Developmental deprivation-induced perceptual and cortical processing deficits in awake-behaving animals
Figures
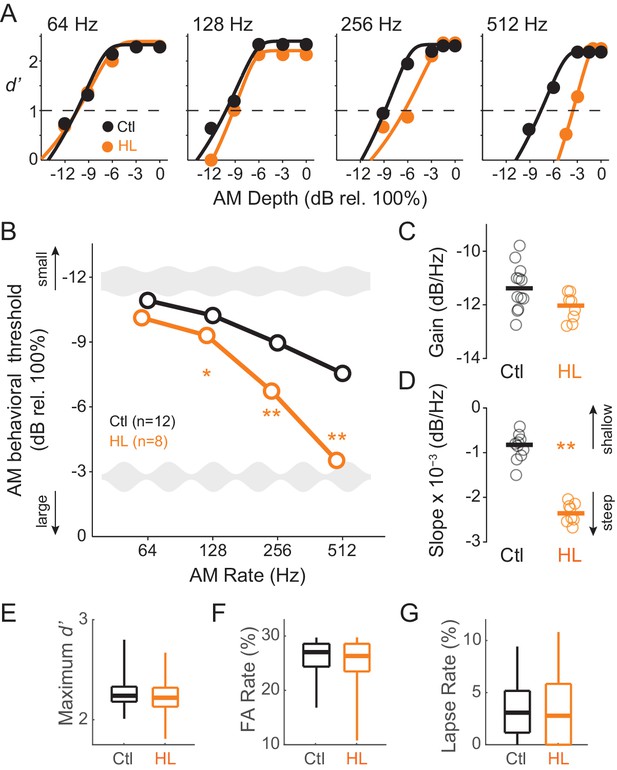
Developmental conductive hearing loss impairs AM rate detection.
(A) Psychometric functions across four tested AM rates (64, 128, 256, and 512 Hz) from a single testing session for a representative Ctl (black) and HL (orange) animal. (B) Average temporal modulation transfer functions for Ctl and HL animals. Mean ±1 SEM. Error bars are smaller than symbol dimensions. (C) Distribution of gain values extracted from each animal’s fitted exponential function. (D) Distribution of slope values extracted from each animal’s fitted exponential function. (E) Boxplots of maximum d’ from all test sessions of Ctl and HL animals. (F) Boxplots of false alarm (FA) rate from all test sessions of Ctl and HL animals. (G) Boxplots of lapse rate from all test sessions of Ctl and HL animals. Thick horizontal bars represent median values. The boxplots span the 25th to 75th distribution percentiles, and the whiskers span the 5th and 95th distribution percentiles. *p<0.05; **p<0.0001.
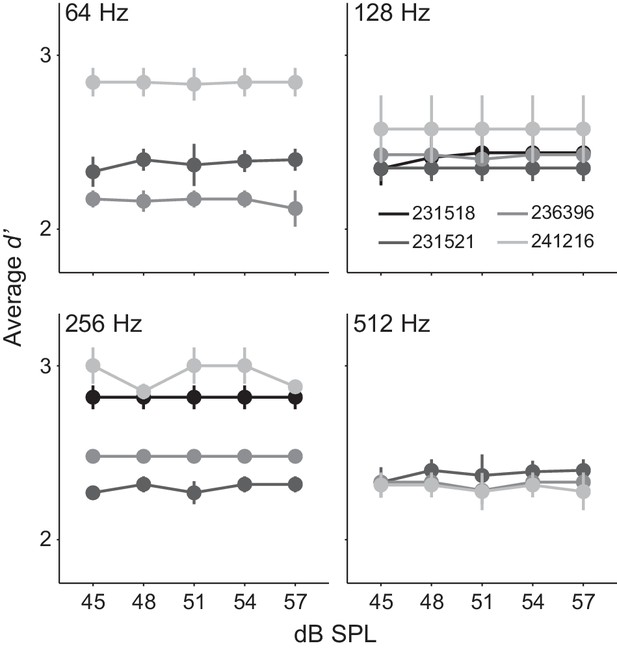
Gerbils did not use an average level cue.
Average d’ across 12 dB SPL sound levels from 4 Ctl animals tested at 64, 128, 256, and 512 Hz AM rates. Mean ± SEM.
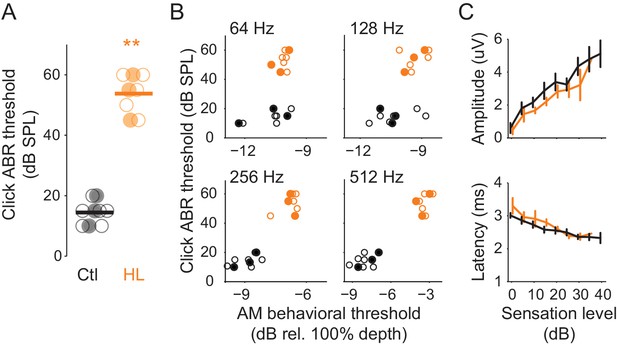
HL-related AM detection deficits cannot be explained by elevated ABR thresholds.
(A) Click ABR thresholds from Ctl (black) and HL (orange) animals. ABR thresholds from HL animals were significantly greater than those from Ctl animals (**p<0.0001). Horizontal bars represent the group means. (B) Individual behavioral AM detection thresholds (x-axis) do not correlate with ABR click thresholds (y-axis). For HL animals, pearson r values ranged between 0.27–0.52, p>0.05. For Ctl animals, pearson r values ranged between 0.35–0.68, p>0.05. Filled symbols correspond to gerbils used for extracellular ACx recordings. (C) Mean ± SEM of maximum ABR amplitude (top) and latency (bottom) plotted as a function of sensation level (dB).
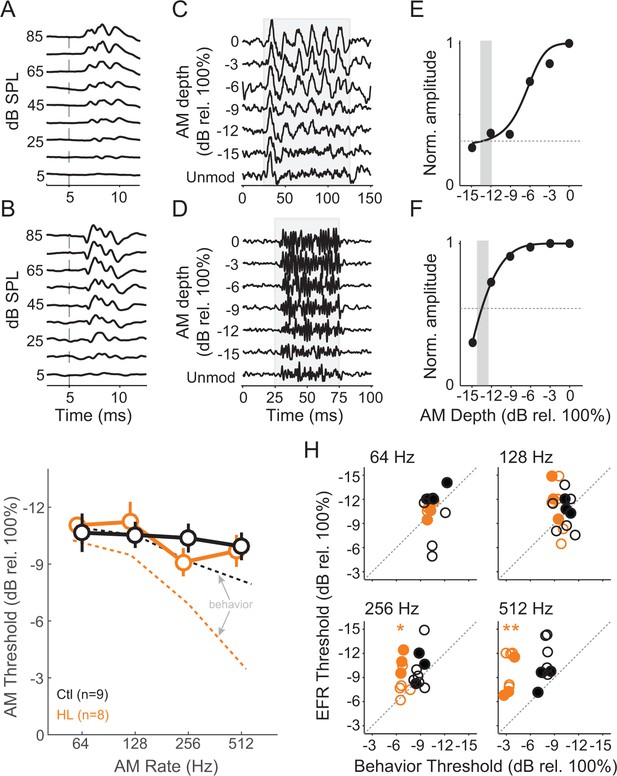
HL-related AM detection deficits cannot be explained by brainstem temporal processing.
(A,B) Example click-evoked ABR traces across dB SPL recorded with separate electrode positions. Vertical dashed-line indicates stimulus onset. (C,D) Representative EFR to 64 Hz (C) and 512 Hz (D) AM noise across depths from pin electrode positions in (A) and (B), respectively. (E,F) Normalized FFT values as a function of AM depth corresponding to 64 Hz (E) and 512 Hz (F). (G) Average temporal modulation transfer functions extracted from EFRs from Ctl (black) and HL (orange) animals. Mean ± SEM. Dashed lines represented average behavior TMTFs. (H) Within groups post-hoc comparisons between EFR versus behavior detection thresholds across 64, 128, 256, and 512 Hz AM rates. *p<0.05; **p<0.0001; Holm-Bonferroni- corrected. Filled symbols correspond to gerbils used for awake-behaving ACx recordings.
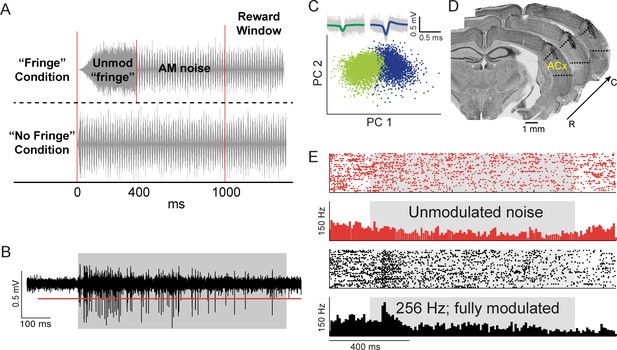
Candidate waveform selection for neurometric analyses.
(A) Schematic of AM stimuli. For a majority of sessions, unmodulated and AM stimuli had a 200 ms onset ramp, followed by an unmodulated period of 200 ms that would maintain as unmodulated or transition to an AM signal (‘Fringe’ Condition). For the remaining number of sessions, the stimuli had cosine-ramped onsets with a 25 ms rise and fall time (‘No Fringe’ Condition). (B) Raw waveform trace of neural response to AM noise (gray shaded region). Red line represents selection criteria of >4 SDs above noise floor. (C) Principal component analysis plot where two waveform clusters (green and blue) are separated. Raw waveforms (gray lines) and averages (green and blue) are displayed within insets. (D) Representative electrode track from Nissl-stained coronal sections from one animal. Sections are arranged from rostral (‘R’) to caudal (‘C’) spanning 180 µm and show electrode track and lesion sites within ACx. (E) Example raster and PSTH for one unit in response to unmodulated noise (red) and 256 Hz AM at 100% modulation depth (black). Gray shaded region indicates period when stimulus was present.
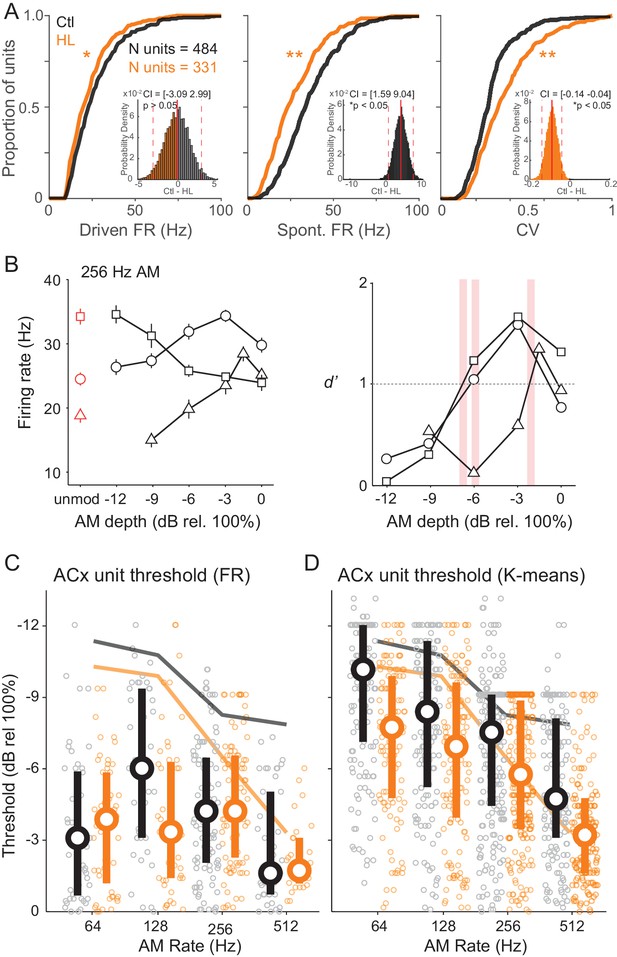
Cortical representation of fast AM rates.
(A) Cumulative distributions of maximum stimulus-driven FR (left), spontaneous FR (middle), and CV (FR standard deviation/average FR; right) for Ctl (black) and HL (orange) units. Inset: Corresponding distributions of average between group differences from a bootstrapped statistical test. Solid and dashed red vertical lines represent the distribution average and 98.75% (two-tailed) confidence intervals (CIs), respectively. A statistically significant difference between groups tested at p<0.05 was determined if zero fell outside the 98.75% CIs. (B) Left: Firing rate responses from three example ACx units to 256 Hz AM noise across modulation depths (mean ± SEM). Right: Corresponding neurometric functions. Red vertical bars indicate each unit’s threshold (d’=1). (C) Distribution of FR-based AM thresholds across AM rates for Ctl and HL units. (D) Distribution of classifier-based (K-means) AM thresholds across AM rates. For C and D, symbols represent individual units, whereas large circles and thick vertical lines represent medians and interquartile range, respectively. Thin solid lines represent average behavior TMTFs.
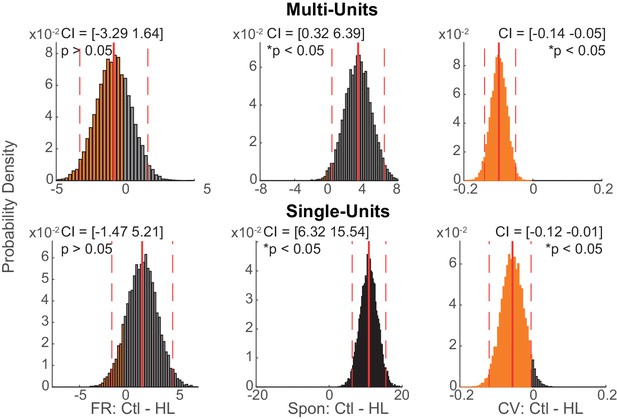
Multi- and single-unit data were analyzed separately with a bootstrapped statistical procedure.
https://doi.org/10.7554/eLife.33891.008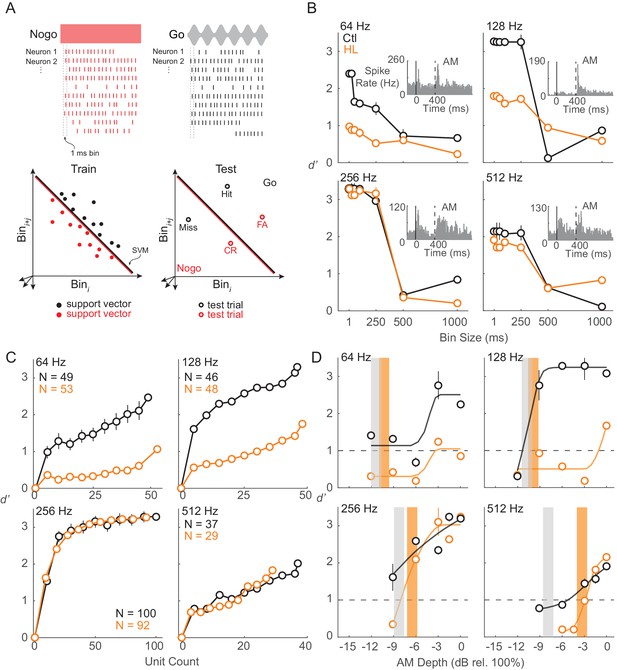
ACx population decoder analysis can explain HL-related behavioral deficits.
(A) Assessing population encoding by measuring discriminability with a linear population readout. Hypothetical population responses for single trials of a Nogo (red) and Go (black) stimulus. Spikes were counted across 1 ms bins. Spike firing responses from N neurons were counted across 1 ms bins to T trials of S stimuli (‘Go’ and ‘Nogo’) and formed a population ‘response vector’. 80% of trials were randomly sampled (without replacement) and averaged across N neurons, reducing the response vector to length Nbin (shown across two dimensions here), and fitted to a linear hyperplane that was determined by a support vector machine (SVM) procedure (‘train set’). Symbols represent ‘support vectors’, which are points used to create the linear boundary. Cross-validated classification performance was assessed on the remaining 20% of trials (‘test’ set). (B) Average population decoder performance between fully modulated AM noise (‘Go’) versus unmodulated noise (‘Nogo’) as a function of bin size. Example unit responses to fully modulated AM noise are shown within each subpanel. Vertical solid and dashed lines represent unmodulated noise fringe and AM noise onset, respectively. (C) Average population decoder performance between fully modulated AM noise versus unmodulated noise as a function of unit count. (D) Average population decoder performance (d') for each tested AM rate as a function of AM depth from Ctl (black) and HL (orange) unit populations. Sigmoidal functions were fitted to the data for visual purposes and are not statistically validated. Vertical lines represent average behavior AM depth thresholds. Error bars represent ± 1 standard deviation across 250 iterations.
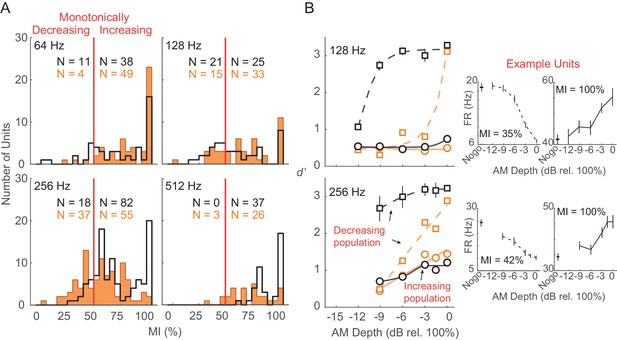
Monotonically increasing versus monotonically decreasing units.
(A) Distribution of monotonicity index (MI) values from all Ctl (black) and HL (orange) units across 64, 128, 256, and 512 Hz AM rate conditions. Solid vertical red line represents the classification cutoff value (MI = 50%). Units with MI values ≤ 50% (>50%) were classified as monotonically decreasing (monotonically increasing). (B) Average population decoder performance from subpopulations of monotonically decreasing (dashed lines) and monotonically increasing (solid lines) units. Sigmoidal functions were fitted to the data for visual purposes and are not statistically validated. Data from 64 and 512 Hz conditions are not shown since very few monotonically decreasing units were classified. Error bars represent ± 1 standard deviation across 250 iterations. Individual example monotonically decreasing and monotonically increasing units are shown (B, right) with error bars representing ± 1 SEM.
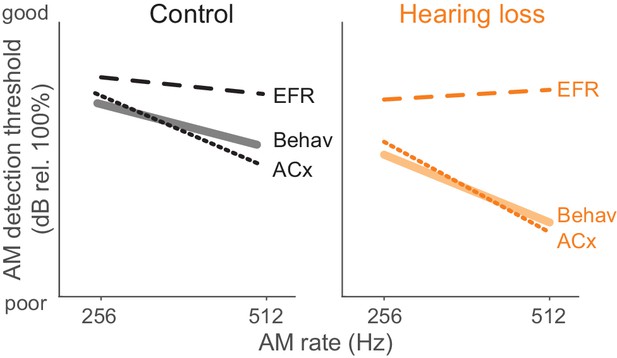
Summary of psychometric, EFR, and cortical threshold values.
Solid lines represent behavior thresholds. Dashed lines represent EFR thresholds. Dotted lines represent ACx population decoder thresholds. Ctl ACx population threshold at 256 Hz was taken as the lowest AM depth presented since decoder output d’ values did not extend below 1. Only 256 and 512 Hz conditions are shown to summarize our main findings that population ACx activity contains sufficient sensory information to explain behavioral detection of very fast AM rates and can account for the perceptual deficits displayed by HL-reared animals. The y-axis (dB rel. 100%) is the same from each of the primary data graphs from which this schematic emerges (Psychophysics: Figure 1; EFR: Figure 4; ACx: Figure 7).
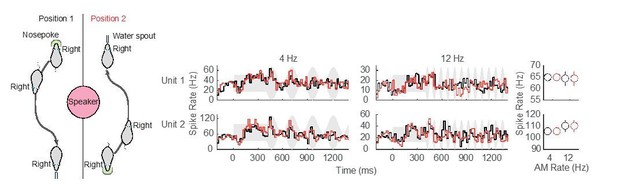
Preliminary assessment of head position.
No difference in neural responses for separate nosepoke and reward apparatus locations was observed in these two example ACx units.
Additional files
-
Transparent reporting form
- https://doi.org/10.7554/eLife.33891.012