A subcortical circuit linking the cerebellum to the basal ganglia engaged in vocal learning
Figures
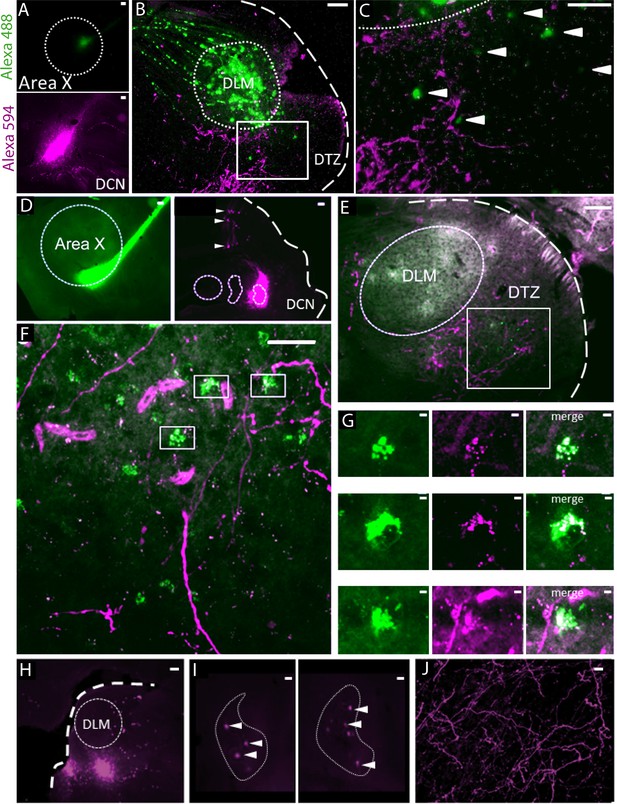
Anatomical connection between DCN and Area X.
(A) Injection sites of Dextran Alexa 488 (green, top panel) and Dextran Alexa 594 (magenta, bottom panel, sagittal sections). Dotted line delimits Area X (top panel). Scale bar: 100 µm. (B) Labelling in the dorsal thalamic zone (DTZ) showing efferent cerebellar fibers (magenta) and cell bodies of neurons in DTZ (green). Large labelling of efferent fibers from Area X nucleus was also found in DLM, as the tracer is anterogradely and retrogradely transported. The dotted line in B delimits nucleus DLM, while the white square indicates magnification location for C. The large dotted line delimits the brain slice contour. Scale bar: 100 µm. DLM: dorsolateral nucleus of the anterior thalamus. (C) Magnification of the dorsal thalamic zone. Cerebellar fibers are labelled in magenta, and somata are labelled in green and indicated with arrowheads. Scale bar: 100 µm. (D) Injection sites of cholera toxin B in Area X (green, left panel) and Dextran Alexa 594 in DCN (magenta, right panel). Dotted lines delimit Area X (left panel) and all three DCN (right panel). The large dotted line delimits the brain slice contour. Retrograde labeling of Purkinje cells projecting to the DCN targeted by dye injection can be observed (right panel, arrowheads). Scale bar: 100 µm. (E–F): Close contacts were observed in the dorsal thalamic zone (DTZ, scale bars: 100 µm). The dotted line in E delimits nucleus DLM, while the white square in E and F indicates magnification location. Efferent fibers from Area X in DLM appear as diffuse green labeling in this nucleus, while green cell somas in DTZ reflect afferent neurons. Magenta-labeled fibers from the DCN surround Area X-projecting neurons in DTZ. (G): Three examples of close contacts between fibers from the DCN (magenta, middle panel) and soma of neurons projecting to Area X (green, left panel) in DTZ. Each panel in G corresponds to a magnification of squares indicated in F. The merge suggests an anatomical connection (right panel). Scale bar: 2 µm. (G) Injection sites of Dextran Alexa 594 in DTZ. The large dotted line delimits slice contours, and the dotted circle represents DLM. Scale bar: 100 µm. (H) Two examples of retrograde labelling in the lateral DCN following DTZ injection showed in G. Both examples are from the same animal, at two different depths. (I) Arrowheads indicate DCN cell soma labelled. The dotted line delimits the lateral DCN contours. Scale bar: 20 µm (J) Example of anterograde labelling in Area X. Only fibers (but no soma) were observed in Area X after DTZ injection. Scale bar: 2 µm.
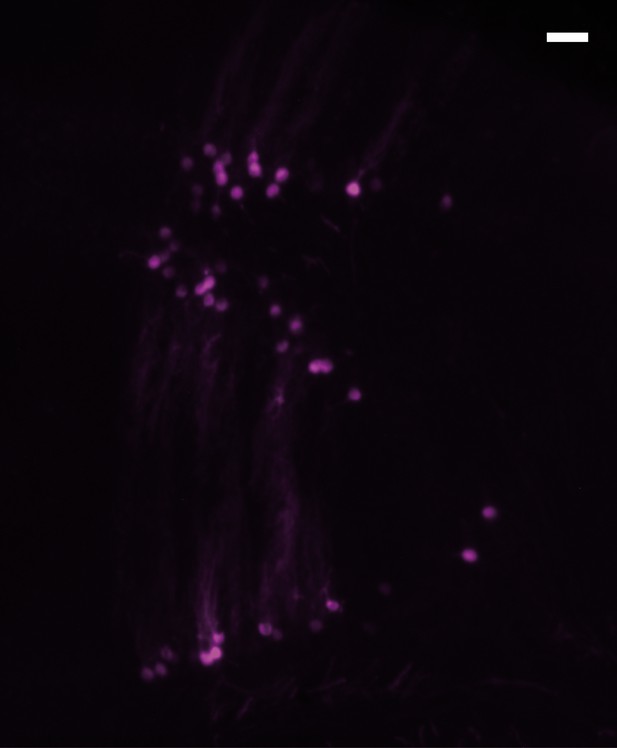
Magnification of Purkinje cell labelling.
Magnification of the Purkinje cell labelling indicated by arrowheads in Figure 1D. This labelling is due to the retrograde transport of Dextran-Alexa 596 after DCN injection. Scale: 50 µm.
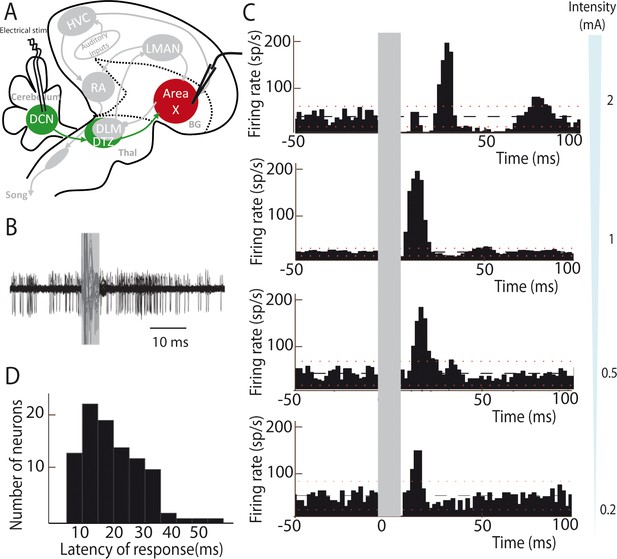
Deep cerebellar stimulation elicits strong excitation in pallidal cells of Area X.
(A) Diagram of the song system in songbirds. In all song system diagrams in Figures 2–6, we highlight nuclei involved in the experiment reported on a given figure in color, while other song system nuclei are in grey shades. The colors used are different for each pathway: red for the song-related basal ganglia-thalamo-cortical circuit composed of the basal ganglia nucleus Area X, the thalamic nucleus DLM, and the cortical nucleus LMAN, green for the cerebello-thalamo-basal ganglia circuit through the DCN and DTZ, and black for the motor pathway composed of HVC and RA. Here, stimulations were performed in the DCN during the recording of pallidal neurons in Area X. HVC: used as a proper name, RA: robust nucleus of the archopallium, LMAN: lateral magnocellular nucleus of the anterior nidopallium, DLM: medial portion of the dorsolateral nucleus of the anterior thalamus, DTZ: dorsal thalamic zone, DCN: deep cerebellar nuclei. (B) Twenty superimposed extracellular recording traces around DCN stimulation show the increase in the number of spikes produced by a representative pallidal neuron following DCN stimulation (grey rectangle) compared to baseline firing. (C) Peri-stimulus-time-histograms (PSTHs) representing the firing rate of 4 different pallidal neurons around DCN stimulation (time bin: 2 ms). The black horizontal dashed line depicts the mean baseline firing rate and red dotted lines indicate confidence intervals (2.5 SD away from the mean baseline firing rate). Different response profiles are shown: excitation only (the two in the bottom, stimulation at 0.2 and 0.5 mA), biphasic response (second PSTH from top, stimulation at 1 mA), or inhibition and biphasic response (top, stimulation at 2 mA). (D) Distribution of response latencies between DCN stimulation and the beginning of the excitatory response (20.80 ± 4.56 ms, median: 21 ms).
-
Figure 2—source data 1
Peri-stimulus time histogram (PSTH) code.
This code source was used to build PSTHs in Figures 2, 4, 5, 6 and 7. Spike train analysis was then performed using Matlab (MathWorks, Natick, MA, USA). We calculated peri-stimulus time histograms (PSTH) of recorded neurons after stimulation. PSTHs were calculated with a 2 ms bin for neurons, or 10 ms to limit bin-to-bin fluctuations in spike count in structures with low firing rate. This code calculate the mean and the standard deviation (SD) of the firing rate over the period preceding the stimulation and the program considered that a neuron exhibited a significant response to the stimulation when at least two consecutive bins of the PSTH were above (for excitation) or below (for inhibition) the spontaneous mean firing rate ±2.5*SD. The return of two consecutive bins at the spontaneous mean firing rate ±2.5*SD indicated the end of the response.
- https://doi.org/10.7554/eLife.32167.006
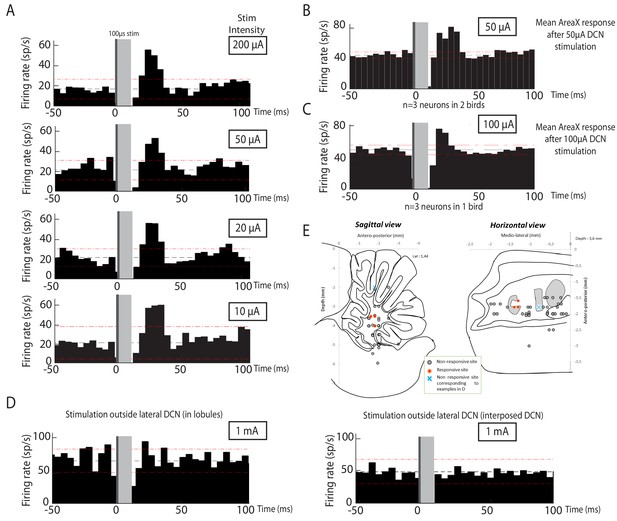
Area X neurons selectively respond to electrical stimulation of the lateral deep cerebellar nucleus (DCN), even at low intensity.
Following low-intensity (from 50 to 200µA) stimulation of the lateral DCN through short-duration (100 µs) electrical pulses induces responses in pallidal-like neurons of the song-related basal ganglia nucleus Area X. (A) Response to DCN stimulation at various current intensities ranging from 50 to 200 µA in a typical pallidal-like neuron from Area X (100 µs monophasic pulses, represented by dark grey rectangles, while light grey rectangles indicate the 'blind' period due to stimulation artifact). With each stimulation intensity, we observe an excitation of pallidal-like neurons following stimulation in the lateral DCN. The black dotted line represents the mean frequency of the pallidal-like neuron during the baseline period, and the red dotted line corresponds to 2.5*standard deviation from baseline. Excitation/inhibition is significant when two consecutive columns are upper/lower than red lines. Time bin: 5 ms. (B) 50µA DCN stimulation induces an increased firing rate in pallidal-like neurons. In n=3 neurons recorded in two different birds, we observed a similar increase in activity following 50 µA stimulation (100 µs monophasic pulses, time bin: 5 ms). (C) 100µA DCN stimulation induces an increased firing rate in pallidal-like neurons. In n=3 neurons recorded in two different birds, we observed a similar increase in activity following 100 µA stimulation. (100 µs monophasic pulses, time bin: 5 ms). (D) Area X pallidal-like neurons do not respond to electrical stimulation outside the lateral DCN. The pallidal-like neurons did not exhibit significant inhibition or excitation when the electrical stimulation was applied away from the lateral DCN even at high intensity (1 mA, 1 ms-long monophasic pulses), neither in the cortex (left panel, coordinates indicated in E by a cross) nor in another DCN (stimulation in interposed nucleus, right panel, coordinates indicated in E by a cross). (E) Responses in Area X pallidal-like neurons were limited to stimulation points located in the lateral DCN. Coordinates used to place the stimulation electrode were summed up on two different schemes corresponding to sagittal (left) or horizontal (right) views. Only a few points (n=3) of stimulation induced responses in pallidal-like neurons (red point). Stimulations at other coordinates (black point, n =32 points) did not induce any response. Blue crosses correspond to the two examples showed in D.
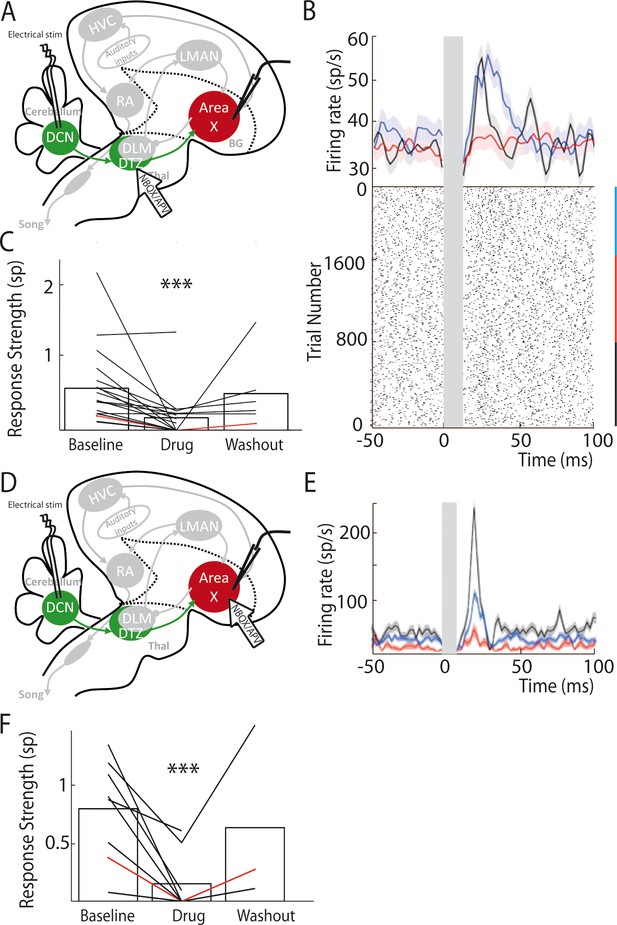
Area X pallidal responses to DCN stimulation are transmitted through excitatory synapses in DTZ and Area X.
(A) Diagram of the song system in songbirds, as in Figure 2A. Recordings were performed in Area X, NBQX/APV were applied in DTZ. (B) PSTH (top part) of a typical pallidal neuron before (black), during (red) and after (blue, washout) drug application in DTZ, and their corresponding raster plots (bottom part). (C) Population data showing the response strength of pallidal neurons in the three conditions (baseline, drug and washout, n = 16 pallidal neurons in 8 birds, paired Wilcoxon test, p value<0.001). The red line represents the example shown in B. (D) Diagram of the song system, as in Figure 2A. Recordings were performed in Area X, NBQX/APV were applied in Area X in proximity to the recorded neuron. (E) PSTH representing the firing rate of one pallidal neuron, before (black), during (red) and after (blue, washout) drug application in Area X. Baseline activity after drug application (red) sometimes slightly decreases in Area X neurons compared to before drug application (black), but no significant change was observed over all neurons recorded in this condition (see Results). (F) Population data showing the evolution of response strength before, during and after drug application (n = 8 pallidal neurons in 7 birds, paired Wilcoxon test, p value<0.001). The red curve represents the example shown in E. In this figure and the following ones, stars indicate significance level (*p<0.05; **p<0.01; ***p<0.001).
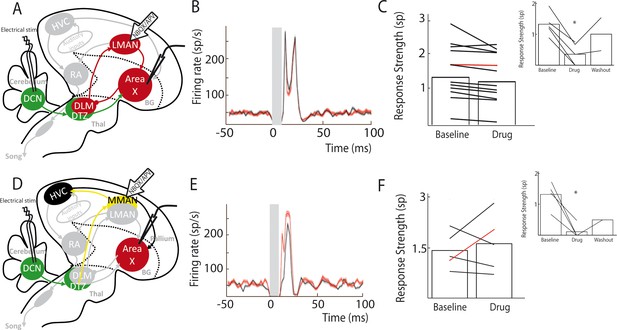
Area X pallidal responses to DCN stimulation are not transmitted through the cortical nuclei LMAN or MMAN.
(A) Diagram of the song system, as in Figure 2A. Recordings were performed in Area X, NBQX/APV were applied in LMAN. (B) PSTH representing the firing rate of a pallidal neuron around DCN stimulation before (black) and during (red) drug application in LMAN. (C) Population data showing no change in response strength before and during LMAN glutamatergic blockade (n = 12 pallidal neurons in 6 birds, paired Wilcoxon test, non-significant). The red curve represents the example shown in B. Inset: confirmation of drugs efficiency by applying drugs around the recorded pallidal neuron (n = 5 pallidal neurons in 5 birds, paired Wilcoxon test, p<0.01). (D) Diagram of the song system. Recordings were performed in Area X, NBQX/APV were applied in MMAN, a nucleus projecting to HVC. (E) PSTH representing the firing rate of pallidal neuron before (black) and during (red) drug application in MMAN. (F) Population data showing the evolution of response strength before and during glutamatergic blockade in MMAN (n = 5 pallidal neurons in 2 birds, paired Wilcoxon test, non-significant). The red curve represents the example shown in E. Inset: confirmation of drug efficiency by applying drug on the recorded pallidal neuron (n = 5 pallidal neurons in 2 birds, paired Wilcoxon test, p<0.05).
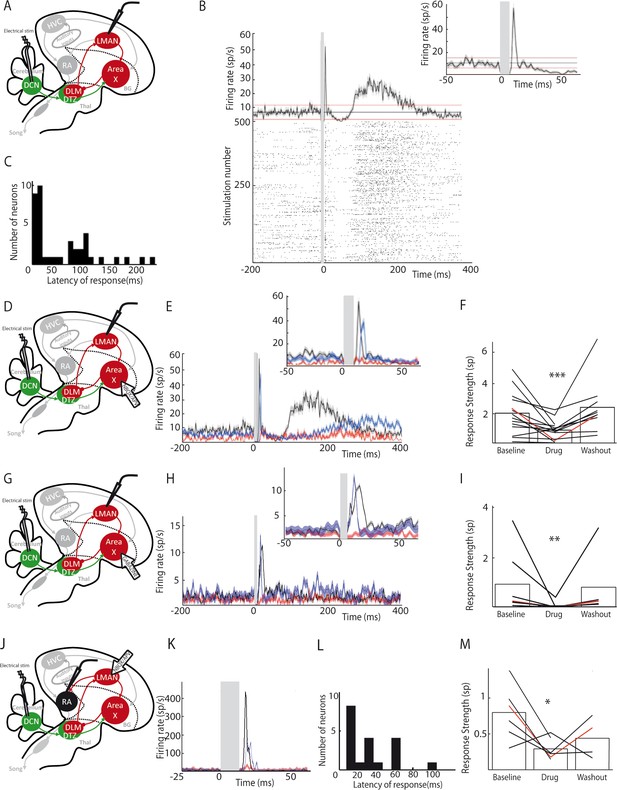
LMAN and RA neurons display responses to DCN stimulation.
(A) Diagram of the song system, as in Figure 2A. Neuronal activity was recorded in LMAN during DCN stimulation (B) Example response in a LMAN multi-unit recording displaying two excitatory peaks following DCN stimulation with the corresponding raster plot. Inset: magnification of the first excitatory peak. (C) Distribution of response latency over all LMAN recordings displaying the two characteristic peaks of response (first peak: 10–30 ms and second peak: 100 ms, see Results, time bin: 10 ms). (D) Diagram of the song system. NBQX/APV were applied in Area X and neuronal activity was recorded in LMAN during DCN stimulation. (E) Example response following DCN stimulation from a multi-unit recording in LMAN, before (black), during (red) and after (blue, washout) the drug application. (F) Population data showing the evolution of response strength over the three periods (baseline, drug, washout, n = 14 multiunit recording sites in 5 birds, paired-Wilcoxon test, p value=0.001). The red curve represents the example shown in E. (G) Diagram of the song system. Gabazine was applied in Area X and neurons were recorded in LMAN during DCN stimulation. (H) Example response from a multi-unit recording in LMAN following DCN stimulation, before (black), during (red) and after (blue, washout) gabazine application. (I) Population data showing the evolution of the response strength over the three periods (baseline, drug, washout, n = 7 multiunit recording sites in 4 birds, paired-Wilcoxon test, p value=0.0156). The red curve represents the example shown in H. (J) Diagram of the song system, as in Figure 2A. Neurons were recorded in RA during DCN stimulation, NBQX/APV were applied in LMAN. (K) PSTH representing the firing rate of a typical RA neuron before (black), during (red) and after (washout, blue). (L) Distribution of RA neurons response latencies (time bin: 10 ms). (M) Population data showing the change of response strength over the three periods (baseline, drug, washout, n = 6 neurons in 5 birds, paired Wilcoxon test, p<0.05). The red curve represents the example shown in C.
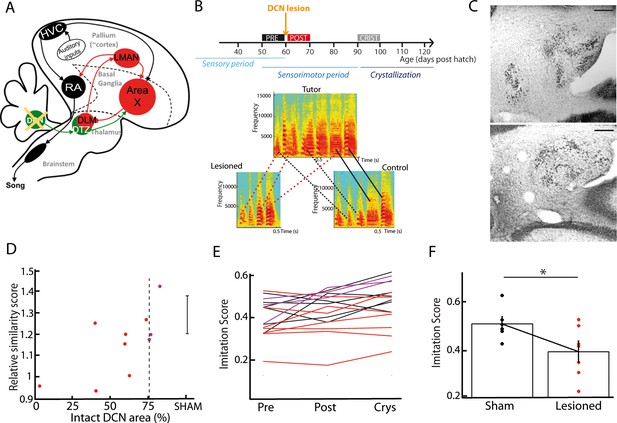
DCN lesions impair song learning and reduce the similarity to tutor song after crystallization.
(A) Diagram of the song system, as in Figure 2A, representing DCN lesion. (B) Top: Diagram of the song learning periods in songbirds: the sensory period, the sensorimotor period in which juveniles start to produce sounds, and the crystallization phase. Lesions were made at 60 dph. Bottom: Examples of three spectrograms of tutor and pupil song motifs at crystallization: top: tutor, bottom left: a pupil with DCN lesion, bottom right: control pupil. Solid lines connect two similar syllables found in the tutor and juvenile song motifs, dotted lines between two syllables reflect a partial copy of the tutor syllable (red lines for the juvenile with DCN lesion, black lines for the control juvenile). (C) Nissl staining on horizontal slices showing the deep cerebellar nuclei. Top: control bird. Bottom: bird with DCN lesion (Scale bar: 100 µm). (D) Normalized imitations score (imitation score at crystallization divided by the imitation score before surgery) plotted as a function of the total area left from the lateral DCN (%) for juveniles with DCN lesion (red and purple dots, n = 10 birds). The mean and SD of the normalized imitation score over the sham group are represented as an error bar (n = 6 sham birds). For the following analysis, we consider the birds with a significant lesion (<75% of intact lateral DCN, vertical dotted line) represented with red dots. A significant correlation was revealed between the similarity and the proportion of lateral DCN left intact (r = 0.68, p<0.05). (E) Population data showing the evolution of the imitation score between the day before the lesion (pre) and the crystallization period (90 dph) in juveniles with sham lesions (black lines for individual birds) and DCN lesions with larger lesion size (red lines) or small lesion size (purple lines). (F) The imitation score at crystallization is significantly larger in the sham group than in the DCN large lesion group (n = 7 birds with large lesion, n = 6 sham birds, Wilcoxon test, p<0.05).
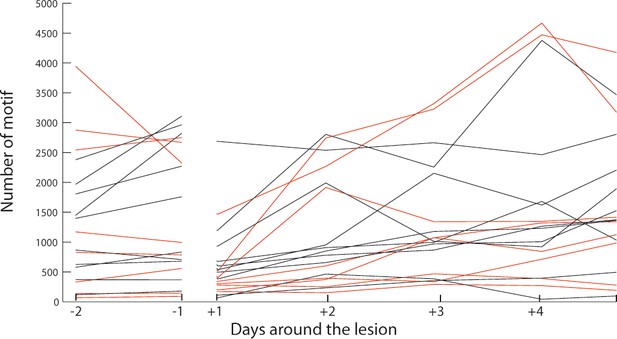
Song rate is not affected by DCN lesion.
Representation of the song rate in the sham (black) and lesion (red) groups around the day of surgery. Each bird is represented with a single line. Day +1 corresponds to the first day with song production following surgery. Quantification of the singing rate among the two groups did not reveal any significant difference between the sham and lesion groups (n = 10 birds in sham group, n = 8 birds in lesioned group, Wilcoxon test, p=0.24). Moreover, all birds resumed singing in the 1–4 days following surgery and there was no difference in the time delay between surgery and the first day with song in the two groups (n = 10 birds in sham group, n = 8 birds in lesioned group, Wilcoxon test, p=0.5).
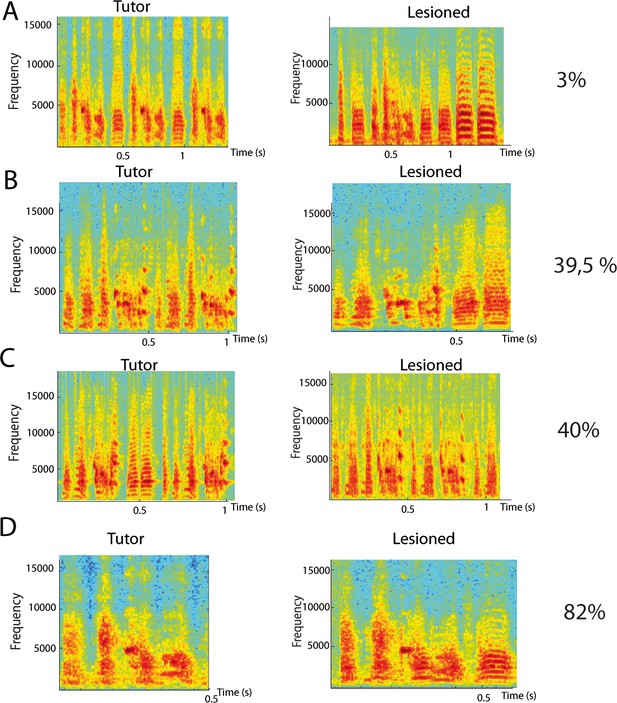
Example spectrogram of tutor and pupil (control and lesion) song motifs as a function of lesion size.
Pupil received DCN partial lesions at 60 dph. (A–D) Example spectrograms of the tutor song motif (left) and the song motif at crystallization of its pupil with a cerebellar lesion (right), in four different families. Histological control indicates that the proportion of intact lateral DCN respectively covered around 3, 40, 39.5, and 18% of the lateral DCN volume in the pupils shown in (A, B, C and D). Note that the three first examples represent large DCN lesions (>50% lateral affected), while the last example is from a bird with a small DCN lesion.
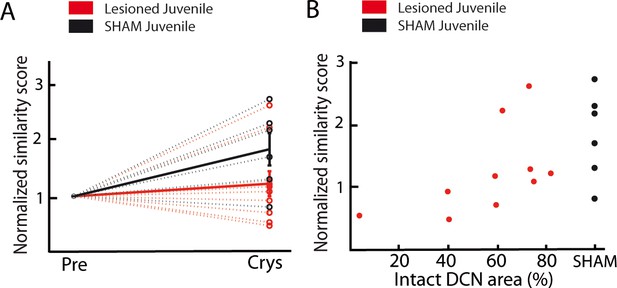
Effect of DCN lesions revealed by a custom-written similarity score based on the peak cross-correlation between the spectrograms of the tutor’s motifs and of the pupil’s songs.
Among all songs produced by the pupil in each considered condition: before lesion or at crystallization (all recordings from a single day of recording were considered for analysis in each condition: pre-surgery or after crystallization), 10 randomly-selected songs were compared to the tutor’s selected motifs using the following procedure. Cross-correlations of the spectrograms were computed between all possible pairs defined as follows: a pair consisted in a tutor’s motif and a pupil’s song. For each pair, a cross-correlation index was calculated as the sum of the cross-correlation function between their two spectrograms, normalized by the square root of the product of their auto-correlation function. The average cross-correlation index over all 100 pairs was called the ‘spectral similarity index’ between tutor and juvenile in that condition. (A) Population data showing the evolution of similarity between the day before the lesion (pre) and the crystallization period (90 dph) in pupils with sham lesions (black dots for individuals, solid black line for the mean) and DCN lesions (red dots for individual, solid red line for the mean). Data are normalized over the pre-lesion period. (B) Normalized similarity score plotted as a function of the total area left from the lateral DCN (%) for juveniles with DCN lesion (red dots, n = 10 birds) or sham lesion (black dots, n = 6 birds). A significant correlation was revealed between the quality of the tutor song imitation and the proportion of lateral DCN left unaffected (Pearson correlation coefficient, r = 0.57, p<0.05).
-
Figure 7—figure supplement 3—source data 1
Source code for similarity score analysis.
Among all songs produced by the pupil in each considered condition: before lesion or at crystallization (all recordings from a single day of recording were considered for analysis in each condition: pre-surgery or after crystallization), 10 randomly-selected songs were compared to the tutor’s selected motifs using the following procedure. Cross-correlations of the spectrograms were computed between all possible pairs defined as follows: a pair consisted in a tutor’s motif and a pupil’s song. For each pair, a cross-correlation index was calculated as the sum of the cross-correlation function between their two spectrograms, normalized by the square root of the product of their auto-correlation function. The average cross-correlation index over all 100 pairs was called the ‘spectral similarity index’ between tutor and juvenile in that condition.
- https://doi.org/10.7554/eLife.32167.015
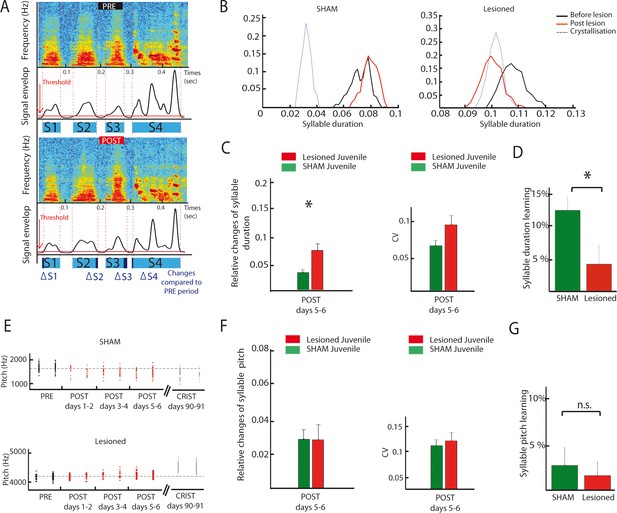
DCN lesions effects on syllable duration and fundamental frequency in juvenile zebrafinches.
(A) Representation of the protocol of syllable duration calculation. The envelop signal of the song was determined, and a threshold was set to determine the beginning and the end of each syllable. (B) Distribution of the duration of a syllable over several days in the sham group (left panel) and in the lesion group (right panel, post lesion period in red, crystallization period in grey). (C) The duration of syllables before and after the lesion were compared based on their relative changes between these two periods. Left panel: Changes in syllable duration relative to baseline in the sham group (green) and the lesion group (red) for juvenile birds one week after cerebellar lesion. Right panel: CV of the duration of syllables in the sham (green) and lesion (red) juvenile groups one week after cerebellar lesion. (D) Learning trajectory for the duration of syllables in the sham (green) and lesion (red) juvenile groups. The learning trajectory is determined by the difference between the relative changes in duration at the crystallization phase (days 90–91) and relative changes in duration during the post days 5–6 after lesion. Learning-related changes in duration in the lesion group significantly differ from those in the sham group (n = 21 syllables in lesion group, mean: 4 ± 2.7%, n = 24 syllables in sham group, mean: 12,1 ± 1,9%, Wilcoxon test, p=0.016). (E) Distribution of the fundamental frequency of example harmonic stacks from the sham group (top panel) or the lesion group (bottom panel) over several days (post lesion period in red, crystallization period in grey). (F) Left panel: Changes in fundamental frequency relative to baseline for harmonic stacks in the sham (green) and lesion (red) groups. Right panel: CV of the fundamental frequency of harmonic stacks in sham (green) and lesion (red) groups. No difference was observed between different conditions for the fundamental frequency analysis, p>0.05. (G) Learning trajectory for the fundamental frequency of harmonic stacks in the sham (green) and lesion (red) groups. The learning trajectories for fundamental frequency were similar in both groups (sham group, n = 10 harmonic stacks, mean: 2.7 ± 1.9%, lesion group, n = 19 harmonic stacks, mean: 1.6 ± 1.5%, Wilcoxon test, p=0.50).
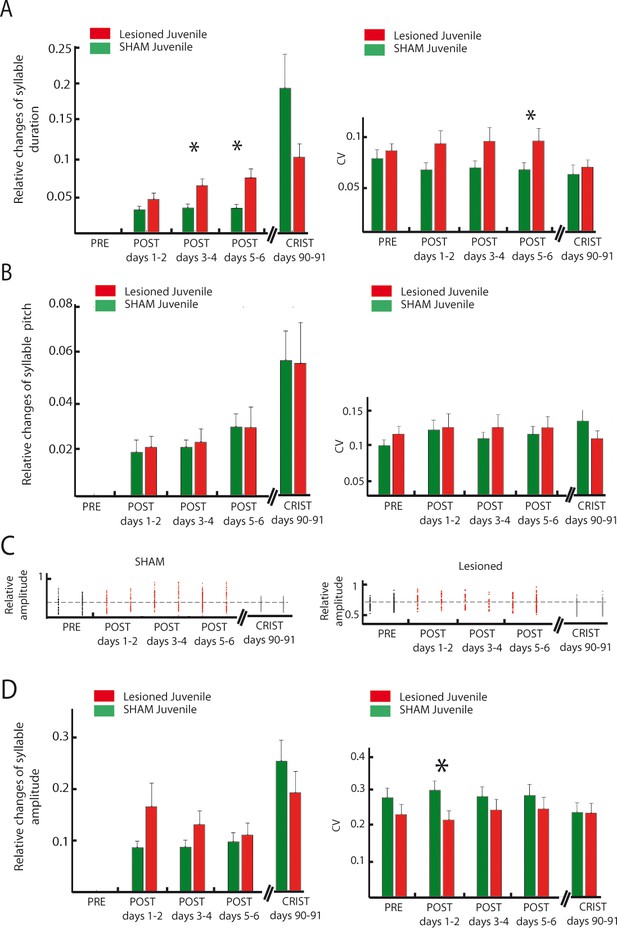
Effect of cerebellar lesions on the time course of syllable duration, fundamental frequency and amplitude in juvenile birds.
(A) Left panel: Changes in syllable duration relative to baseline in the sham (green) and lesion (red) juvenile groups for each consecutive two-day period following cerebellar lesion. Duration changes are significantly different between sham and lesion group for post 5–6 (Wilcoxon test with Bonferroni correction for multiple tests, post days 1–2, p=0.56, post days 3–4, p=0.03, post days 5–6, p=0.003, crystallization days 90–91, p=0.1). Right panel: variability of syllable duration in the sham (green) and lesion (red) juvenile groups for each consecutive two-day period following cerebellar lesion. No difference in the CV of syllable duration was observed for all time periods (Wilcoxon test with Bonferroni correction for multiple tests, pre, p=0.21, post days 1–2, p=0.03, post days 3–4, p=0.06, post days 5–6, p=0.03, crystallization days 90–91, p=0.23). (B) Left panel: Changes in fundamental frequency relative to baseline in the sham (green) and lesion (red) juvenile groups for each consecutive two-day period following cerebellar lesion. No difference in the mean fundamental frequency was observed for all time periods (Wilcoxon test with Bonferroni correction for multiple tests, pre, p=0.4, post days 1–2, p=0.6, post days 3–4, p=0.4, post days 5–6, p=0.3, crystallization days 90–91, p=0.23). Right panel: variability of fundamental frequency in the sham (green) and lesion (red) juvenile groups for each consecutive two-day period following cerebellar lesion. No difference in the CV was observed for all time periods (Wilcoxon test with Bonferroni correction for multiple tests, pre, p=0.2, post days 1–2, p=0.8, post days 3–4, p=0.8, post days 5–6, p=0.6, crystallization days 90–91, p=0.5). (C) Distribution of relative amplitude over several days for an example syllable in the sham (left panel) and lesion juvenile groups (right panel, post lesion period in red, crystallization period in grey). (D) Left: Changes in amplitude relative to baseline in the sham (green) and lesion (red) juvenile groups. No difference was found between the two groups (Wilcoxon test with Bonferroni correction for multiple tests, post days 1–2, p=0.3 post days 3–4, p=0.28, post days 5–6, p=0.9, crystallization days 90–91, p=0.45). Right: CV of relative amplitude in the sham (green) and lesion (red) juvenile groups. No difference was observed for all time periods (Wilcoxon test with Bonferroni correction for multiple tests, pre, p=0.3, post days 1–2, p=0.04, post days 3–4, p=0.6, post days 5–6, p=0.7, crystallization days 90–91, p=0.8).
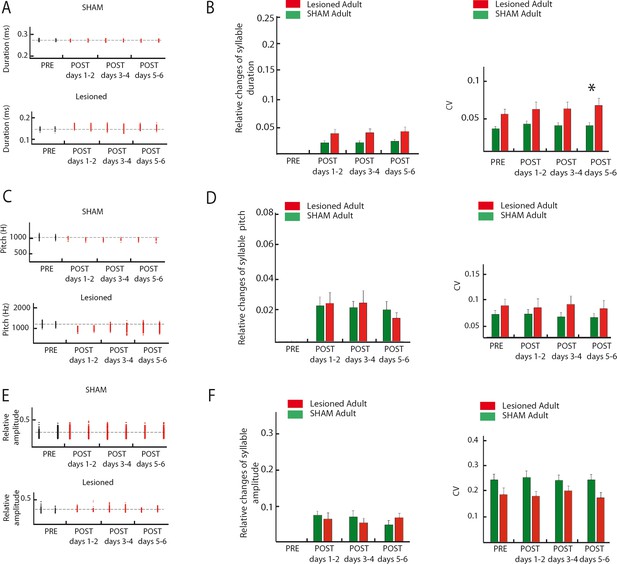
Cerebellar lesions acutely impact syllable duration but do not affect fundamental frequency and amplitude in adult zebra finch song.
(A) Distribution of the duration of an example syllable over several days in the sham (top panel) and lesion (bottom panel) groups for adult birds (period before lesion in black, post lesion period in red). (B) Changes in syllable duration relative to baseline (left panel) in the sham (green, n = 26 syllables in 6 birds) and lesion (red, n = 25 syllables in 5 birds) groups. For all post periods, changes in syllable duration between the two groups were not significantly different (post days 1–2, p=0.05; post days 3–4, p=0.08; post days 5–6, p=0.3, Wilcoxon test with Bonferroni correction for multiple tests). Right panel: variability of syllable duration in sham (green) and lesion (red) groups. The CV of syllable duration was not significantly different between the two groups over all time periods (pre, p=0.06, post days 1–2, p=0.3; post days 3–4, p=0.2; post days 5–6, p=0.04, Wilcoxon test with Bonferroni correction for multiple tests). (C) Distribution of the fundamental frequency of an example harmonic stack over several days in the sham (top panel) and lesion (bottom panel) groups (post lesion period in red). (D) Left panel: Changes in fundamental frequency relative to baseline in the sham (green, n = 16 harmonic stacks in 6 birds) and lesion (red, n = 16 harmonic stacks in 5 birds) groups. No difference was observed for the three periods after lesion (Wilcoxon test, post days 1–2, p=0.9, post days 3–4, p=0.6, post days 5–6, p=0.8). Right panel: CV of the fundamental frequency in the sham (green) and lesion (red) groups. No difference in the variability of fundamental frequency was observed for the four periods around lesion (Wilcoxon test, pre, p=0.7, post days 1–2, p=0.4, post days 3–4, p=0.8, post days 5–6, p=0.9). (E) Distribution of the amplitude of an example syllable over several days in the sham (top panel) and lesion (bottom panel) groups (post lesion period in red). (F) Top: Changes in syllable amplitude relative to baseline in the sham (green, n = 26 syllables in 6 birds) and lesion (red, n = 25 syllables in 5 birds) groups. No difference was found between the two adult groups (Wilcoxon test, post days 1–2, p=0.3, post days 3–4, p=0.9, post days 5–6, p=0.1). Bottom: CV of syllable relative amplitude in the sham (green) and lesion (red) groups. No difference in relative amplitude CV was observed for the four periods around lesion (Wilcoxon test, pre, p=0.4, post days 1–2, p=0.1, post days 3–4, p=0.4, post days 5–6, p=0.1).
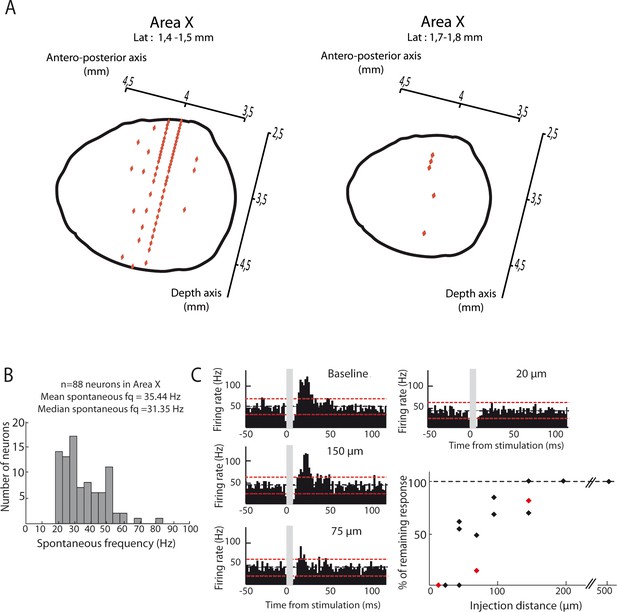
Location of Area X neural recordings and effect of the dispersion of pharmacological agents.
(A) Diagrams showing recordings locations in Area X, for two different lateral plans (1.4–1.5 mm (left panel) or 1.7–1.8 mm (right panel). Each recording point (red diamond) was placed in Area X using antero-posterior and depth coordinates (n = 83 recording sites for a laterality of 1.4–1.5 mm, left panel; n = 5 recording sites for a laterality of 1.7–1.8 mm, right panel). (B) Distribution of spontaneous firing rate for neurons recorded in Area X (n = 88 neurons, mean frequency = 35.4 Hz, median frequency = 31.4 Hz). (C) Effect of pharmacological blockers (CNQX/APV) on Area X neurons as a function of the distance from the injection site. An example pallidal neuron recorded while injecting the blockers at various distances (baseline: no drug injected): 150, 75 and 20 µm, and the PSTHs displayed show its response to the DCN stimulation after drug injection at the various sites (time bin: 5 ms). The black horizontal dashed line depicts the mean baseline firing rate and red dotted lines indicate confidence intervals (2.5 SD away from the mean baseline firing rate). The population data (bottom right) represent the change in response to DCN stimulation induced by drug injection as compared to baseline for each recorded neuron. Red diamonds correspond to the example shown here.
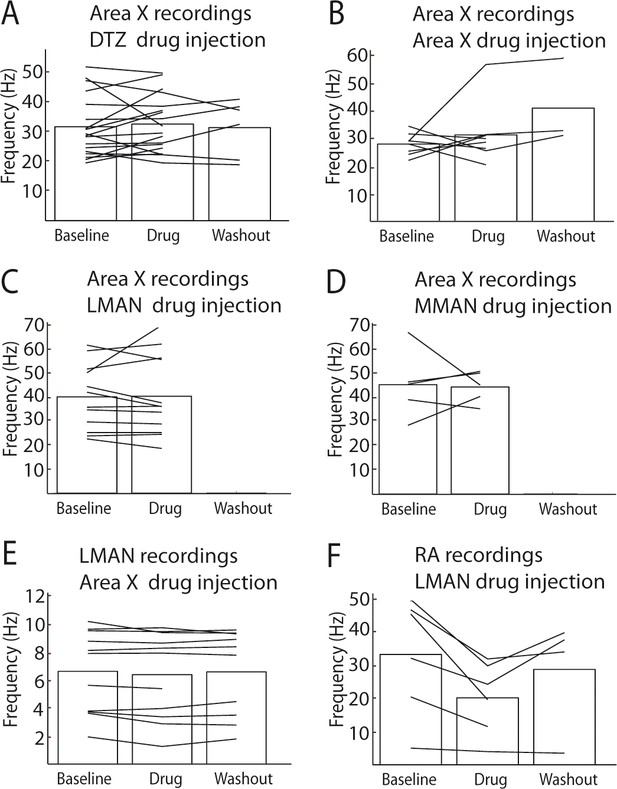
Effect of drug injections (NBQX/APV) on spontaneous activity.
(A) Effect of NBQX/APV injections in DTZ on the spontaneous activity of Area X pallidal neurons. No difference was observed between baseline and drug conditions (n = 16 neurons in 8 birds, paired Wilcoxon-test, p=0.4). (B) Effect of NBQX/APV injections on the spontaneous discharge of Area X pallidal neurons. No significant differences were observed in Area X pallidal neurons discharge between baseline and drug conditions (n = 8 neurons in 7 birds, paired Wilcoxon-test, non-significant, p=0.6). (C) Effect of NBQX/APV injections in LMAN on Area X pallidal neurons spontaneous discharge. No difference was observed between baseline and drug conditions (n = 12 neurons in 6 birds, paired Wilcoxon-test, p=0.6). (D) Effect of NBQX/APV injections in MMAN on Area X pallidal neurons spontaneous activity. No difference was observed between baseline and drug conditions (n = 5 neurons in 2 birds, paired Wilcoxon-test, p=1). (E) Effect of NBQX/APV injections in Area X on LMAN neurons spontaneous discharge. No difference was observed baseline and drug conditions (n = 14 multiunit recordings in 5 birds, paired Wilcoxon-test, p=0.1). (F) Effect of NBQX/APV injections in LMAN on RA neurons spontaneous activity. RA neurons activity decreased significantly following glutamatergic transmission blockade in LMAN (n = 6 neurons in 5 birds, paired Wilcoxon-test, p=0.03).
Tables
Stereotaxic coordinates summary.
Head and arm angle (on the mediolateral axis) are expressed in degrees, anteroposterior and mediolateral coordinates are expressed in millimeters from the sinus junction, and depth coordinates in millimeters from the surface of the brain. DCN: deep cerebellar nuclei. LMAN: lateral magnocellular nucleus of the nidopallium, MMAN: medial magnocellular nucleus of the nidopallium, HVC: used as a proper name, DTZ: dorsal thalamic zone.
Structure | Head angle (°) | Arm angle (°) | Antero-post (mm) | Medio-lateral (mm) | Depth (mm) |
---|---|---|---|---|---|
Area X | 50 | 0 | 4.0 | 1.5 | 3.0–4.0 |
50 | 15 | 4.0 | 2.7 | 3.5–4.5 | |
DCN | 50 | 15 | −2 | 2.5 | 3.5 |
50 | 0 | −1.5/–1.8/−2.1 | 1.3 | 3.4 | |
DTZ | 50 | 0 | −0.3 | 1.2 | 4.3–4.5 |
LMAN | 50 | 0 | 4.1 | 1.8 | 2.3–2.5 |
50 | 15 | 4.1 | 3.0 | 2.4–2.6 | |
MMAN | 50 | 0 | 4.1 | 0.5 | 2.3–2.5 |
50 | 15 | 4.1 | 1.7 | 2.4–2.6 |
Additional files
-
Supplementary file 1
Related to Figure 8.
Statistical values for Wilcoxon test with Bonferonni correction. For each period in each group (adults or juveniles and sham or lesioned birds) and each features (duration, fundamental frequency and amplitude) number of birds, number of syllables, mean, median, standard deviation and SEM were reported. p values for Wilcoxon test with Bonferonni correction were calculated for each repeated test. N.S.: non-significant.
- https://doi.org/10.7554/eLife.32167.022
-
Transparent reporting form
- https://doi.org/10.7554/eLife.32167.023