 32 hr APF. (E) Subcellular localisations of cytoskeletal components. Microtubules, revealed by CLIP-170::GFP, are concentrated in branches and dynamically invade nascent branchlets (white arrowheads). Filopodia (yellow arrowheads), are rich in actin, revealed by Lifeact::Ruby, but largely devoid of microtubules. (F) A time series with 40 min intervals shows the growth of a branch at 29 hr APF. White arrowheads indicate filopodia that become stabilised and mature into branches. The yellow arrowhead indicates a branch that retracts into a filopodium. Scale bars: 250 µm (A), 50 µm (B,C,D), 10 µm (E), 20 µm (F).
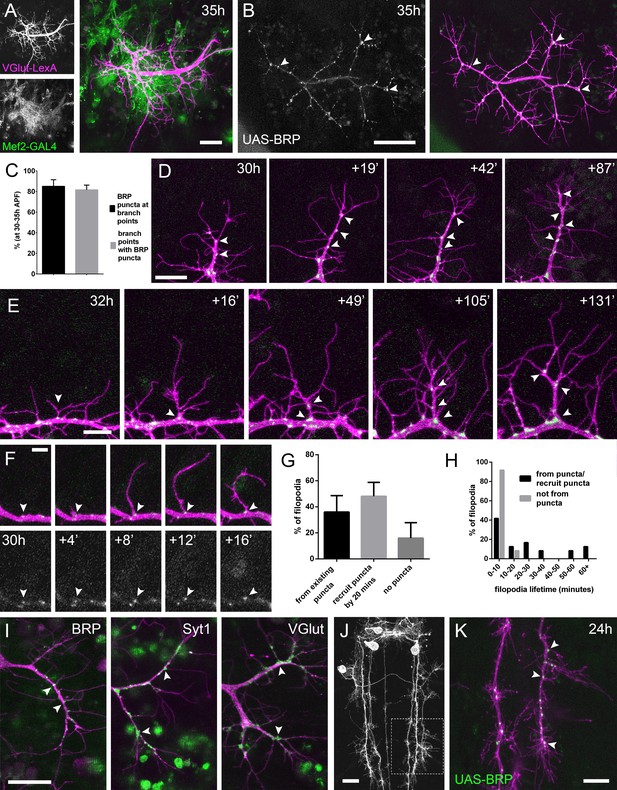
The arrival and localisation of presynaptic components is tightly linked with arbor growth.
(A) Muscles and motoneurons development in close proximity at 35 hr APF. Muscles labelled with myr::tdTomato (Mef2-GAL4) and motoneurons labelled with myr::GFP (VGlut-LexA). (B) A pair of motoneuron axon terminals expressing BRP::RFP (green) and myr::GFP (magenta) under the control of OK371-GAL4 at 35 hr APF. BRP::RFP is particularly clear at branch points (arrowheads). (C) At stages 30–35 hr APF, 85.05 ± 6.42% of BRP::RFP puncta are localised at branch points or the bases of filopodia and 81.62 ± 4.64% of branch points/bases of filopodia host a punctum (n = 5). (D) BRP::RFP puncta are establish following the growth of axon branches. The time series reveals the rapid arrival of BRP::RFP puncta (green) in the growth cone of an extending branch (arrowheads), producing a branch segment studded with puncta. Most BRP::RFP puncta mark sites of filopodia growth. (E) A time series revealing the maturation of a filopodium that originates from a BRP::RFP punctum (arrowhead in first frame) into a branch. Additional BRP::RFP puncta (arrowheads) are rapidly recruited to new branch nodes. (F) Time series shows the emergence of a filopodium from a site marked by a BRP::RFP punctum (arrowhead). (G) The proportions of filopodia which (i) emerge from existing puncta, (ii) recruit a punctum to their base within 20 min and (iii) do not recruit puncta to their bases (83 filopodia from three time-lapse movies at stages 30–33 hr APF). (H) Lifetimes of filopodia which originate from/recruit puncta within 20 min of emergence (21.67 ± 20.30 mins, n = 24) or do not recruit puncta (3.83 ± 2.76 mins, n = 24). Filopodia that originate from puncta/recruit puncta are significantly longer lived than those that do not recruit puncta (Mann-Whitney U = 73, p<0.0001, two-tailed). (I) Protein trap lines reveal the localisation of endogenous BRP, Syt1 and VGlut (MiMIC collection; Venken et al., 2011; arrowheads). Arborisations staged between 30 hr and 33 hr APF. (J and K) Eve + ve interneuron in T3, at 24 hr APF, (from RN2-FLP, Tub-FRT-CD2-FRT-GAL4) with punctate BRP::RFP (arrowheads) concentrated at branch points and bases of filopodia in growing output arborisations. Area with dashed lines in (J) is expanded in (K). Bars represent SDs. Scale bars: 20 µm (A,D,I,J), 50 µm (B), 10 µm (E,K), 5 µm (F).
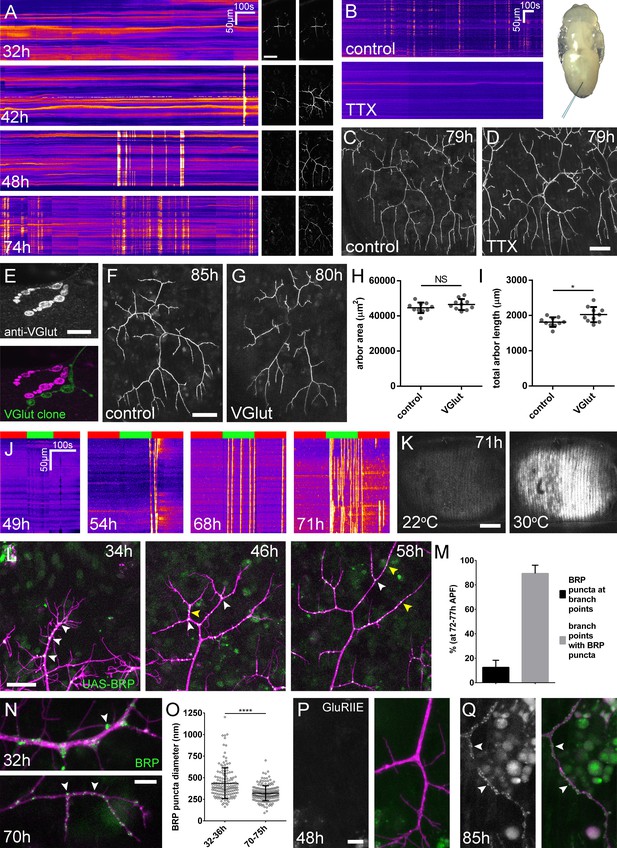
Arbor growth takes place without the formation of functional synapses.
(A) Calcium activity in PM-Mn axon terminals at 32, 42, 48 and 74 hr APF, measured by changes in GCaMP6m fluorescence (Δf) (driven by OK371-GAL4). Sequential images show examples of Δfs displayed as heat registered kymographs (B) GCaMP6m Δfs in PM-Mn axon terminals at 78 hr APF in a pupa injected with a control solution of PBS (top) and a solution of 0.5 mM TTX in PBS (bottom) into the abdomen of a pupa (inset). (C) Arborisations in segment A3 (OK371-GAL4 > myr::GFP) at 79 hr APF following injection at 32 hr APF with a PBS solution and (D) solution containing TTX. (E) Motoneuron axon terminal of a VGlut null MARCM clone (green) alongside a VGlut heterozygous terminal in L3 larva stained with an antibody against VGlut (magenta). (F) The anterior-most arborisation in segment A3 (A3–A) of a heterozygous VGlut control expressing myr::GFP and mCD8::GFP (VGlutNMJX-GAL4) at 85 hr APF (G) an equivalent arborisation in VGlut null MARCM clone at 80 hr APF. (H and I) Arborisations of VGlut null A3-A MARCM clones do not differ significantly from controls in arbor area (controls: 44643 ± 2982 µm2, VGlut: 46518 ± 3083 µm2, n1,2 = 10, t(18) = 1.38, p=0.18, t-test, two-tailed). Marginally greater total arbor lengths (controls: 1812 ± 134 µm, VGlut: 2024 ± 216 µm, n1,2 = 10, t(18) = 2.64, p=0.02, t-test, two-tailed). (J) Muscle GCaMP6m (Mef2-GAL4) Δf in response to motoneuron activation at 49 hr, 54 hr, 68 hr and 71 hr APF, using the warmth-gated ion channel TRPA1 (VGlut-LexA > dTRPA1). Red bars indicate time at the restrictive temperature (22°C), green bars indicate time at the permissive temperature (30°C). (K) Images show muscle GCaMP6m Δfs before and after activation of motoneurons with dTRPA1 at 71 hr APF. (L) Organisation of BRP::RFP puncta through development. Time series of the same arbor segment at 34 hr, 48 hr and 56 hr APF shows a shift from puncta at branch points (white arrowheads) to a distribution along branch lengths (yellow arrowheads). (M) Between 72 hr and 77 hr APF, 12.55 ± 5.79% of total BRP::RFP puncta are found at branch points/bases of filopodia, yet the majority (89.38 ± 6.73%) of branch points/bases of filopodia host puncta (n = 5). (N) Size and distribution of endogenous, GFP labelled BRP. The puncta of BRP (indicated by arrowheads) are larger and more heterogenous in diameter during early arbor growth (32 hr APF) than at later stages (70hAPF), when the major phase of outgrowth has ceased (OK371-GAL4 > mCD8::ChRFP). (O) Diameters of endogenous BRP::GFP puncta measured as the full width at half maximum of peaks in fluorescence are significantly greater at 32 hr APF (435.8 ± 177.8 nm, n = 144) than at 72 hr APF (319.6 ± 87.9 nm, n = 177) (Mann-Whitney U = 6935, p<0.0001, two-tailed). (P) Localisation of GluRIIE at 48 hr APF. This GFP tagged version of GluRIIE (FlyFos; Sarov et al., 2016) driven under the control of the native transcriptional unit cannot be seen in the postsynaptic membrane before or at 48 hr APF. (OK371-GAL4 > mCD8::ChRFP). (Q) At 85 hr APF conspicuous GluRIIE::GFP clusters (arrowheads) are found along the axon terminals. Bars represent SDs. Scale bars: 50 µm (A,C,D,F,G), 10 µm (E), 100 µm (K), 25 µm (L), 5 µm (N,P,Q).
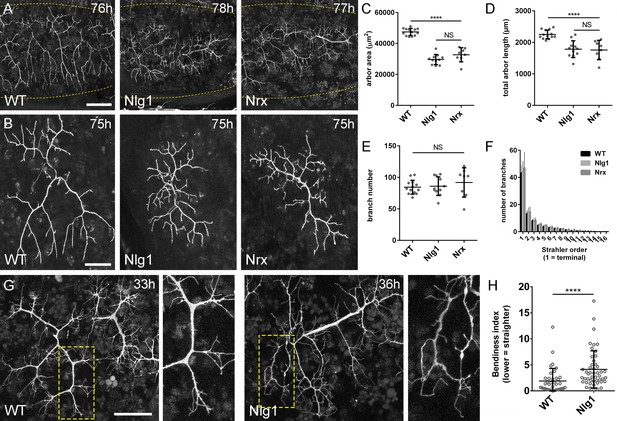
Neurexin and Neuroligin 1 are required during arbor growth.
(A) Arborisations of PM-Mn axons in segments A3-A5 in a wild-type control, an Nlg1 null and an Nrx null staged between 76 hr and 78 hr APF (VGlut-LexA > myr::GFP). Yellow dots show the outline of epidermis (B) Anterior-most arborisations in segment A3 (A3–A) at stages between 75 hr and 85 hr APF. Arborisations belonging to other motoneurons alongside have been removed using image processing. (C–F) Morphometric analysis of A3-A arborisations of Nlg1 nulls (n = 11), Nrx nulls (n = 8) and wild-type controls (n = 13) staged from 75 hr to 85 hr APF. (C) Nlg1 and Nrx nulls cover significantly lower territories (controls: 47445 ± 2298 µm2, Nlg1: 29616 ± 3259 µm2, Nrx: 32696 ± 4565 µm2. Nlg1 vs controls: t(22) = 15.67, p<0.0001. Nrx vs controls: t(19) = 9.89, p<0.0001. t-tests, two-tailed) (D) Total PM-Mn arbor lengths controls: 2251 ± 140 µm, Nlg1: 1785 ± 265 µm, Nrx: 1758 ± 306 µm. Nlg1 vs controls: t(22) = 5.51, p<0.0001. Nrx vs controls: t(19) = 5.07, p<0.0001. t-tests, two-tailed), are significantly different. (E) Total number of PM-Mn branch numbers are not significant (controls: 84.38 ± 10.97, Nlg1: 86.00 ± 14.74, Nrx: 91.88 ± 23.27. Nlg1 vs controls: t(22) = 0.31, p=0.76. Nrx vs controls: t(19) = 1.00, p=0.33. t-tests, two-tailed). (F) Nlg1 nulls, Nrx nulls and controls were no different in their topological organisation. Nulls did not differ from controls in their total number of highest order (terminal) branches (controls: 43.31 ± 5.59, Nlg1: 44.27 ± 7.56, Nrx: 47.00 ± 11.50. Nlg1 vs controls: t(22) = 0.36, p=0.72. Nrx vs controls: t(19) = 0.99, p=0.33. t-tests, two-tailed) or in their total number of orders (controls: 11.62 ± 1.94, Nlg1: 11.27 ± 2.15, Nrx: 11.88 ± 2.36. Nlg1 vs controls: t(22) = 0.41, p=0.67. Nrx vs controls: t(19) = 0.27, p=0.79. t-tests, two-tailed). (G) At early stages of outgrowth Nlg1 null arborisations possess a number of branches that grown precociously, yet are more tortuous and with fewer side branches than wild-types. (H) Branch bendiness of PM--Mns arbors between 32 hr and 36 hr APF. Bendiness was measured as the percentage difference between the actual length and the straight-line length of primary (terminal) and secondary branches. Branches of Nlg1 nulls are less straight (4.13 ± 3.57%, n = 48) than controls (1.89 ± 2.41%, n = 38) at stages between 32 hr and 36 hr APF (Mann-Whitney U = 441, p<0.0001, two-tailed). Bars represent SDs. Scale bars: 100 µm (A), 50 µm (B,G).
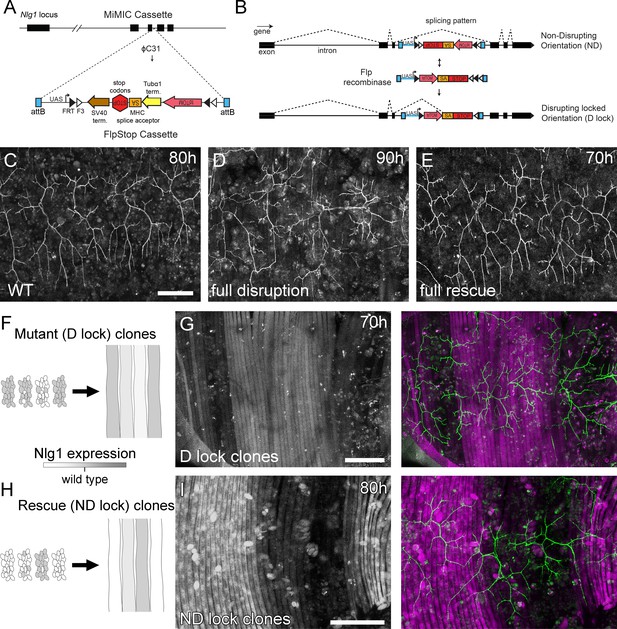
Mosaic analysis shows a local role for endogenous Neuroligin 1 during arbor growth.
(A) A schematic shows the directed insertion of the FlpStop construct into the third coding intron of the Nlg1 genomic locus by recombinase mediated cassette exchange (RMCE) with a MiMIC integration site. (B) A schematic shows the mechanism of FlpStop action for the conditional disruption of endogenous Nlg1. In the initially non-disrupting (ND) orientation the splice acceptor and stop codons are on the non-coding strand and thus do not effect gene function. Upon inversion by FLP recombinase the splice acceptor and stop codons are brought into frame on the coding strand, resulting in disrupted gene expression. The cassette is locked into this disrupting (D-lock) orientation by a FLEx switch (Schnütgen et al., 2003). In addition, inversion brings the coding sequence for tdTomato into proximity with a UAS sequence, enabling GAL4 driven expression. FlpStop lines were also generated in the initially disrupting (D) orientation, which allows rescue of Nlg1 (ND-lock clones) and ‘turns on’ tdTomato (Schematic not shown). (C) Arborisations at 80 hr APF in a wild-type control (VGlut-LexA > myr::GFP). (D) Complete induction of Nlg1 D-lock (disrupting) clones using hsFlp and a long heat-shock protocols produces arbor growth defects comparable to Nlg1 mutants. (E) Germline ND-lock rescues arbor growth to a near wild-type phenotype. (F) Schematic shows the formation of Nlg1 deficient fibres from the fusion of D-lock clonal myoblasts induced using hsFlp and a short heat shock at larval L3 stage. (G) Arbor growth (visualised with VGlut-LexA) onto regions of D-lock muscle clones (Mef2-GAL4) is disrupted, whereas arbor growth on non-clonal regions is close to wild-type. (H) Model shows the rescue of Nlg1 expression in clonal fibres formed from the fusion of ND-lock clonal myoblasts induced using hsFlp and a short heat shock at larval L3 stage. (I) Arbor growth onto ND-lock muscle clones is close to wild-type, whereas growth on non-clonal regions is disrupted. Scale bars: 100 µm (C,D,E,G,I).
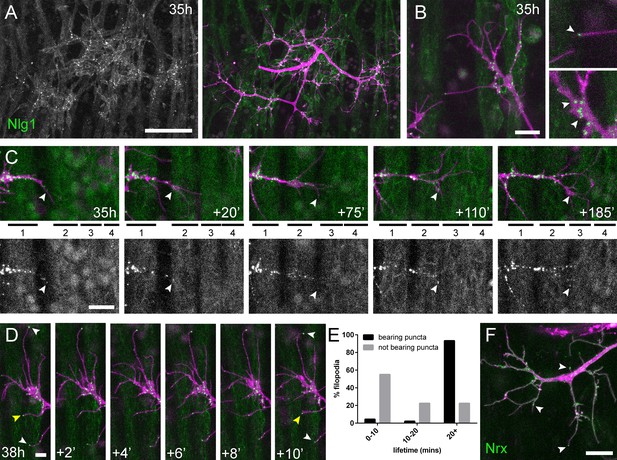
Neuritic Adhesion Complexes (NACs) containing Neurexin and Neuroligin 1 stabilise filopodia during arbor growth.
(A) Nlg1::GFP (Mef2-GAL4; green) expressed in muscles at 35 hr APF forms puncta on the postsynaptic membrane that are arranged exclusively in apposition with the axon terminals (VGlut-LexA > myr::tdTomato; magenta). (B) Higher magnifications show the concentration of Nlg1::GFP puncta at sites of branch growth and at filopodia tips. (C) Rounds of filopodia extension and stabilisation are concomitant with the recruitment of Nlg1::GFP puncta (white arrowheads) to their tips. Time series shows the extension of a branch across a muscle field at 35 hr APF (myotubes numbered from anterior to posterior). (D) Detail of relationship between the dynamics of filopodia and Nlg1::GFP puncta. Puncta (white arrowheads) mark the tips of filopodia that persist. Yellow arrowhead indicates a punctum marking the limit of filopodial retraction. (E) Relationship between lifetimes of filopodia and Nlg1::GFP puncta. Histogram showing lifetimes of filopodia with Nlg1::GFP puncta at their tips (n = 45) and without puncta at their tips (n = 49). Filopodia bearing Nlg1::GFP puncta were significantly longer lived than those without puncta (with puncta: 19.36 ± 2.82 mins, without puncta: 10.73 ± 6.88 mins, Mann-Whitney U = 305.5, p<0.0001, two-tailed). (F) Localisation of Nrx::GFP (OK371-GAL4; green) in PM-Mn growing axonal arborisation at 35 hr APF (mCD8::ChRFP; magenta). Arrowheads indicate puncta positioned on filopodia. Bars represent SDs. Scale bars: 50 µm (A), 10 µm (B,F), 20 µm (C), 5 µm (D).
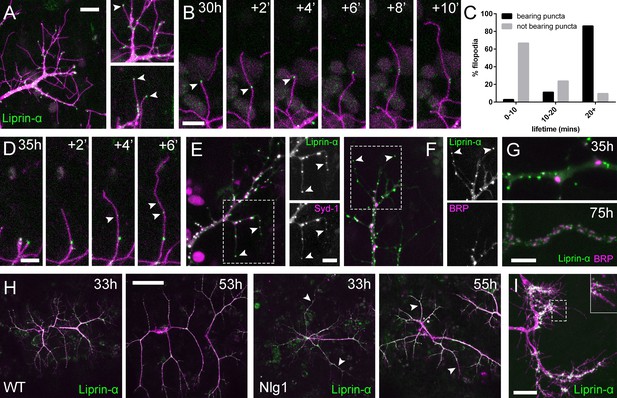
Neuritic Adhesion Complexes (NACs) contain Liprin-α and Syd1.
(A) Localisation of Liprin-α::GFP in growing PM-Mn axonal arborisation at 35 hr APF. Liprin-α::GFP (green) expressed with mCD8::ChRFP (OK371-GAL4; magenta). Cutaway images show Liprin-α::GFP puncta in branches and at tips of filopodia (arrowheads). (B) Detail showing the extension of a filopodium and its retraction only as far as a Liprin-α::GFP punctum (arrowheads). (C) Histogram showing lifetimes of filopodia with Liprin-α::GFP puncta at their tips (n = 36) and without puncta at their tips (n = 21). Filopodia bearing Liprin-α::GFP puncta lived significantly longer than those without puncta (with puncta: 19.44 ± 1.96 mins, without puncta: 8.81 ± 5.88 mins, Mann-Whitney U = 51.5, p<0.0001, two-tailed). (D) Time series shows the rapid precipitation of new Liprin-α::GFP puncta (indicated by arrowheads) within a filopodium. (E) Liprin-α::GFP and Syd-1::Straw puncta co-localise in growing PM-Mn terminals, including at filopodia tips, (arrowheads). Slight misalignment due to sequentially imaging channels. (F) Liprin-α::GFP and BRP::RFP only co-localise at a few sites. BRP::RFP puncta are not found within filopodia. (G) Liprin::GFP and BRP::RFP puncta occupy distinct subcellular regions at 35hAPF. At 75hAPF Liprin::GFP becomes localised to the edges of BRP::RFP puncta along branches. (H) wild-type and Nlg1 null arborisations expressing Liprin-α::GFP and mCD8::ChRFP imaged at 33 hr APF and ~20 hr later. Unusually long, unbranched branches of Nlg1 nulls (white arrowheads) lack Liprin-α::GFP puncta and have collapsed by later stages (I) In Eve + ve interneurons Liprin-α::GFP forms distinct puncta on the filopodia of growing axon terminals at 24 hr APF. Bars represent SDs. Scale bars: 10 µm (A), 5 µm (B,D,E/F,G), 50 µm (H), 20 µm (I).
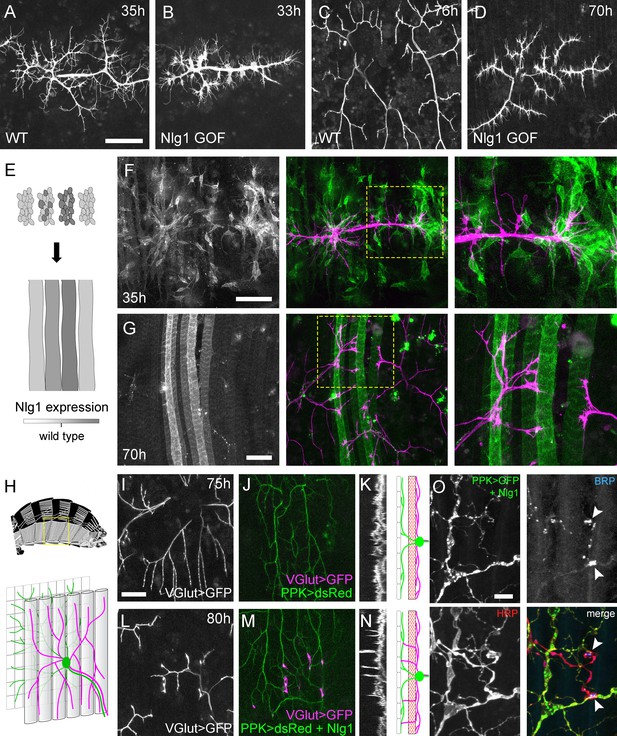
Neuroligin 1 acts locally to drive early arbor growth.
(A–D) Postsynaptic Nlg1 overexpression disrupts PM-Mn axon arbor growth. (A) Control PM-Mn arborisations at 35 hr APF and (B), PM-MN Arborisations at 33 hr APF in a background of postsynaptic Nlg1untagged expression (Mef2-GAL4; VGlut-LexA > myr::GFP). (C) Control arborisations at 76 hr APF. (D) arborisations in a background of muscle specific Nlg1untagged expression at 70 hr APF (E) Schematic of ‘flip-out’ clones generation. Muscle precursor clones induced with hsFlp at larval L3 stages produce clusters of GAL80 negative myoblasts which fuse to form ‘stripes’ of clonal muscle fibres expressing Nlg1untagged and myr::tdTomato. (F) At 35 hr APF, PM-Mn motoneuron branches (magenta) show preferential elaboration onto clonal Nlg1untagged expressing myotubes and myoblast clusters (green). (G) Axonal branches in contact with clonal, Nlg1untagged expressing muscle fibres show a hyper-stabilisation phenotype. Growth of branches appears to be preferentially directed along clonal fibres. (H–O) Ectopic expression of Nlg1 in class IV da sensory neurons drives changes in motoneuron axonal arbor morphology. (H) Schematic shows the relative positions of class IV v’ada sensory input arborisations (green), pleural muscles (grey tubes) and motor axon arborisations (magenta) in the pleural abdominal body wall (schematic of musculature adapted from Demerec, 1950). (I) Motoneuron axon terminals expressing myr::GFP (VGlut-LexA) at 80 hr APF in a control (J) the v’ada input arborisations in the same region expressing dsRed (PPK-Gal4; Grueber et al., 2003). (K) Transverse projection shows the PM-Mn axon arborisations restricted to a single plane. Schematic transverse shows the separation of the v’ada arborisation (green) from the motor axon terminals (magenta) by the pleural muscles (red). (L–N) Nlg1untagged expression in class IV da sensory neurons results in axonal branches that penetrate gaps between the muscle fibres and make contact with the sensory arborisations. These aberrant branches are shown in the transverse view and run perpendicularly to the rest of the arborisation. (O) Abdominal fillet of a newly eclosed adult expressing Nlg1untagged and CD8::GFP in the class IV da sensory neurons. Anti-HRP reveals the motor axon terminals and BRP immunoreactivity reveals presynaptic specialisations at contacts between motor axons and the sensory arborisation (arrowheads). Scale bars: 50 µm (A,B,C,D,F,G), 25 µm (I,J,L,M), 5 µm (O).
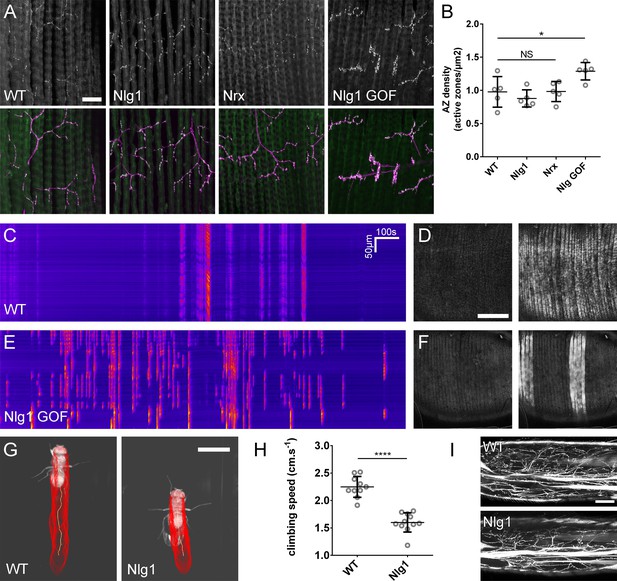
The roles of Nlg and Nrx in NAC dependent arbor construction appear decoupled from synapse formation and neurotransmission.
(A–B) Synapse formation at the light microscope level appears largely unaffected by disruptions to Nrx-Nlg signalling. (A) Active zones at the pleural neuromuscular junctions in adult abdominal fillets from a wild-type control, an Nlg1 null, an Nrx null and an Nlg1 gain-of-function (Mef2-GAL4 > Nlg1 untagged) revealed by antibody staining against BRP. (B) Active zone densities calculated from BRP puncta in synaptic terminals. No significant differences were found between the densities of active zones in controls (0.98 ± 0.23 µm−2, n = 5) and Nlg1 nulls (0.88 ± 0.13 µm−2, n = 5) or controls and Nrx nulls (0.98 ± 0.15 µm−2, n = 5) (Nlg1 vs controls: Mann-Whitney U = 9, p=0.53, two-tailed. Nrx vs controls: Mann-Whitney U = 12, p=0.94, two-tailed). A small, but significant difference was found between active zone densities in Nlg1 gain-of-function terminals (1.29 ± 0.13 µm−2, n = 5) and controls (Mann-Whitney U = 1, p=0.0159, two-tailed). (C–F) Nlg1 overexpression causes changes in PM-Mn axonal arbor morphology, which result in altered connectivity and transmission. (C) GCaMP6m Δfs in a control and in a pupa expressing Nlg1untagged in muscles (Mef2-GAL4) staged within 10 hr of eclosion, (D). In controls, calcium events are generally synchronous across the pleural muscle field whereas in gain-of-function pupae events are often restricted to smaller muscle subsets. This is shown by breaks in the light-coloured bars on the kymograph and in the sequential images (D and F). (G–I) Loss of Nlg1 has a pronounced impact on locomotor ability (G) Automated body tracking performed using FlyLimbTracker (Uhlmann et al., 2017) in Icy (de Chaumont et al., 2012) of a control and an Nlg1 null, 2 days post-eclosion (H) Control flies have a significantly faster climb speed than Nlg1 nulls (controls: 2.25 ± 0.19 cm.s−1, n = 10. Nlg1: 1.60 ± 0.18 cm.s−1, n = 11. t(8.15) = 19, p<0.0001, t-test, two-tailed). (I) Motoneuron axon terminals (VGlut-LexA > myr::GFP) innervating the femur 1 day post-eclosion of an Nlg1 null and of a control. Bars represent SDs. Scale bars: 20 µm (A), 100 µm (D,F,I), 0.2 cm (G).
Videos
PM-Mn axon arbor growth is exploratory and highly dynamic.
A pair of Pm-Mn axon arborisations growing over 15 hr from 35 hr APF (OK371-GAL4 > cytoplasmicGFP). Arbor growth is an exploratory ‘trial-and-error’ process involving high filopodia turnover and the extension and collapse of larger branch segments. Frames taken at 10 min intervals. Time format: hh:mm.
PM-Mn axon arborisations and their synaptic targets grow concurrently and in close association.
PM-Mn axon terminals and their muscle partners interact dynamically during early growth (31 hr APF; OK371-GAL4 + Mef2-GAL4 > cytoplasmicGFP). A branch projecting into the upper right extends across multiple myotubes via rounds of filopodia extension, stabilisation and maturation. Frames taken at 2 min intervals. Time format: hh:mm.
Arbor growth is associated with distinct localisations of presynaptic proteins.
PM-Mn axon arborisations expressing myr::GFP and BRP::RFP at 32 hr APF (OK371-GAL4). BRP::RFP forms puncta which are strongly localised to branch nodes and filopodial bases. Puncta at filopodial bases are correlated with filopodia lifetimes. Frames at 2 min intervals. Time format hh:mm.
The development of PM-Mn activity; 32h APF.
GCaMP6m expressed with OK371-GAL4 reveals the electrical development of the Pm-MNs. At 32 hr APF the lack of large changes in fluorescence indicates an absence of action potentials. Frames taken at 1 s intervals. Time format mm:ss.
The development of PM-Mn activity; 43h APF.
GCaMP6m expressed with OK371-GAL4 reveals the electrical development of the Pm-MNs. By 43 hr APF calcium activity is characterised by large changes in fluorescence indicative of membrane depolarisations. Frames taken at 1 s intervals. Time format mm:ss.
The development of PM-Mn activity; 78h APF.
GCaMP6m expressed with OK371-GAL4 reveals the electrical development of the Pm-MNs. By 78 hr APF activity is defined by bouts of high frequency transients. Frames taken at 1 s intervals. Time format mm:ss.
Nlg1::GFP puncta are correlated with filopodia stability and growth.
Nlg1::GFP (green) in muscles forms puncta which decorate the axon terminals (magenta), particularly at sites of filopodia growth and at the tips of filopodia (38 hr APF; Mef2-GAL4 > Nlg1::GFP, VGlut-LexA > myr::tdTomato). Filopodia which are tipped with stable Nlg1::GFP puncta are significantly longer lived and less motile than those which are not. Filopodia retract only to the point of the last stable Nlg1::GFP punctum. Frames taken at 1 min intervals. Time format: hh:mm.
Nrx::GFP localises to growing axon terminals.
Nrx::GFP expressed in the PM-Mns (OK371-GAL4) faintly coats the axonal membrane, but forms strongly labelled puncta at sites equivalent to Nlg1::GFP i.e. in branch terminals and at the tips of filopodia (35 hr APF). Frames recorded at 1 min intervals. Time format: hh:mm.
Liprin-α::GFP puncta are correlated with filopodia stability and growth.
Liprin-α::GFP (green) expressed in motoneurons (magenta) has a punctate localisation like Nlg1 and Nrx at 35 hr APF. Like Nlg1::GFP, Liprin-α::GFP puncta at filopodial tips are strongly correlated with filopodial stability and regularly mark the limits of filopodial retraction. (OK371-GAL4 > mCD8::Cherry + Liprin-α::GFP). Frames recorded at 30 s intervals. Time format: mm:ss.
Functional consequence of disrupted Nlg1 signalling; control.
GCaMP6m was used as a proxy to explore the effect of disrupted Nlg1 signalling on late-stage postsynaptic activity. In controls, calcium activity within 10 hr prior to eclosion is characterised by changes in fluorescence which are synchronous across the muscle field (Mef2-GAL4 > GCaMP6m). Frames recorded at 1 s intervals. Time format mm:ss.
Functional consequence of disrupted Nlg1 signalling; Nlg1 GOF.
GCaMP6m was used as a proxy to explore the effect of disrupted Nlg1 signalling on late-stage postsynaptic activity. Nlg1 overexpression in muscles results in heterogenous muscle calcium activity, with subsets of muscles firing more frequently than others (Mef2-GAL4 > GCaMP6m + Nlg1untagged). Frames recorded at 1 s intervals. Time format mm:ss.
Tables
Reagent type (species) or resource | Designation | Source or reference | Identifiers | Additional information |
---|---|---|---|---|
genetic reagent (D. melanogaster) | OK371-GAL4 | Bloomington Drosophila Stock Centre | BDSC:26160 | |
genetic reagent (D. melanogaster) | Mef2-GAL4 | Bloomington Drosophila Stock Centre | BDSC:27390 | |
genetic reagent (D. melanogaster) | PPK-GAL4 | Bloomington Drosophila Stock Centre | BDSC:32078 | |
genetic reagent (D. melanogaster) | VGlutNMJX-GAL4 | Daniels et al. (2008) | P{VGlut-GAL4.D}NMJX | |
genetic reagent (D. melanogaster) | VGlut(Trojan)-T2A-LexA | Bloomington Drosophila Stock Centre | BDSC:60314 | |
genetic reagent (D. melanogaster) | Df(2L)vglut2 | Daniels et al. (2006) | ||
genetic reagent (D. melanogaster) | Nlg1I960 | Banovic et al. (2010) | ||
genetic reagent (D. melanogaster) | Nlg1ex2.3 | Banovic et al. (2010) | ||
genetic reagent (D. melanogaster) | Nrx241 | Li et al. (2007) | ||
genetic reagent (D. melanogaster) | Df(3R)BSC747 | Gift from Hermann Aberle | FlyBase: FBab0045813 | |
genetic reagent (D. melanogaster) | Df(3R)Exel6191 | Gift from Hermann Aberle | FlyBase: FBab0038246 | |
genetic reagent (D. melanogaster) | RN2-Flp | Roy et al. (2007) | ||
genetic reagent (D. melanogaster) | UAS-myr::GFP | Pfeiffer et al., 2012 | ||
genetic reagent (D. melanogaster) | UAS-cytoplasmicGFP | Pfeiffer et al., 2012 | ||
genetic reagent (D. melanogaster) | UAS-Lifeact::Ruby | Bloomington Drosophila Stock Centre | BDSC:35545 | |
genetic reagent (D. melanogaster) | UAS-CLIP170::GFP | Stramer et al., 2010 | ||
genetic reagent (D. melanogaster) | UAS-mCD8::ChRFP | Bloomington Drosophila Stock Centre | BDSC:27391 | |
genetic reagent (D. melanogaster) | UAS-myr::tdTomato | Bloomington Drosophila Stock Centre | BDSC:30124 | |
genetic reagent (D. melanogaster) | UAS-BRP::RFP | Gift from Stephan Sigrist | ||
genetic reagent (D. melanogaster) | UAS-Nlg1::GFP | Banovic et al. (2010) | ||
genetic reagent (D. melanogaster) | UAS-Nlg1untagged | Banovic et al. (2010) | ||
genetic reagent (D. melanogaster) | UAS-Nrx::GFP | Banovic et al. (2010) | ||
genetic reagent (D. melanogaster) | UAS-Liprin-α::GFP | Fouquet et al. (2009) | ||
genetic reagent (D. melanogaster) | UAS-Syd1::Straw | Owald et al. (2010) | ||
genetic reagent (D. melanogaster) | UAS-GCaMP6M | Bloomington Drosophila Stock Centre | BDSC: 42750 | |
genetic reagent (D. melanogaster) | UAS-GCaMP6M | Bloomington Drosophila Stock Centre | BDSC: 42748 | |
genetic reagent (D. melanogaster) | GluRIIE::GFP | FlyFos TransgeneOme (fTRG) library | fTRG:154 | |
genetic reagent (D. melanogaster) | BRP::GFP | Bloomington Drosophila Stock Centre | BDSC:59411 | |
genetic reagent (D. melanogaster) | VGlut::GFP | Bloomington Drosophila Stock Centre | BDSC:59292 | |
genetic reagent (D. melanogaster) | Syt1::GFP | Bloomington Drosophila Stock Centre | BDSC:59788 | |
genetic reagent (D. melanogaster) | Nlg1FlpStop | FlpStop construct from Fisher et al. (2017) | ||
genetic reagent (D. melanogaster) | LexAop-myrGFP | Bloomington Drosophila Stock Centre | BDSC:32210 | |
antibody | antiVglut (C-term) (rabbit polyclonal) | Mahr and Aberle (2006) | RRID:AB_2490071; aa 561–632 | (1:10,000) |
antibody | antiBRP (nc82) (mouse monoclonal) | Developmental Studies Hybridoma Bank | RRID:AB_231486; aa 1227–1740 | (1:5) |
antibody | antiGFP (chicken polyclonal) | Thermo Fisher Scientific (Invitrogen) | Cat# PA1-86341, RRID:AB_931091 | (1:1000) |
antibody | antiHRP-Cy3 (goat polyclonal) | Jackson Immunoresearch | (1:5000) | |
antibody | Alexa-488 secondary | Thermo Fisher Scientific (Invitrogen) | (1:500) | |
antibody | Cy3 and Cy5 secondaries | Jackson Immunoresearch | (1:500) |
Additional files
-
Transparent reporting form
- https://doi.org/10.7554/eLife.31659.022