Efficient termination of nuclear lncRNA transcription promotes mitochondrial genome maintenance
Figures
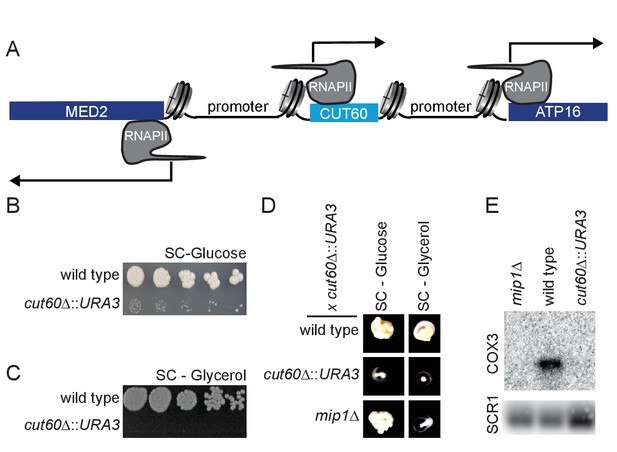
The divergent non-coding transcript CUT60 promotes growth and mitochondrial genome maintenance.
(A) Schematic representation of the bidirectional promoter of MED2. CUT60 is a divergent lncRNA transcript originating from the MED2 promoter, and it is located upstream in tandem of ATP16. (B) Serial dilution growth assay of wild type and cut60Δ::URA3 strains on SC-Glucose medium (n = 3). (C) Serial dilution growth assay of wild type and cut60Δ::URA3 strains on SC-Glycerol medium (n = 3). (D) Growth of wild type, cut60Δ::URA3 and mip1Δ on SC-Glucose and SC-Glycerol after mating with cut60Δ::URA3 strain (n = 3). (E) Northern blot analysis of COX3 and SCR1 (as loading control) transcripts in mip1Δ, wild type and cut60Δ::URA3 (n = 3).
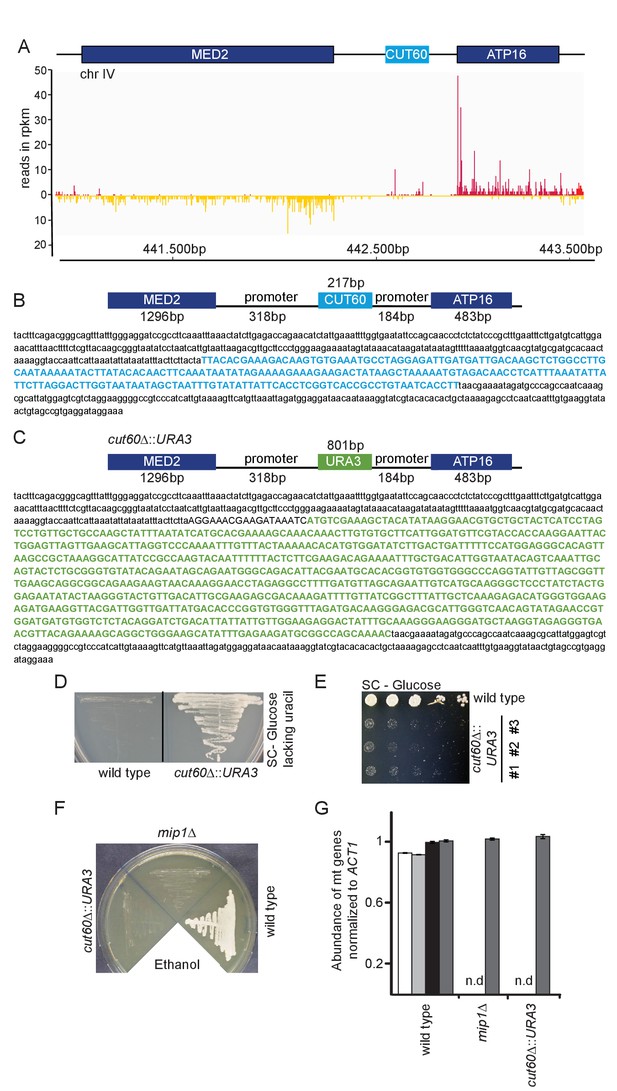
NET-seq data showing low transcription from CUT60 locus compared to MED2 and ATP16 coding regions.
(A) Reads in rpkm shown for a 2.5 kb window of chromosome IV. Schematic representation of MED2, CUT60 and ATP16 transcripts is shown (top). NET-seq reads for the +strand containing CUT60 and ATP16 indicated in red, reads from the – strand containing MED2 indicated in yellow (n = 1). The data were described in (Marquardt et al., 2014). (B) Schematic representation of the locus between MED2 and ATP16. DNA sequence of the individual parts of this locus is shown in the lower panel. ((C)) Schematic representation of the locus between MED2 and ATP16 when the coding sequence of URA3 (green) replaces CUT60 (cut60Δ::URA3). DNA sequence of the individual parts is shown in the lower panel. (D) Left panel shows lack of wild type cell growth, right panel shows growth of cut60Δ::URA3 cells on SC-Glucose media lacking uracil (n = 3). (E) Serial dilution growth assay on SC-Glucose media of wild type, cut60Δ::URA3#1, cut60Δ::URA3#2 and cut60Δ::URA3#3 strains (n = 3). These strains represent independently generated isolates of the cut60Δ::URA3 mutation. (F) Growth of wild type, cut60Δ::URA3, mip1Δ strains on plates with the non-fermentable carbon source ethanol (n = 3). (G) Mitochondrial DNA content in wild type, mip1Δ, cut60Δ::URA3 strains assayed by qPCR. qPCR analysis of three genes encoded by mitochondrial genome COX2 (white), COX3 (grey), ATP8 (black) and nuclear encoded household gene ACT1 (dark grey) in wild type, mip1Δ, cut60Δ::URA3 strains. Gene expression normalized to expression of the nuclear ACT1 transcript. Background level transcript-abundance is indicated by ‘n.d.’ (not detectable). Error bars are s.e.m. of three biological repeats.
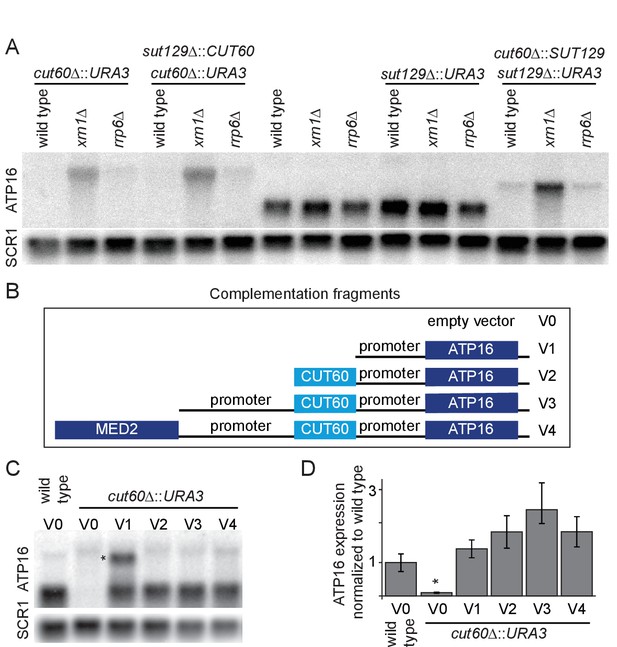
CUT60 promotes ATP16 expression as cis-acting transcript.
(A) Northern blot analysis of ATP16 and SCR1 (as loading control) transcripts in cut60Δ::URA3, sut129Δ::CUT60 + cut60Δ::URA3, wild type, sut129Δ::URA3 and cut60Δ::SUT129 + sut129Δ::URA3 strains. For all strains the transcripts were analyzed in three different backgrounds: Wild type or respective decay pathway mutant background indicated above blot (n = 1). (B) Schematic representation of the five different fragments used for complementation in Figure 2C. V0 is the empty vector control, V1 contains the ATP16 promoter sequence excluding CUT60, V2 expands V1 with CUT60, V3 expands V2 with the MED2 promoter, V4 expands V3 with the sequence of MED2. (C) Northern blot analysis of ATP16 and SCR1 (as loading control) transcript in wild type +empty vector (V0, as shown in Figure 2B), cut60Δ::URA3 with complementation vectors (V0-4) (n = 3). * indicates extended transcript in cut60Δ::URA3 + V1 strain. (D) Quantification of ATP16 expression of Figure 2C. Error bars are s.e.m. of three biological replicates. Statistical significance assessed by t-test, wild type compared to cut60Δ::URA3 is significant (p<0.02). All complementation vectors show no statistical significant increase of ATP16 expression compared to wild type ATP16 expression.
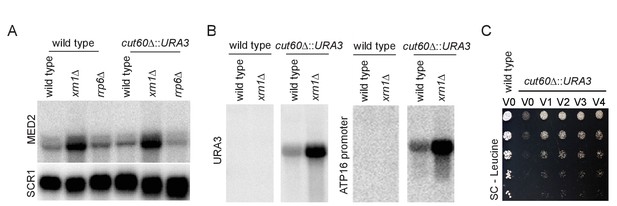
CUT60 influences downstream ATP16 locus in cis.
(A) cut60Δ::URA3 mutants show no detectable effect on MED2 expression. Northern blot analysis of MED2 and SCR1 (as loading control) transcripts in wild type and cut60Δ::URA3 strains. Genetic backgrounds indicated on top (n = 1). (B) Northern blot analysis of URA3 and transcripts derived from expression of the ATP16 promoter region in wild type and cut60Δ::URA3 strains. Genetic backgrounds indicated on top (n = 1). Samples are run on same gel, but the gel was spliced to show the indicated genotypes. (C) Serial dilution growth assay of wild type +V0 and cut60Δ::URA3 with V0-4 on SC-Glucose–Leucine media (constructs shown in Figure 2B) (n = 3).
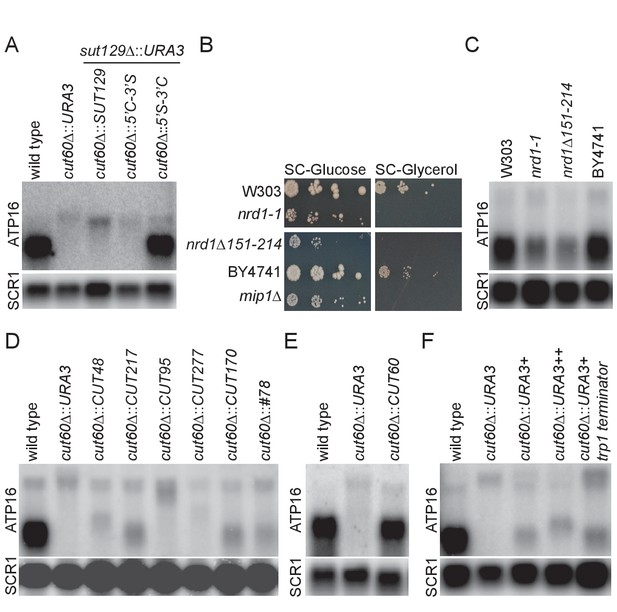
Upstream transcriptional termination promotes ATP16 expression.
(A) Northern blot analysis of ATP16 and SCR1 (as loading control) transcripts in wild type, cut60Δ::URA3, cut60Δ::SUT129 + sut129Δ::URA3, cut60Δ::5’C-3’S + sut129Δ::URA3, cut60Δ::5’S-3’C + sut129Δ::URA3 strains (n = 4). (B) Serial dilution growth of W303 (wild type for nrd1-1), nrd1-1, nrd1Δ151–214 and BY4741 (wild type for nrd1Δ151–214) strains on SC-Glucose and SC-Glycerol plates (n = 2). (C) Northern blot analysis of ATP16 and SCR1 (as loading control) transcripts in W303 (wild type for nrd1-1), nrd1-1, nrd1Δ151–214 and BY4741 (wild type for nrd1Δ151–214) (n = 3). (D) Northern blot analysis of ATP16 and SCR1 (as loading control) transcripts in wild type, cut60Δ::URA3, cut60Δ::CUT48, cut60Δ::CUT217, cut60Δ::CUT95, cut60Δ::CUT277, cut60Δ::CUT170 and cut60Δ::#78 strains (n = 3). (E) Northern blot analysis of ATP16 and SCR1 (as loading control) transcripts in wild type, cut60Δ::URA3 and cut60Δ::CUT60 strains (n = 3). (F) Northern blot analysis of ATP16 and SCR1 (as loading control) transcripts in wild type, cut60Δ::URA3+, cut60Δ::URA3++ and cut60Δ::URA3+trp1 terminator strains (n = 3).
-
Figure 3—source data 1
Table of 68 CUTs with similar genomic configuration to CUT60.
CUT name and genomic coordinates are indicated. Distance and identifier of tandem downstream gene is given. NET-seq data inform on the directionality of the downstream gene promoters. The read-through index calculated from Pol II PAR-CLIP data following Nrd1 depletion is shown. Nrd1 and Nab3 PAR-CLIP data are given normalized to 4sU-seq data to quantify Nrd1 and Nab3 binding.
- https://doi.org/10.7554/eLife.31989.011
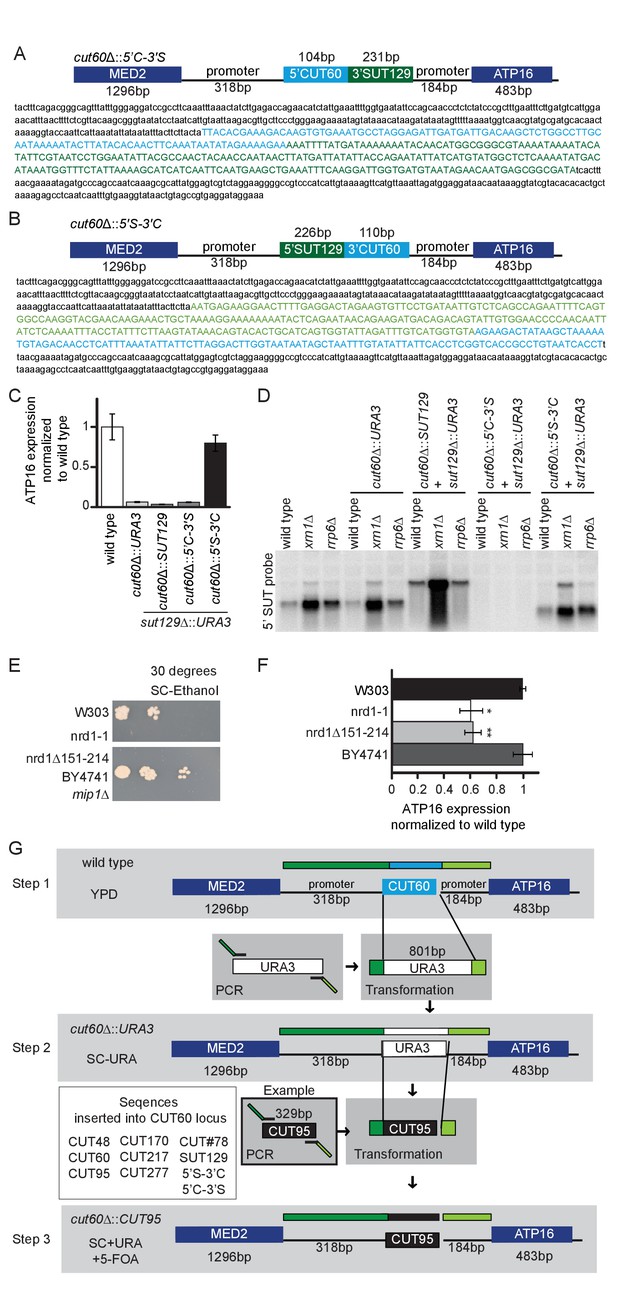
Genomic replacement of CUT60.
(A) Schematic representation of the locus between MED2 and ATP16 when the 5’ sequence of SUT129 is fused to the 3’ sequence of CUT60, and this fusion sequence is inserted into the location of CUT60. The DNA sequences of the individual parts are shown in the lower panel. (B) Schematic representation of the locus between MED2 and ATP16 when the 5’ sequence of CUT60 is fused to the 3’ sequence of SUT129, and this fusion sequence is inserted into the location of CUT60. The DNA sequences of the individual parts of this locus are shown in the lower panel. (C) Quantification of ATP16 expression in northern blot shown in Figure 3A normalized to wild type. Data of expression in wild type, cut60Δ::URA3, cut60Δ::SUT129 + sut129Δ::URA3, cut60Δ::5’C-3’S + sut129Δ::URA3 and cut60Δ::5’S-3’C + sut129Δ::URA3 strains. Error bars are s.e.m. of 4 biological replicates (D) Northern blot analysis with a probe against the 5’-region of SUT129 transcripts in wild type, cut60Δ::URA3, cut60Δ::SUT129 + sut129Δ::URA3, cut60Δ::5’C-3’S + sut129Δ::URA3 and cut60Δ::5’S-3’C + sut129Δ::URA3 strains. For all strains the transcripts were analyzed in three different backgrounds: wild type, rrp6Δ and xrn1Δ indicated on top (n = 1). (E) Serial dilution growth of W303 (wild type for nrd1-1), nrd1-1, nrd1Δ151–214 and BY4741 (wild type for nrd1Δ151–214) strains on SC-Ethanol plates (n = 2). (F) Quantification of ATP16 expression in northern blot shown in Figure 3C. ATP16 expression in W303 (wild type for nrd1-1) and nrd1-1 are normalized to W303. ATP16 expression in BY4741 and nrd1Δ151–214 are normalized to BY4741 (wild type for nrd1Δ151–214). Error bars are s.e.m. of 3 biological replicates. (G) Schematic representation of the three steps needed to generate precise site-specific genomic replacements of CUT60. In step1 wild type yeast needs to be transformed with a PCR product containing the coding sequence of URA3 with overhangs homologous to the sequences upstream and downstream of CUT60. These transformants need to be selected on plates lacking uracil (SC-URA). In step two these transformants need to be transformed with a PCR product containing the sequence of the non-coding sequence for desired CUT60 replacements (CUT95 is shown as example) with overhangs homologous to the sequences upstream and downstream of CUT60. In Step 3, transformants are selected on plates containing 5-FOA and additional uracil (SC + URA + 5 FOA) and confirmed by genotyping.
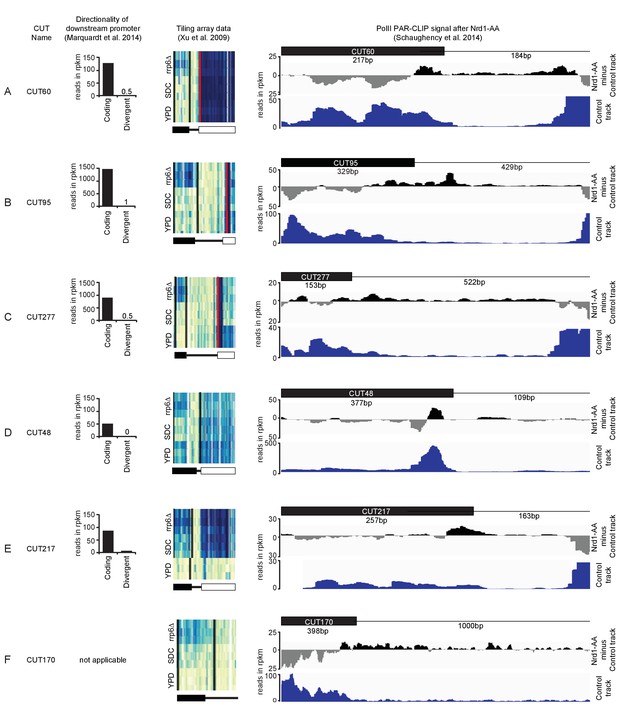
Genomic configuration of CUTs selected for CUT60 replacement.
(A–F) Visualization of three features of CUT60 and CUTs selected for genomic replacement of CUT60. From left to right the name of the CUT, a plot showing the directionality of the promoter of the downstream protein coding gene, a genome browser snapshot of the rrp6Δ, SDC and YPD track described in (Xu et al., 2009) and a genome browser snapshot visualizing differential Pol II PAR-CLIP signal after Nrd1 Anchor-away. The directionality of the downstream protein coding gene is calculated using NET-seq data from (Marquardt et al., 2014) and shown for the coding and the divergent direction of the promoter. Screenshots of the browser described in (Xu et al., 2009) show the level of transcription intensity across the CUTs and the respective downstream sequence by tiling array analysis. The transcription intensity is shown for three replicates in rrp6Δ background, for wild type yeasts grown in SDC medium and wild type yeast grown in YPD medium. Dark blue lines indicate high transcription, white lines indicated low transcription. The schematic underneath the three tracks indicate the starts of the CUTs (black box), the start and end of the downstream promoter (black line) and the start of the downstream gene (white box). The last screenshots visualizing the change of Pol II PAR-CLIP signal upon Nrd1 depletion consist of two tracks: (i) Upper: the difference track where the Pol II PAR-CLIP signal in the negative control (baseline) sample was subtracted from the Nrd1-anchor away (AA) sample. Genomic regions covered by the positive (black) difference values are interpreted as intervals where the transcription read-through is suppressed by Nrd1-dependent termination in the wild type, but becomes visible in Nrd1-AA samples; (ii) Lower: the original baseline (negative control) track to show the overall magnitude of nascent transcription at given region.
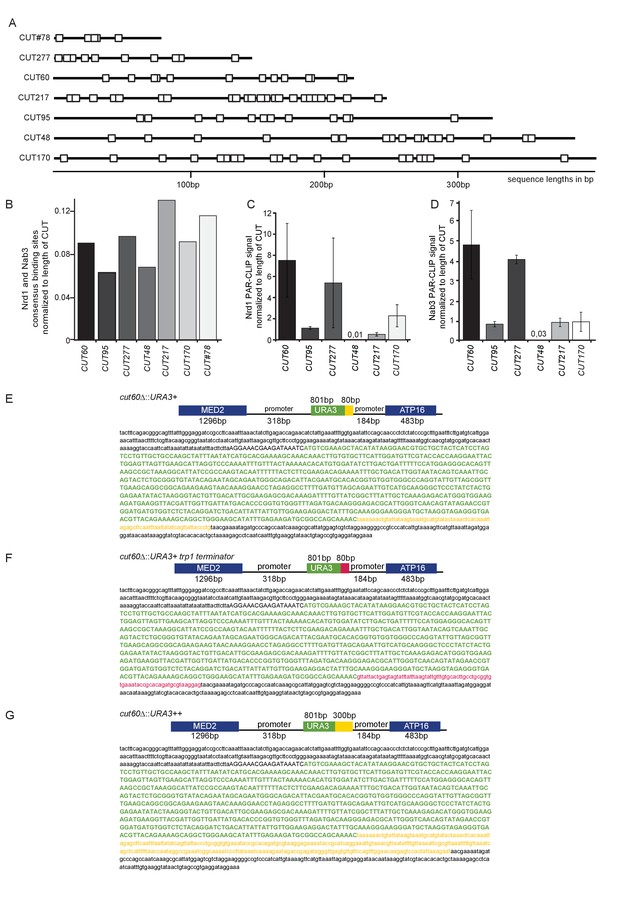
Features preventing Transcriptional Interference highlighted in CUT60 and CUTs selected for genomic replacements.
(A) Schematic representation of all CUTs inserted at the CUT60 locus, their length and their Nrd1p/Nab3p binding sites. Binding sites from (Jamonnak et al., 2011; Carroll et al., 2004; Wlotzka et al., 2011; van Nues et al., 2017; Delan-Forino et al., 2017; Schulz et al., 2013) are shown and indicated as white boxes. (B) Number of consensus Nrd1 and Nab3 binding sites normalized to the length of the individual CUTs are shown for CUT60, CUT95, CUT277, CUT48, CUT217, CUT170 and CUT#78. (C) Nrd1 PAR-CLIP signal (Schulz et al., 2013) normalized to length of the CUTs is shown for CUT60, CUT95, CUT277, CUT48, CUT217 and CUT170 (n = 2). (D) Nab3 PAR-CLIP signal (Schulz et al., 2013) normalized to length of the CUTs is shown for CUT60, CUT95, CUT277, CUT48, CUT217 and CUT170 (n = 2). (E) Schematic representation of the locus between MED2 and ATP16 when the URA3 including an 80 bp endogenous URA3 terminator as present in pRS316 (indicated in yellow) is inserted into the location of CUT60. The DNA sequences of the individual parts of this locus are shown in the lower panel. (F) Schematic representation of the locus between MED2 and ATP16 when the URA3 including an 80 bp terminator derived from the TRP1 locus (indicated in red) is inserted into the location of CUT60. The terminator in this sequence is homologous to the TRP1 terminator found in pRS314. The DNA sequences of the individual parts of this locus are shown in the lower panel. (G) Schematic representation of the locus between MED2 and ATP16 when the URA3 including a 300 bp (indicated in yellow) terminator as present in pRS316 is inserted into the location of CUT60. The DNA sequences of the individual parts of this locus are shown in the lower panel.
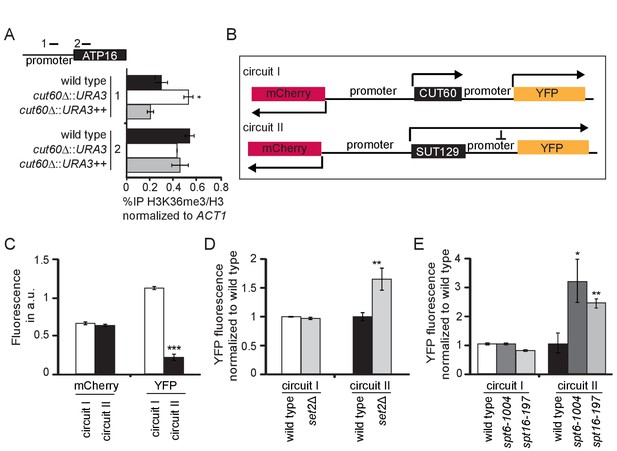
CUT60 prevents ATP16 repression by Transcriptional Interference.
(A) ChIP-qPCR experiments for histone H3K36 trimethylation (H3K36me3) relative to the histone H3 levels at the promoter and gene body of ATP16 in wild type (black), cut60Δ::URA3 (white) and cut60Δ::URA3++ (grey) strains. Error bars are s.e.m. of three biological replicates. * indicates p values of p<0.05 comparing %IP of H3K36/H3 in cut60Δ::URA3 to the %IP of H3K36me3/H3 in wild type. Position of ChIP-qPCR probes indicated in top panel. (B) Schematic representation of synthetic circuits. mCherry replaces MED2, YFP replaces ATP16. Circuit I represents wild type circuit containing CUT60, circuit II represents the cut60Δ::SUT129 mutant circuit. (C) Relative fluorescence of mCherry and YFP in circuit I (white) and circuit II (black). Error bars are s.e.m. of nine biological replicates, the mean was derived from multiple thousand individual measurements. *** indicates p values of p<0.0001. (D) Relative YFP fluorescence of circuit I in wild type (white) and set2Δ (light grey) background, and of circuit II in wild type (black) and set2Δ (light grey) background. YFP fluorescence levels are normalized to the corresponding wild-type values. Error bars are s.e.m. of six biological replicates. ** indicates p values p < 0.01 comparing YFP fluorescence in set2Δ to the respective wild type. (E) Relative YFP fluorescence in circuit I in wild type (white), spt6-1004 (dark grey) and spt16-197 (light grey) background, and in circuit II in wild type (black), spt6-1004 (dark grey) and spt16-197 (light grey) background. YFP fluorescence is normalized to the corresponding wild-type values. Error bars are s.e.m. of six biological replicates. * and ** indicate p values of p<0.05 and p<0.01 respectively comparing YFP fluorescence in spt6-1004 and spt16-194 to the respective wild type.
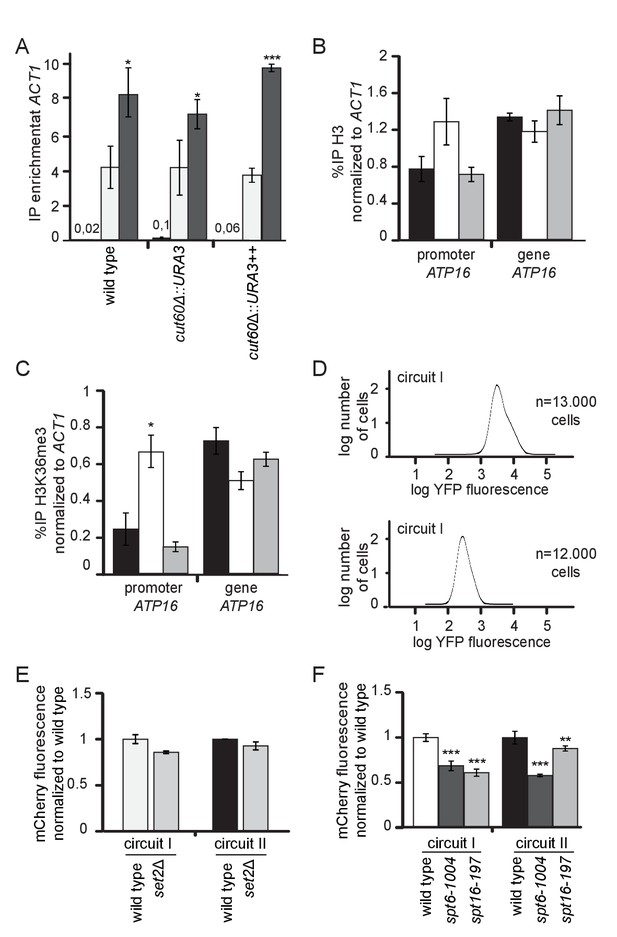
Set2p, Spt6p and Spt16p participate in Transcriptional Interference of ATP16.
(A) Enrichment of DNA corresponding to the Actin control gene for H3 (white) and H3K36me3 (grey) antibodies relative to no antibody control (black, values indicated) in wild type, cut60Δ::URA3 and cut60Δ::URA3 ++ strains. Error bars are s.e.m. of 3 biological replicates. *and *** indicate p values of p<0.05 and p<0.001 comparing H3K36me3 to no antibody control. (B) ChIP-qPCR experiments for histone H3 at the promoter and gene body of ATP16 in wild type (black), cut60Δ::URA3 (white), cut60Δ::URA3++ (grey) strains. Enrichment is normalized to ACT1. Error bars are s.e.m. of three biological replicates. (C) ChIP-qPCR for H3K36me3 levels at the promoter and gene body of ATP16 in wild type (black), cut60Δ::URA3 (white), cut60Δ::URA3++ (grey) strains. Enrichment is normalized to ACT1 locus. Error bars are s.e.m. of three biological replicates. * indicates p values of p<0.05 comparing H3K36me3 at the promoter of ATP16 in cut60Δ::URA3 to wild type. (D) Histograms showing the frequency of YFP fluorescence values of individual cells of cell populations containing circuit I (top panel, n = 13.000 cells) or circuit II (bottom panel, n = 12.000 cells). The distributions suggest variation around one distinct fluorescence state rather than a bi-modal distribution. (E) Relative mCherry fluorescence of circuit I in wild type (white) and set2Δ (light grey) background, and of circuit II in wild type (black) and set2Δ (light grey) background. mCherry fluorescence levels are normalized to the circuit I or circuit II wild type data. Error bars are s.e.m. of six biological replicates. (F) Relative mCherry fluorescence of circuit I in wild type (white), spt6-1004 (dark grey) and spt16-197 (light grey), and of circuit II in wild type (black), spt6-1004 (dark grey) and spt16-197 (light grey). mCherry fluorescence levels are normalized to the circuit I or circuit II wild type data. Error bars are s.e.m. of six biological replicates. ** and *** indicate p values of p<0.01 and respectively p<0.001 comparing mCherry fluorescence in spt6-1004 and spt16-194 to the respective wild types.
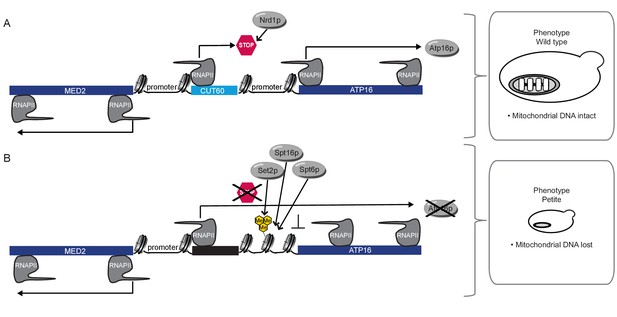
Model for CUT60 function as insulator from Transcriptional Interference.
(A) In the presence of CUT60 transcription initiating from the bidirectional MED2 promoter terminates efficiently with the help of Nrd1p to prevent Transcriptional Interference of ATP16. Atp16p promotes the maintenance of mitochondrial DNA. (B) ATP16 is repressed by Transcriptional Interference if CUT60 is replaced with sequences terminating inefficiently. Interfering transcripts initiate from the bidirectional MED2 promoter. The interference mechanism is enforced by Set2p, Spt6p and Spt16p. This leads to the loss of mitochondrial DNA triggered by the lack of Atp16p.
Tables
Reagent type (species) or resource | Designation | Source or reference | Identifiers | Additional information |
---|---|---|---|---|
strain, strain background (S. cerevisiae) | Yeast Strains | in this study | See Supplementary file 1 Yeast strains | |
strain, strain background (E.coli) | E. coli | Thermo Fisher | Cat#18265017 | E. coli DH5α Competent Cells |
antibody | H3 | Abcam | Ab1791; RRID: AB_302613 | Anti-Histone H3 |
antibody | H3K36me3 | Abcam | Ab9050; RRID: AB_306966 | Anti-Histone H3 tri methyl K36 |
commercial assay or kit | QIAprep Spin Miniprep Kit | QIAGEN | Cat#27106 | |
commercial assay or kit | Wizard SV Gel and PCR Clean-Up System | Promega | Cat#A9182 | |
commercial assay or kit | MasterPure Yeast RNA Purification Kit | Lucigen | MPY03100 | |
chemical compound, drug | Protein A magnetic beads | GenScript | Cat. No. L00273 | |
chemical compound, drug | Phusion high-fidelity PCR master mix | NEB | cat. no. M0531S | |
chemical compound, drug | GoTaq qPCR Master Mix | Promega | Cat#A6002 | |
chemical compound, drug | NAT | Jena Bioscience | AB-102L | Nourseothricin dihydrogen, cloNAT |
chemical compound, drug | G418 | Duchefa-biochemie | G0175 | G-418 disulphate |
chemical compound, drug | 5FOA | Thermo Fisher | R0811 | 5-Fluoroorotic Acid |
software, algorithm | ImageQuant | GE Healthcare | NA | |
software, algorithm | Software for flow cytometry and bioinformatic analysis: see Materials and methods | NA | NA | |
other | Oligonucleotides | in this study | NA | See Supplementary file 2 Oligonucleotides |
other | Equipment: BD Fortessa | BD biosciences | NA | |
other | Plasmids | in this study | NA | See Supplementary file 3 Plasmids |
Additional files
-
Supplementary file 1
Table S1 Yeast strains.
Names, genotypes, purposes and origins of yeast strains used in the manuscript are indicated.
- https://doi.org/10.7554/eLife.31989.015
-
Supplementary file 2
Table S2 Oligonucleotides.
Names, DNA sequences and purposes of oligonucleotides used in the manuscript are indicated.
- https://doi.org/10.7554/eLife.31989.016
-
Supplementary file 3
Table S3 Plasmids.
Names, identifiers and purposes of plasmids used in the manuscript are indicated.
- https://doi.org/10.7554/eLife.31989.017