Thermoregulation: Bugs battle stress from hot blood
Over 14,000 species of insects, arachnids and other arthropods feed on the blood of vertebrates. This blood-feeding lifestyle appears to have evolved independently at least six times since the Jurassic and Cretaceous periods, and perhaps up to 20 times (Benoit et al., 2014; Lehane, 2005; Sterkel et al., 2017). Blood is notoriously devoid of many essential micronutrients, but it is a good source of the proteins and lipids that are essential for development and the generation of eggs in arthropods (Lehane, 2005; Coast, 2009; Sterkel et al., 2017).
Acquiring a blood meal is laden with considerable risks before, during and after feeding (Figure 1). For example, the sheer size difference between the vertebrate and the blood-feeder makes the defensive actions of the host potentially lethal for the arthropod, and so must be avoided. One strategy commonly used to avoid detection is for the arthropod to ingest large blood meals to minimize how often feeding needs to occur. Such gluttony can lead to dramatic increases in size: mosquitoes and tsetse flies grow 2–3 times bigger after a blood meal (Benoit et al., 2014; Lehane, 2005; Coast, 2009), whereas ticks and kissing bugs (which spread Chagas disease in South America and the southern United States) expand 10–100 fold (Sonenshine and Roe, 2014).
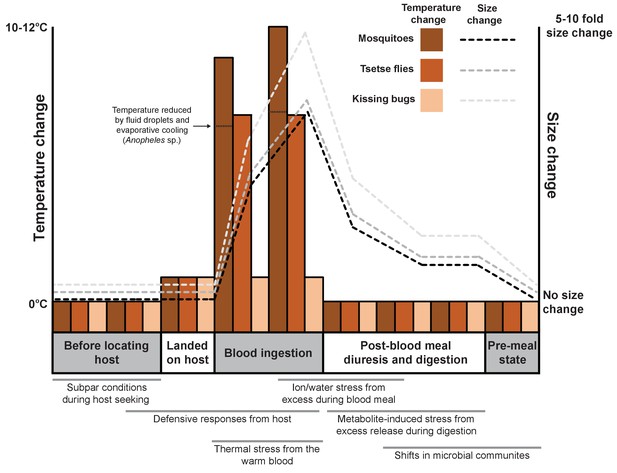
Feeding on blood leads to various physiological shifts in arthropods.
Changes in body temperature (bars; left axis) and size (dashed lines; right axis) for three species of arthropod (mosquitoes, tsetse flies and kissing bugs) before, during and after feeding on the blood of a vertebrate. The process of blood feeding exposes the arthropods to a number of stresses that are likely to necessitate a biological response (shown under the graph; gray bars indicate when each stress is likely to occur).
The heat of the vertebrate represents a frequently overlooked stress associated with blood ingestion even though the temperature of blood-feeding arthropods may increase by up to 15°C in less than one minute during their meal (Benoit et al., 2011; Lahondère and Lazzari, 2012; Lahondère and Lazzari, 2015). It is known that the thermal stress generated by the blood meal can trigger the arthropod’s heat shock response, as demonstrated by the increased production of heat shock proteins (Benoit et al., 2011).
Arthropods use a range of different mechanisms to reduce heat stress during blood feeding: some open small holes called spiracles on their surface to increase heat loss from breathing; the mosquito Anopheles stephensi (Lahondère and Lazzari, 2012) retains drops of urine on the abdomen that cool as they evaporate; and tsetse flies cool their meals by feeding from pools of blood instead of directly from blood vessels (Lahondère and Lazzari, 2015). Now, in eLife, Claudio Lazzari from the University of Tours and colleagues – including Chloé Lahondère as first author – report a heat exchange mechanism that enables kissing bugs to control their temperature as they ingest a warm blood meal (Lahondère et al., 2017).
Heat dissipates quickly from the head of a kissing bug while it feeds, allowing the rest of the body to remain at ambient temperature. This is in stark contrast to what happens in many of the other blood-feeding arthropods examined by Lahondère et al. (most of the body increases to a temperature near that of the host (Lahondère and Lazzari, 2012; Lahondère and Lazzari, 2015).
Using histology, micro-computed tomography and X-ray synchrotron imaging, Lahondère et al. – who are based at Tours, the Universidade Federal de Minas Gerais, the University of Saskatchewan and the Canadian Light Source Inc. – noted the close proximity of the circulatory and ingestion systems in the head. They suggested that the flow of the kissing bug’s blood toward the head could help to cool the blood meal as it moves through the ingestion system, before it reaches the thorax and abdomen. This is an example of a countercurrent heat exchanger – a system where two fluids that flow in opposite directions act to reduce the temperature difference between them.
To test this hypothesis Lahondère et al. conducted a set of experiments in which they stopped blood circulating around the kissing bug by severing the dorsal vessel. This intervention caused the abdominal temperature of the bug to soar to near that of the host. This triggered the production of more heat shock proteins in the bug, and demonstrates that the head thermal exchanger plays a critical role in dissipating heat from the blood meal.
When an arthropod ingests a blood meal, there are a multitude of stresses that must be prevented or tolerated. As well as heat stress, arthropods must eliminate large amounts of excess water and ions (Beyenbach and Piermarini, 2011), detoxify the harmful products that result from a high-protein diet (Sterkel et al., 2017), and tolerate massive increases in the number of bacteria in their gut (Wang et al., 2011). By improving our understanding of the protective mechanisms used by blood-feeding arthropods to counter bouts of blood meal-induced stress, the work of Lahondère et al. will help with efforts to develop new ways to prevent blood feeding and reduce the spread of diseases carried by blood-feeding arthropods.
References
-
Neuroendocrine control of ionic homeostasis in blood-sucking insectsJournal of Experimental Biology 212:378–386.https://doi.org/10.1242/jeb.024109
-
Thermal effect of blood feeding in the telmophagous fly Glossina morsitans morsitansJournal of Thermal Biology 48:45–50.https://doi.org/10.1016/j.jtherbio.2014.12.009
Article and author information
Author details
Publication history
Copyright
© 2017, Benoit et al.
This article is distributed under the terms of the Creative Commons Attribution License, which permits unrestricted use and redistribution provided that the original author and source are credited.
Metrics
-
- 1,165
- views
-
- 103
- downloads
-
- 11
- citations
Views, downloads and citations are aggregated across all versions of this paper published by eLife.
Download links
Downloads (link to download the article as PDF)
Open citations (links to open the citations from this article in various online reference manager services)
Cite this article (links to download the citations from this article in formats compatible with various reference manager tools)
Further reading
-
- Ecology
- Evolutionary Biology
Eurasia has undergone substantial tectonic, geological, and climatic changes throughout the Cenozoic, primarily associated with tectonic plate collisions and a global cooling trend. The evolution of present-day biodiversity unfolded in this dynamic environment, characterised by intricate interactions of abiotic factors. However, comprehensive, large-scale reconstructions illustrating the extent of these influences are lacking. We reconstructed the evolutionary history of the freshwater fish family Nemacheilidae across Eurasia and spanning most of the Cenozoic on the base of 471 specimens representing 279 species and 37 genera plus outgroup samples. Molecular phylogeny using six genes uncovered six major clades within the family, along with numerous unresolved taxonomic issues. Dating of cladogenetic events and ancestral range estimation traced the origin of Nemacheilidae to Indochina around 48 mya. Subsequently, one branch of Nemacheilidae colonised eastern, central, and northern Asia, as well as Europe, while another branch expanded into the Burmese region, the Indian subcontinent, the Near East, and northeast Africa. These expansions were facilitated by tectonic connections, favourable climatic conditions, and orogenic processes. Conversely, aridification emerged as the primary cause of extinction events. Our study marks the first comprehensive reconstruction of the evolution of Eurasian freshwater biodiversity on a continental scale and across deep geological time.
-
- Ecology
- Neuroscience
Prey must balance predator avoidance with feeding, a central dilemma in prey refuge theory. Additionally, prey must assess predatory imminence—how close threats are in space and time. Predatory imminence theory classifies defensive behaviors into three defense modes: pre-encounter, post-encounter, and circa-strike, corresponding to increasing levels of threat—–suspecting, detecting, and contacting a predator. Although predatory risk often varies in spatial distribution and imminence, how these factors intersect to influence defensive behaviors is poorly understood. Integrating these factors into a naturalistic environment enables comprehensive analysis of multiple defense modes in consistent conditions. Here, we combine prey refuge and predatory imminence theories to develop a model system of nematode defensive behaviors, with Caenorhabditis elegans as prey and Pristionchus pacificus as predator. In a foraging environment comprised of a food-rich, high-risk patch and a food-poor, low-risk refuge, C. elegans innately exhibits circa-strike behaviors. With experience, it learns post- and pre-encounter behaviors that proactively anticipate threats. These defense modes intensify with predator lethality, with only life-threatening predators capable of eliciting all three modes. SEB-3 receptors and NLP-49 peptides, key stress regulators, vary in their impact and interdependence across defense modes. Overall, our model system reveals fine-grained insights into how stress-related signaling regulates defensive behaviors.