Spatio-temporal regulation of concurrent developmental processes by generic signaling downstream of chemokine receptors
Figures
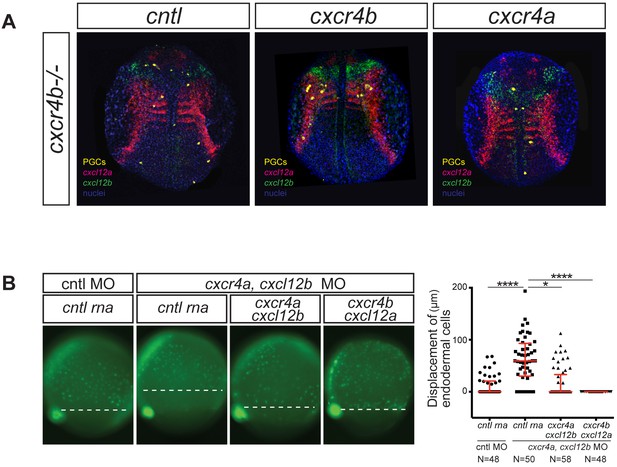
The signaling cascades initiated by Cxcr4a and Cxcr4b are functionally equivalent.
(A) The position of PGCs (detected by nanos3 RNA expression, yellow) relative to tissues expressing cxcl12a (magenta) and cxcl12b (green) RNAs in 12 hpf cxcr4b-/- embryos. PGCs express control RNA (cntl, left panel) or RNAs encoding for Cxcr4b (middle panel) or Cxcr4a (right panel). 20 pg of control RNA and RNA encoding for Cxcr4a and Cxcr4b were injected. (B) Epifluorescence image of transgenic sox17::gfp embryos at 8 hpf. The embryos were injected with control (cntl) morpholino and control RNA (left panel). Experimental embryos were knocked down for Cxcr4a and Cxcl12b and the effect of control RNA, cxcr4a and Cxcl12b or cxcr4b along with cxcl12a mRNAs was examined. The quantitation of the endoderm displacement with respect to the forerunner cells is presented in the graph on the right. See also Figure 1—figure supplement 1. 0.8 pmol of each morpholino was used. 100 pg of receptor and 50 pg of ligand encoding mRNA was used, as well as equimolar amounts of control RNA.
-
Figure 1—source data 1
Source data file contains the results of the measured displacement of endoderm from forerunner cells under different experimental conditions.
The data shows that the expression of Cxcr4b together with Cxcl12a can reverse the phenotype of displaced endoderm in Cxcr4a morphants. Three biological replicates are presented.
- https://doi.org/10.7554/eLife.33574.005
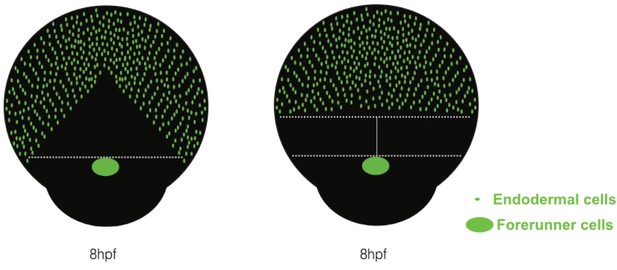
Cartoon showing the procedure employed for measuring the displacement between endoderm and forerunner cells.
https://doi.org/10.7554/eLife.33574.004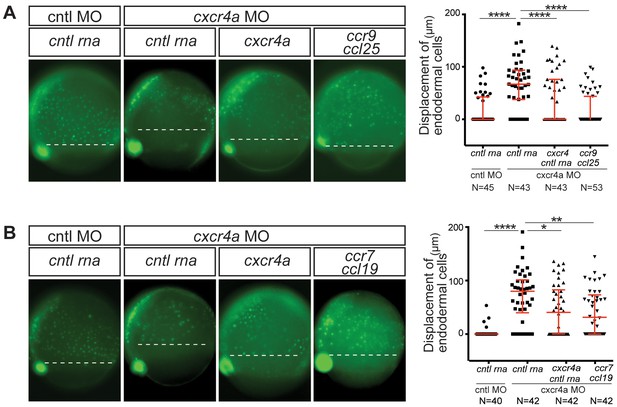
CC receptors can control a process regulated by CXC receptors.
(A) Epifluorescence image of sox17::gfp transgenic control embryos (left panel) and embryos knock down for Cxcr4a. The Cxcr4a knocked down embryos were injected with control RNA (cntl) or with cxcr4a RNA, or co-injected with ccr9 RNA and its ligand ccl25 RNA. The quantitation of the endoderm displacement with respect to the forerunner cells is presented in the graph on the right. See also Figure 1—figure supplement 1. (B) Epifluorescence image of sox17::gfp transgenic control embryos (left panel) and of embryos knocked down for Cxcr4a. The embryos were injected with control RNA, with cxcr4a RNA, or co-injected with ccr7 RNA and its ligand ccl19 RNA. The quantitation of the endoderm displacement with respect to the forerunner cells is presented in the graph on the right. 0.8 pmol of each morpholino was used. 100 pg of receptor and 50 pg of ligand encoding mRNA was used, as well as equimolar amounts of control RNA.
-
Figure 2—source data 1
Cxcr4b can initiate signaling cascade functionally equivalent to Cxcr4a in endoderm cells.
Source data contains two files, Figure 2—source data 1A contains the results of the measured displacement of endoderm from forerunner cells under different experimental conditions. The data shows that the expression of Ccr9 together with Ccl25 can reverse the phenotype of displaced endoderm in Cxcr4a morphants. Figure 2—source data 1B contains data showing that expression of Ccr7 together with Ccl19 can reverse the phenotype of displaced endoderm in Cxcr4a morphants. Three biological replicates are presented for both the experiments.
- https://doi.org/10.7554/eLife.33574.009
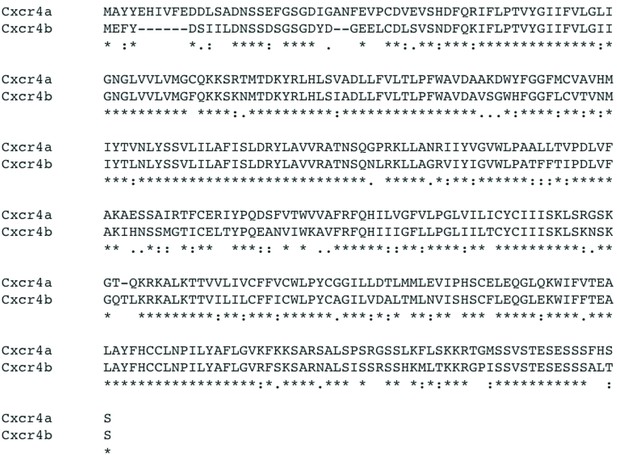
Protein sequence alignment of Cxcr4a and Cxcr4b using Clustal omega.
(*)=Fully conserved residue. (:)=Conservation among groups of strongly similar properties, (.)=Conservation among group of weakly similar properties.
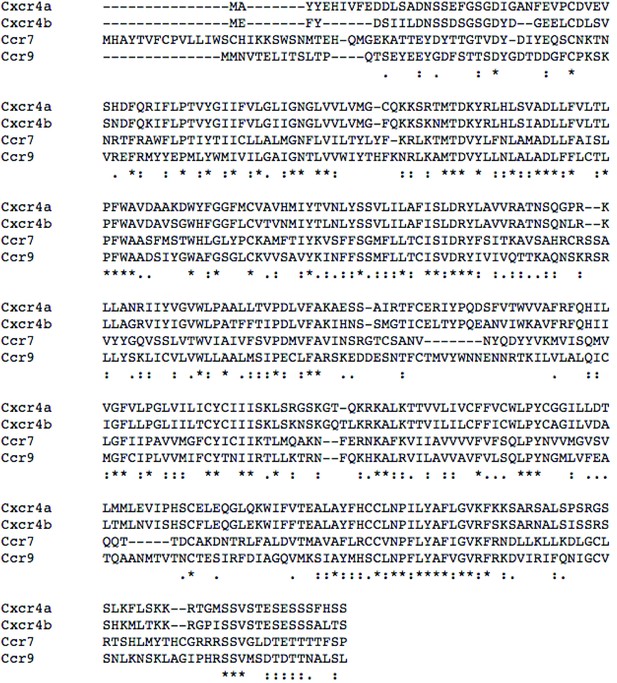
Protein sequence alignment of Cxcr4a, Cxcr4b, Ccr7 and Ccr9 using Clustal omega.
(*)=Fully conserved residue. (:)=Conservation among groups of strongly similar properties, (.)=Conservation among group of weakly similar properties.
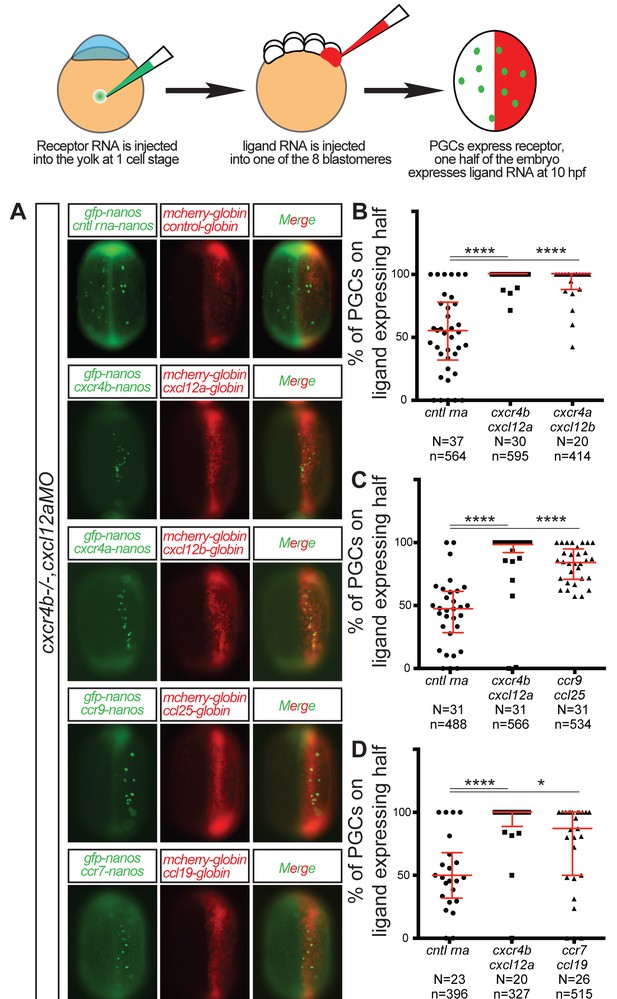
CC and CXC receptors can direct the migration of primordial cells.
The experimental scheme is provided at the top. (A) Epifluorescence image of 10 hpf embryos expressing the control RNA or RNA encoding for the indicated ligand (Cxcl12a, Cxcl12b, Ccl25 and Ccl19) in one half of the embryo and control RNA or Receptor-encoding RNA (Cxcr4b, Cxcr4a, Ccr9 or Ccr7) in PGCs. Merged images show the position of PGCs with respect to control or ligand-expressing domains (red). (B–D) Graphs show the quantitation of the migration of PGCs as the percentage of GFP-labeled cells located within the ligand-expressing part of the embryos. 60 pg of mGFP-nanos was used to label PGCs in green and 40 pg of m-cherry mRNA was used for labeling the ligand expressing half of the embryo. 20 pg of receptor-encoding RNA was used and 30 pg of ligand-encoding RNA was used. 0.2 pmol of Cxcl12a morpholino was used. Equimolar amounts of control RNA were used. For raw data see Figure 3—source data 1.
-
Figure 3—source data 1
The data presents the percentage of PGCs located on the ligand expressing embryo half under conditions where the Cxcr4b and Cxcl12a proteins are not expressed.
Figure 3—source data 1B contains data showing that Cxcr4a can direct PGCs towards the Cxcl12b-expressing half. Figure 3—source data 1C contains data showing that Ccr9 can direct PGCs toward the Ccl25 expressing half. Figure 3—source data 1D contains data showing that Ccr7 can direct PGCs toward Ccl19 expressing half. Three biological replicates are presented for each experiment.
- https://doi.org/10.7554/eLife.33574.013
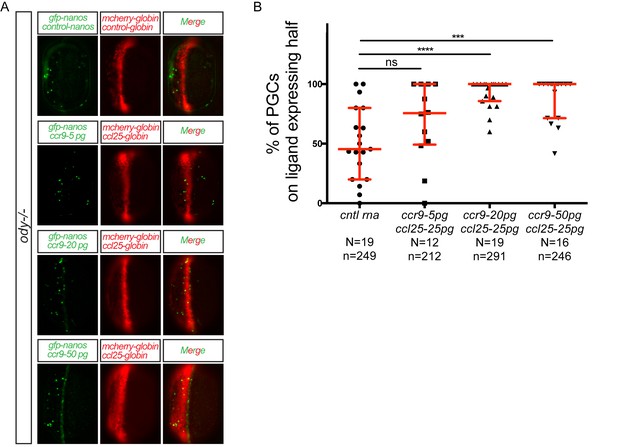
Different levels of chemokine receptor signaling leads to functionally same response.
(A) Epifluorescence images of 10 hpf embryos. Embryos express the control RNA or the RNA encoding for Ccl25 in one half of the embryo and control RNA or different concentrations of RNA encoding for Ccr9 in PGCs. Merged images show the position of PGCs with respect to control or ligand-expressing domains (red). (B) Graphs show the quantitation of the migration of PGCs as the percentage of GFP-labeled cells located within the ligand-expressing domain of the embryos. 60 pg of mGFP-nanos was used to label PGCs in green and 40 pg of m-cherry mRNA was used for labeling the ligand expressing half of the embryo. For raw data see Figure 3—figure supplement 1—source data 1.
-
Figure 3—figure supplement 1—source data 1
The file contains data presenting the percentage of PGCs expressing different amounts of Ccr9 located within the Ccl25-expressing half of the embryo.
Three biological replicates are presented for each experiment.
- https://doi.org/10.7554/eLife.33574.012
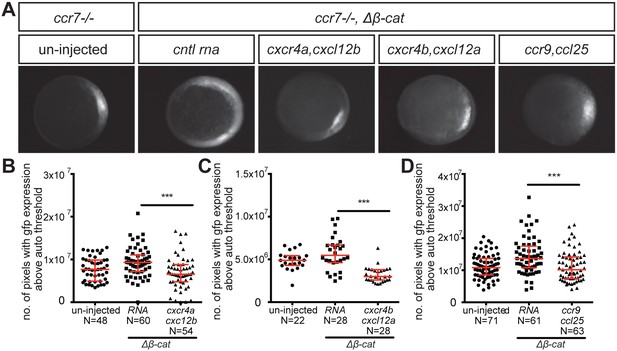
Control of dorsoventral axis specification by different chemokine receptors.
(A) Epifluorescence image of 5 hpf ccr7-/- mutant embryos carrying the gsc::gfp transgene. Uninjected embryo (left panel) and embryos sensitized by injection of Δβ-Catenin encoding RNA (right panels) are presented. The embryos were also injected with control RNA (cntl) or with RNA encoding for different chemokine receptors (Cxcr4a, Cxcr4b or Ccr9) and their cognate ligands (Cxcl12b, Cxcl12a or Ccl25). (B–D) Graphs showing the area of the goosecoid expression domain determined by quantifying the number of pixels with GFP signal above the auto threshold in the different treatments. 100 pg of mRNA encoding for the receptors was injected and 60 pg of RNA encoding for the ligands. 2.5 pg of Δβ-Catenin-encoding RNA was inject to sensitize the embryos. Equimolar amounts of control RNA were used. For raw data see Figure 4—source data 1.
-
Figure 4—source data 1
The data presents the number of pixels showing GFP expression above the threshold (Area of goosecoid RNA expression) in embryos under different experimental conditions.
Figure 4—source data 1B shows that expression of Cxcr4a together with Cxcl12b lead to a reduction in the area of goosecoid expression. Figure 4—source data 1C shows that expression of Cxcr4b together with Cxcl12a lead to a reduction in the area of goosecoid expression. Figure 4—source data 1D shows that expression of Ccr9 together with Ccl25 lead to a reduction in the area of goosecoid expression. A minimum of three biological replicates are presented for each experiment.
- https://doi.org/10.7554/eLife.33574.017
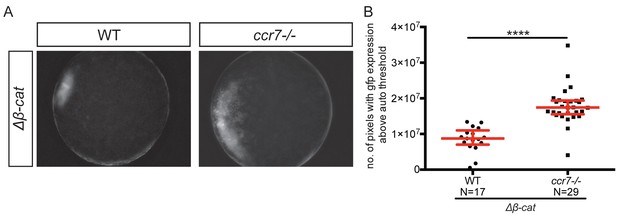
Embryos lacking Ccr7 are hypersensitive to very low dose of Δβ-catenin.
(A) Expansion of the goosecoid expression domain in early embryos. Epifluorescence image of wild-type (WT) tg gsc::gfp and ccr7-/- gsc::gfp embryos sensitized by injection of 2.5 pg of Δβ catenin RNA. (B) Graph showing the area of goosecoid domain measured by quantifying the number of pixels with GFP signal above the auto threshold.
-
Figure 4—figure supplement 1—source data 1
The data presents the number of pixels showing GFP expression above the threshold (Area of goosecoid RNA expression) in ccr7-/- and WT embryos sensitized by injection of Δβ-catenin RNA.
Three biological replicates are presented for each experiment.
- https://doi.org/10.7554/eLife.33574.016
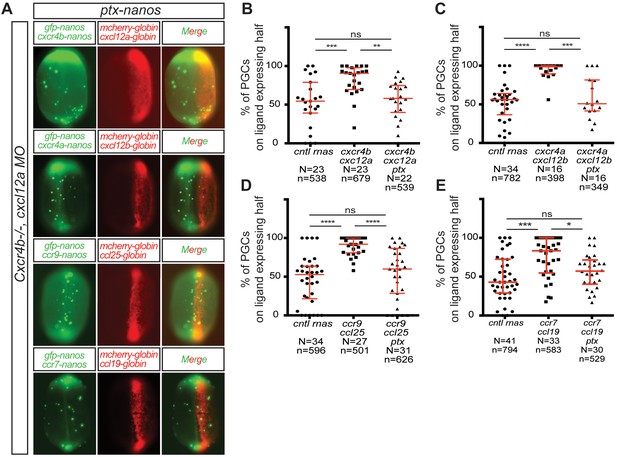
CC and CXC receptors can regulate the migration of primordial cells employing the same downstream signaling pathway.
(A) Epifluorescence image of 10 hpf embryos expressing different ligands (Cxcl12a, Cxcl12b, Ccl25 and Ccl19) in one half of the embryo (mCherry-expressing cells) and PGCs (mGFP) expressing different chemokine receptors (Cxcr4b, Cxcr4a, Ccr9 and Ccr7) along with PTX. Merged images show the position of PGCs with respect to control (cntl), or ligand-expressing cells. (B–E) Graphs showing the quantitation of directed cell migration, by presenting the percentage of PGCs located within the ligand-expressing domains. 60 pg of mGFP-nanos was used to label PGCs in green and 40 pg of m-cherry-globin mRNA was used for labeling the ligand-expressing half of the embryo. 10 pg of PTX-encoding RNA were injected to inhibit the Gi protein. 20 pg of receptor-encoding RNA was used and 30 pg of ligand-encoding RNA was used. 0.2 pmol of cxcl12a morpholino was used. Equimolar amounts of cntl RNA were used. For raw data see Figure 5—source data 1 .
-
Figure 5—source data 1
The data presents the percentage of PGCs expressing pertussis toxin present on ligand expressing embryo half.
Figure 5—source data 1B shows that Cxcr4b cannot direct PGCs expressing PTX towards the Cxcl12a expressing half. Figure 5—source data 1C shows that Cxcr4a cannot direct PGCs expressing ptx toward the Cxcl12b expressing embryo half. Figure 5—source data 1D shows that Ccr9 cannot direct PGCs expressing PTX toward the Ccl25 expressing embryo half. Figure 5—source data 1E shows that Ccr7 cannot direct PGCs expressing ptx toward Ccl19 expressing embryo half. Minimum of three biological replicates are presented for each experiment.
- https://doi.org/10.7554/eLife.33574.021
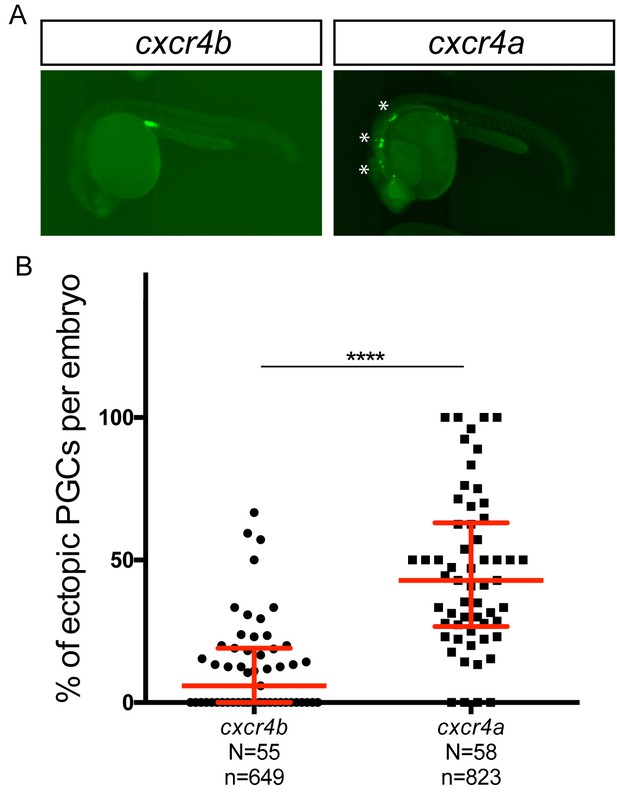
Overexpression of Cxcr4a in wild-type PGCs leads to increase in number of ectopic PGCs.
(A) Wild-type embryos expressing Cxcr4b (left) or Cxcr4a (right) in their PGCs. (B) Graph showing percentage of ectopic PGCs per embryo. 80 pg of RNA encoding for either Cxcr4a or Cxcr4b was injected into the embryo. The inclusion of the nanos 3’UTR leads to preferential expression of the proteins in the PGCs. 60 pg of mGFP-nanos RNA was used to label PGCs in green. For raw data see Source data file Figure 5—figure supplement 1.
-
Figure 5—figure supplement 1—source data 1
The file contains data presenting percentage of ectopic PGCs per embryo.
The data shows that PGCs expressing Cxcr4a are present at ectopic locations within the embryo. Three biological replicates are presented.
- https://doi.org/10.7554/eLife.33574.020
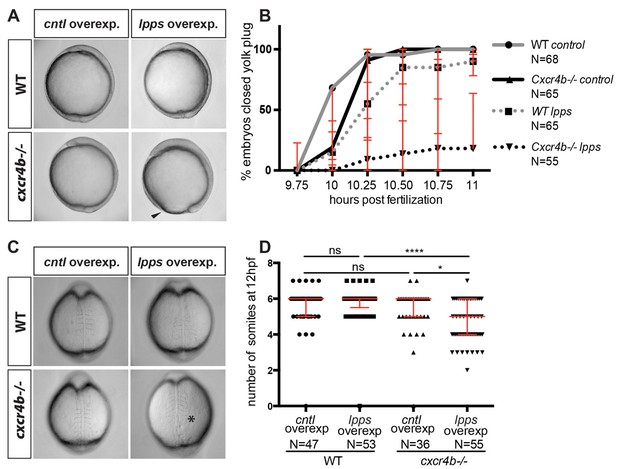
Functional compensation between G-protein-coupled receptors from different groups.
(A) Brightfield images showing 10 hpf embryos overexpressing RNA encoding for LPPs (lpp1-varX1, lpp3-varX1) or control RNA. Wild-type (WT) embryos overexpressing Lpps and embryos lacking Cxcr4b function (cxcr4b-/-) developed normally. Cxcr4b-deficient embryos overexpressing Lpps exhibited a delay in gastrulation and failed to close the yolk plug. (B) Graph showing the percentage of embryos with closed yolk plug between 9.75 and 11 hpf. (C) Brightfield images of embryos at 12 hpf. WT embryos overexpressing Lpps and embryos lacking Cxcr4b function (cxcr4b-/-) developed normally and formed six somites by this stage. Embryos lacking Cxcr4b function (cxcr4b-/-) that overexpress Lpps developed only five somites that appear abnormal. (D) Graph showing the number of somites at 12 hpf in WT and cxcr4b-/- embryos expressing control or Lpps encoding RNA. 75 pg of RNA encoding each Lpp was injected. Equimolar amounts of control RNA were used. For raw data see Figure 6—source data 1.
-
Figure 6—source data 1
GPCRs from different groups cooperate during gastrulation and somitogenesis.
Figure 6—source data 1B contains data showing the proportion of cxcr4b-/- and WT embryos expressing control or lpps RNA that completed gastrulation between 9.5 hpf and 11 hpf. Figure 6D—source data 1 presents data showing the number of somites in cxcr4b-/- and WT 12 hpf embryos expressing control or lpps RNA. Three biological replicates are presented for each experiment.
- https://doi.org/10.7554/eLife.33574.023
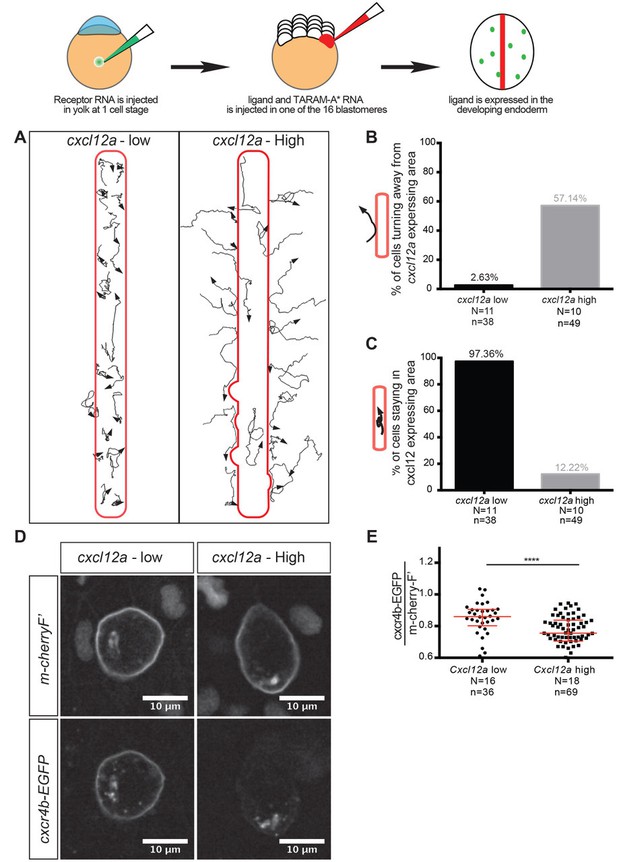
Regulation of reverse migration of PGCs by receptor internalization.
(A) Tracks of PGCs with respect to domains in the embryo expressing low or high levels of Cxcl12a, in embryos lacking the endogenous ligand. PGCs migrated into domains expressing low levels of the attractant and remained within them, while the cells turned away when the domains expressed high levels of Cxcl12a. (B) Graph showing percentage of cells that moved away from the Cxcl12a expressing domains (2.6% in the case of low Cxcl12a expression and 57.1% in the case of high Cxcl12a levels). (C) Graph showing the percentage of cells that remained for 90 minutes or more within the Cxcl12a expressing area 97.4% in the case of the low expression of the chemokine and 12.2% in the case of high Cxcl12a expression). (D) High-magnification images of PGCs expressing EGFP-tagged Cxcr4b and farnesylated mCherry on their membranes. The cells interacted with low and high Cxcl12a expressing domains as in A. (E) Graph showing the level of Cxcr4b on the PGC membrane as a ratio between the EGFP signal and that of the farnesylated mCherry in cells exposed to low and high concentrations of the ligand. 20 pg of cxcr4b-nanos, 400 pg and 25 pg of cxcl12a RNA was injected to achieve high and low expression domains; 60 pg of mGFP-nanos was used to label the PGCs; 30 pg of m-cherry-H2B was used to label the cells expressing Cxcl12; and 2 pg of TARAM-A*. 101 pg of cxcr4b-EGFP-nanos was used in the receptor internalization assay and 60 pg of m-cherry-nanos was used to label the membrane of PGCs. 0.2 pmol of Cxcl12a morpholino was used. Equimolar amounts of control RNA were used. For raw data see Figure 7—source data 1.
-
Figure 7—source data 1
PGCs undergo reverse migration upon exposure to high amount of chemoattractant.
Figure 7—source data 1A,B,C contains data from 180 min long time-lapse movies. The data represent number of PGCs that turned away or remained within the Cxcl12a expressing region. 1 out of 16 blastomeres was injected with high (400 pg) or low (25 pg) amounts of RNA encoding for Cxcl12a as well as with RNA encoding for the activated version of TARAM-A that direct the cells to the endodermal lineage. Figure 7—source data 1E presents the intensity of the mcherry F’ signal and Cxcr4b-EGFP signal on the membrane of PGCs exposed to the low or high amount of Cxcl12a. A minimum of three biological replicates are presented for each experiment.
- https://doi.org/10.7554/eLife.33574.027
Two examples of 10X time-lapse epifluorescence images of PGCs responding to low levels of Cxcl12a expressed in the endoderm (25 pg RNA injected).
PGCs are labeled in green and endoderm in red. PGCs remain within the ligand-expressing region.
Three examples of 10X time-lapse epifluorescence images of PGC response to high levels of Cxcl12a expressed in the endoderm (400 pg RNA injcted).
PGCs are labeled in green and endoderm in red. PGCs often turn away from the ligand expressing tissue.
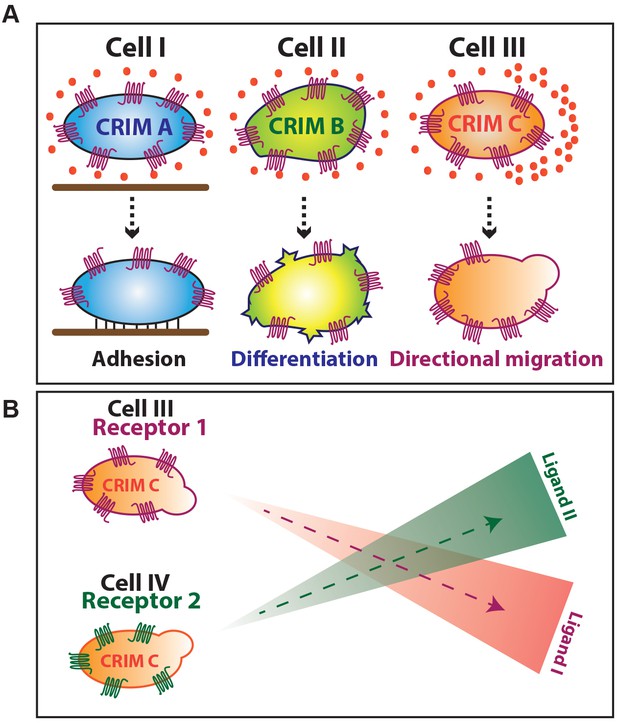
Graphical representation of Chemokine Receptor-signal Interpretation Module (CRIM).
(A) Graphical summary of results. (B) Schematic representation of concurrent trafficking of different cell types possessing identical CRIM and expressing different chemokine receptors.
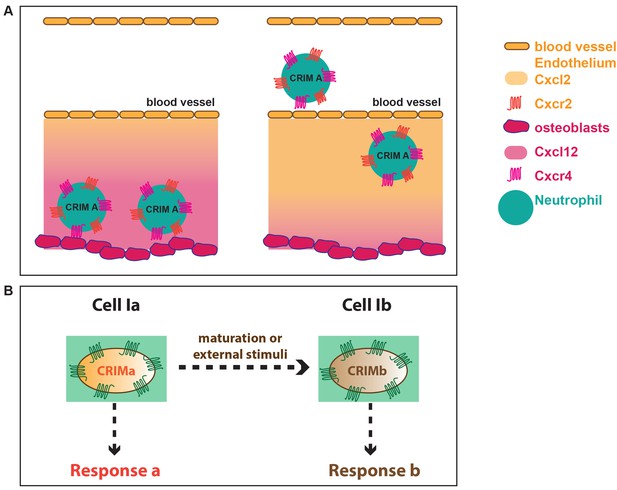
Graphical explanation of the effect of CRIM on cell behavior.
(A) Positioning of cells by different ligands expressed in spatially distinct locations. (B) Schematic explanation of a scenario showing cells altering their CRIM following maturation.
Additional files
-
Supplementary file 1
Table 1: list of constructs used in the study.
Table 2: list of primers used in the study. Table 3: List of Morpholinos used in the study
- https://doi.org/10.7554/eLife.33574.030
-
Transparent reporting form
- https://doi.org/10.7554/eLife.33574.031