Septal secretion of protein A in Staphylococcus aureus requires SecA and lipoteichoic acid synthesis
Abstract
Surface proteins of Staphylococcus aureus are secreted across septal membranes for assembly into the bacterial cross-wall. This localized secretion requires the YSIRK/GXXS motif signal peptide, however the mechanisms supporting precursor trafficking are not known. We show here that the signal peptide of staphylococcal protein A (SpA) is cleaved at the YSIRK/GXXS motif. A SpA signal peptide mutant defective for YSIRK/GXXS cleavage is also impaired for septal secretion and co-purifies with SecA, SecDF and LtaS. SecA depletion blocks precursor targeting to septal membranes, whereas deletion of secDF diminishes SpA secretion into the cross-wall. Depletion of LtaS blocks lipoteichoic acid synthesis and abolishes SpA precursor trafficking to septal membranes. We propose a model whereby SecA directs SpA precursors to lipoteichoic acid-rich septal membranes for YSIRK/GXXS motif cleavage and secretion into the cross-wall.
https://doi.org/10.7554/eLife.34092.001Introduction
Surface proteins of Staphylococcus aureus and other gram-positive cocci enter the secretory pathway with their N-terminal signal peptides (DeDent et al., 2008). Once translocated across the membrane, surface proteins are covalently linked to cell wall peptidoglycan via sortase A-catalyzed cleavage at the LPXTG motif of C-terminal sorting signals (Schneewind et al., 1992; Schneewind et al., 1995; Mazmanian et al., 1999). Some, but not all surface proteins are secreted at septal membranes and incorporated into cross-wall peptidoglycan (Cole and Hahn, 1962; Carlsson et al., 2006; DeDent et al., 2008). Following division and separation of spherical daughter cells, cross-wall anchored surface proteins are displayed over large segments of the bacterial surface (DeDent et al., 2007). Cross-wall trafficking of surface proteins requires a signal peptide with YSIRK/GXXS motif (Carlsson et al., 2006; DeDent et al., 2008). The YSIRK/GXXS motif is positioned N-terminal of the hydrophobic core, common to all signal peptide precursors traveling the Sec pathway (Emr et al., 1978; Emr et al., 1981; von Heijne, 1986).
Gram-positive bacteria rely on cell wall-anchored surface proteins for adherence to host tissues, evasion from host immune responses and acquisition of host-specific nutrients (Foster et al., 2014). Surface proteins with YSIRK/GXXS signal peptides are produced with high abundance and fulfill essential virulence functions during infection. For example, staphylococcal protein A (SpA) is well known for its attribute of binding to host immunoglobulin and disrupting adaptive immune responses (Forsgren and Sjöquist, 1966; Kim et al., 2016). SpA is synthesized as a precursor with an N-terminal YSIRK/GXXS signal peptide and a C-terminal LPXTG motif sorting signal (Abrahmsén et al., 1985; Schneewind et al., 1992). After initiation into the secretion pathway, the signal peptide is cleaved by signal peptidase (Abrahmsén et al., 1985; Schallenberger et al., 2012). Sortase A recognizes the LPXTG motif of the sorting signal, cleaves the polypeptide between the threonine (T) and the glycine (G) of the LPXTG motif and forms an acyl-enzyme intermediate with the C-terminal threonine (Mazmanian et al., 1999; Ton-That et al., 1999). The acyl-enzyme is resolved by the nucleophilic attack of the amino-group of the pentaglycine crossbridge within lipid II, the precursor for peptidoglycan synthesis (Ton-That et al., 2000; Perry et al., 2002). The product of this reaction, surface protein linked to lipid II, is then incorporated into peptidoglycan via the transglycosylation and transpeptidation reactions of cell wall synthesis (Ton-That et al., 1997; Ton-That and Schneewind, 1999).
Newly synthesized SpA is secreted into the cross-wall compartment, bounded by septal membranes of burgeoning cells during division (DeDent et al., 2007). Upon completion of peptidoglycan synthesis within the cross-wall, its peptidoglycan layer is split (Frankel et al., 2011). The adjacent cells separate and assume a spherical shape, resulting in SpA display on the bacterial surface (DeDent et al., 2007). Staphylococci divide perpendicular to previous cell division planes (Tzagoloff and Novick, 1977). By incorporating secreted polypeptides into newly synthesized cross-walls, staphylococci distribute SpA and other sortase A-anchored products over the bacterial surface (DeDent et al., 2008). However, not all sortase-anchored products traffic to septal membranes. Those that are secreted at polar membranes are also anchored to peptidoglycan but are not distributed over the bacterial surface (DeDent et al., 2008). In S. aureus strain Newman, thirteen different sortase-anchored surface proteins and four additional proteins are endowed with YSIRK/GXXS signal peptides for septal secretion: lipase (Lip), glycerol-ester hydrolase (Geh), murein hydrolase LytN and the cell size determinant Ebh (Yu and Götz, 2012; Frankel et al., 2011; Cheng et al., 2014).
The mechanisms supporting YSIRK/GXXS precursor secretion at septal membranes are not known. Here we show that the signal peptide of SpA is cleaved at the YSIRK/GXXS motif. Amino acid substitutions in the SpA signal peptide that affect cleavage at the YSIRK/GXXS motif also impair septal secretion. When used as bait for the isolation of the secretion machinery, SpA Ser18Leu (S18L) precursor co-purified with SecA, SecDF and LtaS. We studied the contribution of these factors towards protein A secretion into the cross-wall compartment.
Results
SpA signal peptide variants
To facilitate the analysis of signal peptide mutants, we generated SpAED, a variant of protein A that is truncated for its C-terminal immunoglobulin binding domains, region X (Xr) and the LPXTG sorting signal (Figure 1a). S. aureus WY110 (ΔspaΔsbi, pSpAED) cultures expressing spaED were fractionated into culture supernatant (S) and bacterial pellet (P) and analyzed by immunoblotting. SpAED was found in the extracellular medium; its precursor species was detected in the bacterial pellet (Figure 1bc). Site-directed mutagenesis was used to generate short deletions and amino acid substitutions in the signal peptide of SpAED (Figure 1b). Deletion of the YSIRK motif (ΔYSIRK) diminished the abundance of the SpAED/ΔYSIRK precursor and its processing (Figure 1b). Single amino acid substitutions at two positions in the YSIRK motif (I9S and R10A) resulted in precursor accumulation (Figure 1b). Further, the R10A variant exhibited diminished secretion and accumulated a precursor species that migrated faster on SDS-PAGE than the full-length precursor (Figure 1bc). Amino acid substitution at lysine 11 (K11A) of the YSIRK motif had no effect on SpAED/K11A precursor processing and secretion (Figure 1bc). Deletion of GIAS (ΔGIAS) or of the two variable residues in the GXXS motif (ΔIA) caused precursor accumulation and blocked precursor processing (Figure 1bc). Substitution of glycine 15 (G15L) reduced the abundance of SpAED/G15L and led to the accumulation of a unique precursor species that migrated faster on SDS-PAGE than full-length precursor (Figure 1b). Substitution of serine 18 (S18L) caused accumulation of full-length and processed precursors as well as reduced secretion (Figure 1bc).
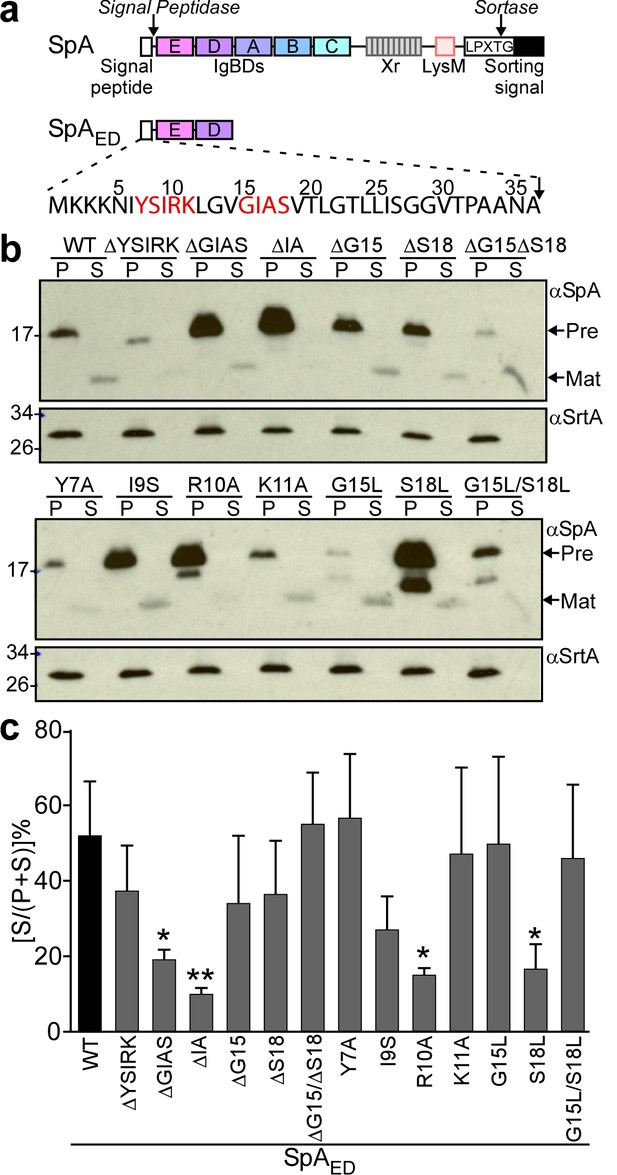
Mutagenesis of the signal peptide of staphylococcal protein A (SpA).
(a) Schematic illustrating the primary structure of SpA and of SpAED with the immunoglobulin binding domains (IgBDs, designated E, A, B, C and D), region X (Xr), LysM domain and LPXTG sorting signal. Cleavage sites for signal peptidase and sortase A are indicated. The amino acid sequence of the SpA signal peptide is displayed. YSIRK/GXXS motif residues are printed in red. (b) The structural genes for SpAED and its variants were cloned into pOS1, expressed from the spa promoter in S. aureus WY110 (∆spa ∆sbi) and secretion of SpAED was analyzed by immunoblotting with SpA-specific antibody in culture supernatant (S) and lysostaphin-digested bacterial pellet (P) samples. Signal peptide bearing SpAED precursors are labeled ‘Pre’ on the side of each blot; ‘Mat’ denotes mature protein without signal peptide. The calculated molecular weight (MW) of the variant precursors are: SpAED, 16.78 kD; SpAED/ΔYSIRK,16.13 kD; SpAED/ΔGIAS, 16.45 kD; SpAED/ΔIA, 16.59 kD; SpAED/ΔG15, 16.72 kD; SpAED/ΔS18, 16.69 kD; SpAED/ΔG15ΔS18, 16.63 kD; SpAED/Y7A, 16.68 kD; SpAED/I9S, 16.75 kD; SpAED/R10A, 16.69 kD; SpAED/K11A, 16.72 kD; SpAED/G15L, 16.83 kD; SpAED/S18L, 16.8 kD; SpAED/G15L/S18L, 16.86 kD. The MW of SpAED mature protein is 13.15 kD. Sortase A (SrtA, MW 23.54 kD) immunoblot serves as loading control. (c) Percent secretion of wild-type SpAED and its variants was quantified from triplicate experiments as the intensity of immunoblotting signals in the supernatant (S) divided by the sum signals in (S + P) fractions × 100. Statistical significance was analyzed with one-way ANOVA comparing each variant with wild-type and p values were recorded: WT vs. ∆GIAS, p=0.031; WT vs. ∆IA, p=0.0032; WT vs. R10A, p=0.0116; WT vs. S18L, p=0.0172. * denotes p<0.05, ** denotes p<0.01.
S. aureus WY110 cultures were fractionated into culture supernatant (S), cell wall extract (W), membranes (M) and cytoplasm (C). The SpAED precursor was found in the cytoplasm and membrane, whereas mature product was secreted into the culture supernatant (S) (Figure 2a). Precursors of the SpAED/ΔIA and SpAED/R10A variants accumulated mostly in the cytoplasm, whereas the SpAED/S18L precursor was located predominantly in the membrane (Figure 2ab). Pulse-labeling experiments revealed that wild-type SpAED precursor was processed within 60 s into mature, secreted product (Figure 2c). In contrast, processing of the SpAED/ΔIA, SpAED/R10A and SpAED/S18L precursors was delayed (Figure 2c). To test whether signal peptide variations affect trafficking of full-length SpA, mutations that encode the ΔIA, R10A and S18L amino acid changes were introduced into wild-type spa. Wild-type and mutant staphylococci were treated with trypsin to remove all surface proteins from the bacterial surface and incubated for 20 min to allow for cell wall deposition of newly synthesized SpA. To localize SpA, bacteria were viewed by fluorescence microscopy after labeling with SpA-specific monoclonal antibody and Alexa Fluor 647-conjugated secondary IgG (red) and with BODIPY FL-vancomycin (green), which binds to cell wall peptidoglycan. As expected, wild-type SpA was assembled in the cross-wall compartment, whereas SpASP-SasF, a protein A variant that is secreted via a canonical (non-YSIRK/GXXS) signal peptide, was deposited into peripheral segments of the cell wall envelope (DeDent et al., 2008)(Figure 2d). SpAΔIA, SpAR10A and SpAS18L exhibited defects in surface display, consistent with their observed defects in precursor processing and secretion (Figure 2d). Residual amounts of cross-wall localization were observed for SpAR10A and SpAS18L, whereas SpAΔIA was not detected in the cross-wall compartment (Figure 2e). Together these data indicate that some features of the YSIRK/GXXS motif, specifically Arg10, Ser18 and the GXXS motif, are crucial for septal secretion of SpA in S. aureus (Figure 2e).
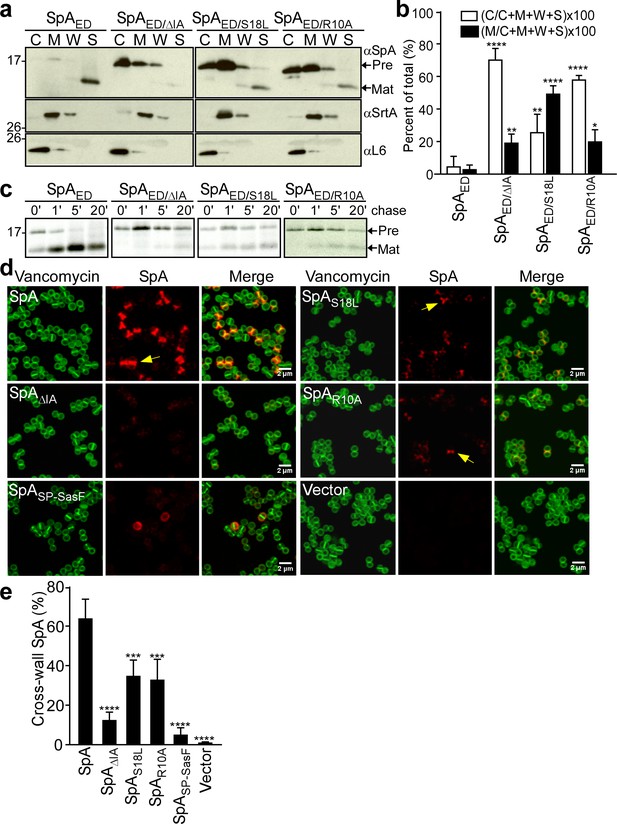
SpA signal peptide variants defective in precursor processing and septal secretion.
(a) S. aureus cultures were fractionated into cytoplasm (C), membrane (M), cell wall (W) and culture supernatant (S) and analyzed by immunoblotting with αSpA to reveal the subcellular location of wild-type SpAED precursor and secreted product of the SpAED/ΔIA, SpAED/S18L, and SpAED/R10A variants. Immunoblotting with αSrtA and αL6 was used to establish fractionation and loading controls. (b) Quantification of immunoblot signal intensity in (a) using Image J. Precursor abundance (%) the bacterial cytoplasm (C) and membrane (M) was quantified from triplicate experiments as the intensity of immunoblotting signals divided by the sum signals in all four fractions (C + M + W + S)×100. Statistical significance was analyzed with one-way ANOVA comparing each variant with wild-type and p values were recorded: for [C/(C + M + W + S)]×100, WT vs. ∆IA, p<0.0001; WT vs. S18L, p=0.0042; WT vs. R10A, p<0.0001; for [M/(C + M + W + S)]×100, WT vs. ∆IA, p=0.0056; WT vs. S18L, p<0.0001; WT vs. R10A, p=0.0405. **** denotes p<0.0001, ** denotes p<0.01, * denotes p<0.05. (c) S. aureus cultures were pulse-labeled for 60 s with [35S]methionine and labeling quenched by adding an excess of non-radioactive methionine (chase). At timed intervals during the pulse (0’) or 1 (1’), 5 (5’), and 20 (20’) minutes after the pulse (chase), culture aliquots were precipitated with trichloroacetic acid (TCA), lysostaphin-treated, immunoprecipitated with αSpA and analyzed by autoradiography. (d) S. aureus WY110 (∆spa ∆sbi) harboring chromosomal pCL55-insertions of wild-type spa (SpA), spaΔIA (SpAΔIA) spaS18L (SpAS18L), spaR10A (SpAR10A) spaSP-SasF (SpASP-SasF) or pCL55 alone (Vector) were treated with trypsin to remove SpA. Bacteria were incubated for 20 min to allow for secretion and cell wall deposition of newly synthesized SpA. Samples were incubated with BODIPY-FL vancomycin (Vancomycin) (green) to stain the bacterial cell wall and with SpA-specific monoclonal antibody and Alexa fluor 647-labeled secondary IgG (red) to reveal SpA. (e) SpA-positive staphylococci in images derived from samples in (d) were analyzed for SpA deposition at the cross wall of diplococci (n = 200). Data from three independent experiments were used to derive the mean (± SEM) and were analyzed for significant differences with one-way ANOVA for comparisons between wild-type and mutant SpA. p values were recorded: SpA vs. SpA∆IA, p<0.0001; SpA vs. SpAS18L, p=0.0006; SpA vs. SpAR10A, p=0.0004; SpA vs. SpASP-SasF, p<0.0001; SpA vs. Vector, p<0.0001. **** denotes p<0.0001, *** denotes p<0.001.
Processing of SpA signal peptide variants
Wild-type SpAED, SpAED/ΔIA, SpAED/R10A and SpAED/S18L were purified from staphylococcal membranes via affinity chromatography, analyzed by Coomassie-stained SDS-PAGE and identified by Edman degradation (Figure 3a). For wild-type SpAED, full-length precursor (SpAED-1, starting at Met1), as well as two precursors with faster mobility on SDS-PAGE (SpAED-2 and SpAED-3) and mature product (SpAED-4), that is SpsB signal peptidase-cleaved SpAED starting at Ala37, were identified (Figure 3ab). Edman degradation revealed that SpAED-2 is a product of proteolytic cleavage within the YSIRK/GXXS motif (N-terminus Gly13). SpAED-3 is a product of further cleavage, as Edman degradation identified its N-terminal amino acid 10 residues downstream (N-terminus Thr23) (Figure 3ab). Purified SpAED-1 precursor as well as its SpAED-2, SpAED-3 and SpAED-4 cleavage products were analyzed by MALDI-TOF-MS, confirming the predicted mass of the precursor and its cleaved species (Table 1). SDS-PAGE and Edman analysis of the SpAED/S18L sample revealed the same four species as wild-type SpAED, albeit that the abundance of SpAED/S18L-1 and SpAED/S18L-4 was increased over that of SpAED/S18L-2 and SpAED/S18L-3 (Figure 3ab). Analysis of the SpAED/R10A sample also identified four species, including SpAED/ R10A-1 precursor, SpAED/ R10A-3 cleavage product (N-terminus Thr23) and SpAED/ R10A-4 mature product (N-terminus Ala37), whereas SpAED/ R10A-2 represented a variant cleavage product (N-terminus Ala10) (Figure 3ab). The SpAED/ΔIA sample yielded the same precursor and cleavage species as SpAED and SpAED/S18L, however the abundance of SpAED/ΔIA-1 was increased over that of SpAED/ΔIA-2, SpAED/ΔIA-3 and SpAED/ΔIA-4 (Figure 3ab). Taken together, these data indicate that the SpA precursor (SpAED-1) is cleaved between Leu12 and Gly13, which are positioned between the two motifs (underlined) of the YSIRKL/GVGIAS sequence. The R10A substitution alters the cleavage site and diminishes precursor cleavage, whereas the S18L substitution and ΔIA deletion diminish precursor cleavage without altering the cleavage site between the YSIRK/GXXS motifs. Precursor cleavage between Gly22 and Thr23 was observed for all SpA variants, suggesting that it represents a proteolytic event unrelated to the function of the YSIRK/GXXS motif in targeting SpA secretion to septal membranes.
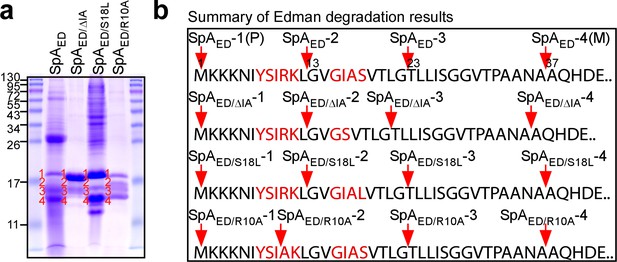
Proteolytic cleavage of the SpA signal peptide.
(a) Wild-type SpAED or SpAED/ΔIA, SpAED/S18L, and SpAED/R10A variant precursors and cleavage products were purified from detergent-solubilized staphylococcal membranes using affinity chromatography on IgG-sepharose and analyzed on Coomassie-Blue stained SDS-PAGE. Full length precursors (1) and their cleavage products (2-4) were analyzed by Edman degradation and MALDI-TOF mass spectrometry. See Table 1 for mass spectrometry data. (b) Schematic illustrating the proteolytic cleavage sites for each of the four precursors SpAED, SpAED/ΔIA, SpAED/S18L and SpAED/R10A.
MALDI-TOF-MS ion signals of purified SpAED species and their variants
https://doi.org/10.7554/eLife.34092.005Protein | Observed m/z | Calculated m/z* | ∆obs.- calc. |
---|---|---|---|
SpAED-1 | 16776.47 | 16777.7 | 1.23 |
SpAED-2 | 15273.92 | 15273.78 | 0.14 |
SpAED-3 | 14418.92 | 14418.78 | 0.14 |
SpAED-4 | 13152.40 | 13152.32 | 0.08 |
SpAED/S18L-1 | 16803.02 | 16803.78 | 0.76 |
SpAED/S18L-2 | 15298.89 | 15299.86 | 0.97 |
SpAED/S18L-3 | 14417.89 | 14418.78 | 0.89 |
SpAED/S18L-4 | 13152.22 | 13152.32 | 0.10 |
SpAED/∆IA-1 | 16592.95 | 16593.46 | 0.51 |
SpAED/∆IA-2 | 15088.71 | 15089.54 | 0.83 |
SpAED/∆IA-3 | 14417.86 | 14418.78 | 0.92 |
SpAED/∆IA-4 | 13151.55 | 13152.32 | 0.77 |
SpAED/R10A-1 | 16692.99 | 16692.59 | 0.40 |
SpAED/R10A-2 | 15584.97 | 15586.2 | 1.23 |
SpAED/R10A-3 | 14418.46 | 14418.78 | 0.32 |
SpAED/R10A-4 | 13152.07 | 13152.32 | 0.25 |
-
*Based on average mass calculated with the online ExPASy tool.
Identification of proteins that co-purify with mutant SpA precursor
We used a biochemical approach to identify staphylococcal proteins that interact with SpA precursor in septal membranes. The SpAED/S18L precursor accumulates in septal membranes (Figure 2bc) and can be purified following solubilization with detergent (Figure 3ab). After crosslinking with formaldehyde, SpAED/S18L precursor and associated species were isolated by affinity chromatography, heat-treated (95°C) to resolve crosslinks and analyzed by Coomassie-stained SDS-PAGE and immunoblotting with anti-SpA (Figure 4ab). As compared to SpAED/SP-SasF, several proteins co-purified with SpAED/S18L and were identified by mass spectrometry (Figure 4ab and Supplementary file 1). SecA was the most abundant protein. In E. coli, SecA forms a homodimer and binds signal peptide-bearing precursors for subsequent translocation through the SecYEG translocon (Grady et al., 2012; Tsirigotaki et al., 2017). In agreement with this model, SecA co-purification with SpAED/S18L, but not SpAED, from staphylococcal extracts was detected by immunoblotting both in the presence and in the absence of crosslinking agent (Figure 4c). SecA also did not co-purify with formaldehyde-treated SpAED/SP-SasF (Figure 4d). Most of the proteins crosslinked to SpAED/S18L are components of the peptidoglycan (PBP2, MurE2, MurG, FemA, FemB, FemX), wall teichoic acid (TagB, TagF) and lipoteichoic acid synthesis pathways (LtaS) that are known to be localized to septal membranes (Pinho and Errington, 2005; Mann et al., 2013; Reichmann et al., 2014). We also identified EzrA, a cell division machinery component (Steele et al., 2011), PurL, ClpB, ClpC, PknB, as well as the products of three uncharacterized genes: SAOUHSC_01854, SAOUHSC_02423, and SAOUHSC_01583. When analyzed for SpA trafficking via immunofluorescence microscopy, S. aureus mutants lacking EzrA, PBP2, PurL, ClpB, ClpC, PknB, SAOUHSC_01854, SAOUHSC_02423 (UDP-N-acetylglucosamine pyrophosphorylase), or SAOUHSC_01583 (conserved hypothetical phage protein) did not exhibit defects in septal precursor translocation (Figure 4—figure supplement 1). SecA and SecDF, members of the bacterial protein secretory pathway (Oliver and Beckwith, 1981; Gardel et al., 1987; Pogliano and Beckwith, 1994), and LtaS, lipoteichoic acid synthase (Gründling and Schneewind, 2007), were selected for further study.
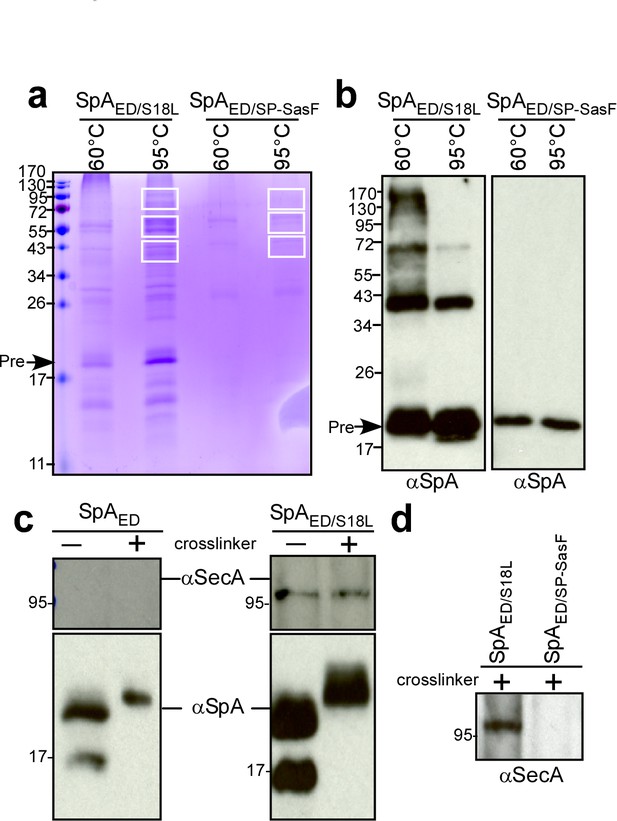
Crosslinking of staphylococcal proteins to SpAED/S18L or SpAED/SP-SasF.
(a) Bacteria from S. aureus WY110 (pSpAED/S18L) and S. aureus WY110 (pSpAED/ SP-SasF) cultures were crosslinked with 0.9% formaldehyde, membrane proteins detergent-solubilized and SpAED/S18L as well as SpAED/SP-SasF precursors purified by affinity chromatography on IgG-sepharose. Eluate was treated for 20 min at 95°C to reverse cross-linking or kept at 60°C (cross-linked control) and analyzed on Coomassie-stained SDS-PAGE. Bands were excised as indicated and individual proteins identified via ESI-MS analyses of tryptic peptides and data comparison with in silico trypsin-cleaved translation products derived from the genome sequence of S. aureus. Immunoblotting of 60 and 95°C samples to validate crosslinking of SpAED/S18L (b). The identity of the SpA-immunoreactive species migrating at 43 and 72 kDa in the left panel of Figure 4b is not known. (c) Bacteria from S. aureus WY110 (pSpAED) and S. aureus WY110 (pSpAED/S18L) cultures were treated with 0.9% formaldehyde (+crosslinker) or left untreated (- crosslinker), membrane proteins detergent-solubilized, and SpAED/S18L as well as SpAED precursors purified by affinity chromatography on IgG-sepharose. Eluate was treated for 20 min at 95°C to reverse crosslinking and samples analyzed by immunoblotting with antibodies specific for SpA and SecA (MW 95.96 kD). (d) Eluates of crosslinked SpAED/S18L and SpAED/SP-SasF precursors were examined by immunoblotting for the presence of SecA. See Supplementary file 1 for a summary of proteins crosslinked to SpAED/S18L.
SecA depletion in S. aureus
In Escherichia coli, secA is an essential gene (Oliver and Beckwith, 1981). SecA functions as an ATPase that moves many, but not all, precursor proteins across the SecYEG translocon (Tsirigotaki et al., 2017). To study the contribution of secA towards the septal secretion of SpA, we generated an inducible allele, Pspac-secA, in S. aureus WY223 (Figure 5a). When induced with isopropyl β-D-1-thiogalactoside (IPTG), S. aureus WY223 (Pspac-secA) forms colonies on agar and replicates in liquid media culture in a manner similar to wild-type S. aureus (Figure 5bc). However, in the absence of IPTG, S. aureus WY223 cannot form colonies (Figure 5c). Following dilution of bacteria from IPTG-containing media into broth without inducer, S. aureus WY223 replicates for 3 hr at a rate similar to wild-type (Figure 5b). Upon further dilution and incubation, S. aureus WY223 eventually exhibits growth retardation (6 hr time point). When analyzed by immunoblotting with SecA-specific antibody, SecA was already depleted in S. aureus WY223 (Pspac-secA) cultures 3 hr following dilution into inducer free medium (Figure 5d). After 6 hr of incubation, SecA could no longer be detected (Figure 5d). These observations indicate that depletion of SecA does not cause immediate growth retardation in S. aureus WY223; SecA-depletion likely impairs replenishment of translocated proteins that are required for staphylococcal growth, which manifests after 6 hr of SecA depletion.
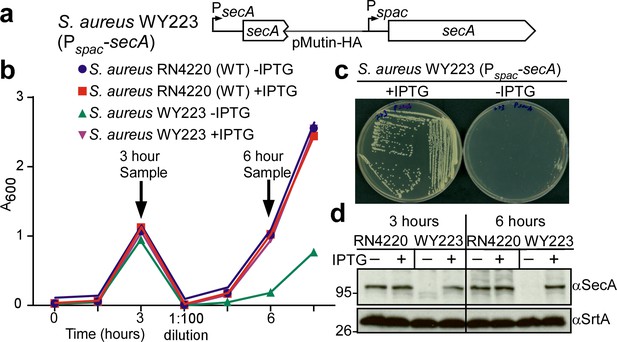
Depletion of SecA in S. aureus.
(a) Diagram of the secA gene locus in S. aureus RN4220 (wild-type parent, WT) and its Pspac-secA variant. (b) Bacteria from overnight cultures of wild-type S. aureus and S. aureus Pspac-secA grown in TSB with 1 mM IPTG were washed and suspended in fresh TSB with or without 1 mM IPTG. Subsequent growth was monitored as increased absorbance at 600 nm (A600). After three hours, cultures were diluted 1:100 into fresh TSB with or without 1 mM IPTG and incubated for additional growth measurements. (c) S. aureus Pspac-secA was streaked on tryptic soy agar with or without 1 mM IPTG supplement and incubated for 16 hr at 37°C for growth. (d) Culture samples retrieved after 3 and 6 hr in (b) were analyzed by immunoblotting with antibodies against SecA (αSecA) and sortase A (αSrtA).
SecA depletion blocks SpA secretion
After dilution into media with and without inducer, wild-type (S. aureus RN4220) and Pspac-secA (S. aureus WY223) were subjected to pulse labeling with [35S]methionine and protein A precursor processing was analyzed by immunoprecipitation. In wild-type, SpA precursors are processed within 60 s; similar rates of processing were observed when the Pspac-secA mutant was grown with IPTG inducer (Figure 6a). In the absence of IPTG, SpA precursor processing was slowed to about 5 min, indicating that SecA depletion inhibits precursor translocation (Figure 6a). When analyzed by fluorescence microcopy in trypsin-treated staphylococci incubated for 20 min without protease, wild-type S. aureus deposited protein A into the cross wall (Figure 6b, yellow arrow). Cross wall localization was diminished in Pspac-secA mutant bacteria grown without IPTG inducer and restored to wild-type levels when bacteria were grown in the presence of inducer (Figure 6c). Six hours after dilution into broth without IPTG inducer, S. aureus WY223 (Pspac-secA) cells were grossly enlarged and surrounded by a thin layer of peptidoglycan with aberrant cross-wall formation (Figure 6d, blue arrow); at this time point, SpA could not be detected in the bacterial envelope. As a control, growth of S. aureus WY223 in the presence of IPTG did not affect cell size and SpA deposition into the cell wall (Figure 6d).
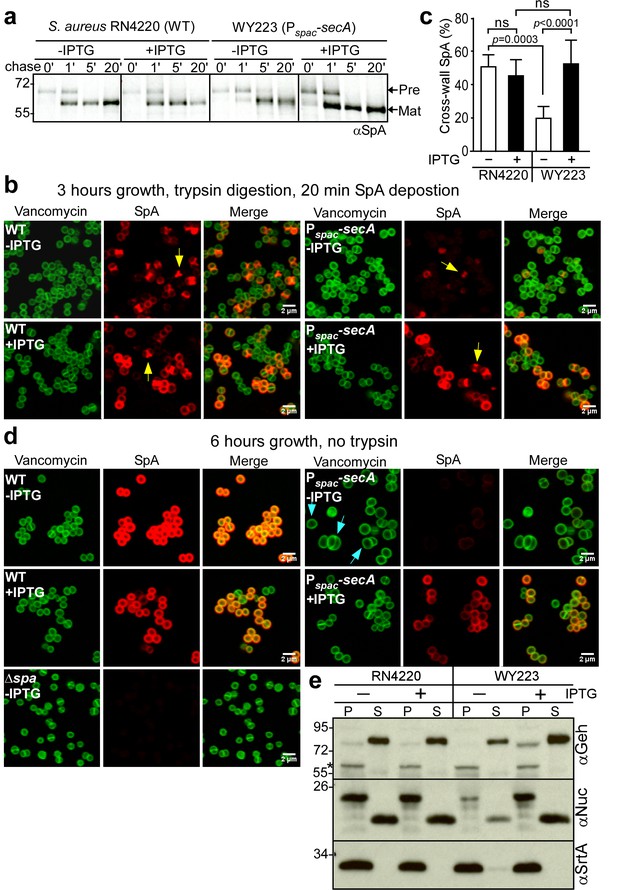
SecA depletion diminishes septal secretion of SpA in S. aureus.
(a) SpA precursor processing of [35S]methionine pulse-labeled S. aureus RN4220 (WT) or S. aureus Pspac-secA grown in the presence or absence of 1 mM IPTG. Bacteria were pulse-labeled for 60 s with radioactive methionine and then incubated with an excess of non-radioactive methionine. During the pulse (0’) or 1 (1’), 5 (5’) and 20 (20’) min after the addition of excess unlabeled methionine, culture aliquots were withdrawn, precipitated with TCA, digested with lysostaphin, and subjected to SDS-PAGE and autoradiography of immunoprecipitated SpA. Wild-type S. aureus (WT) and its Pspac-secA variant were grown for 3 (b) and 6 hr (d) in the presence or absence of 1 mM IPTG (see Figure 5). Samples in (b) were treated with trypsin to remove SpA from the bacterial surface. Bacteria were incubated for 20 min to allow for secretion and cell wall deposition of newly synthesized SpA. Samples were incubated with BODIPY-FL vancomycin (green) to stain the bacterial cell wall and with SpA-specific monoclonal antibody and Alexa Fluor 647-labeled secondary IgG (red) to reveal SpA. As a control for SpA-specific staining, the S. aureus ∆spa variant grown in the absence of IPTG was analyzed by fluorescence microscopy. (c) SpA-positive staphylococci in images derived from samples in (b) were analyzed for SpA deposition at the cross wall of diplococci (n = 200). Data from three independent experiments were used to derive the mean (± SEM) and were analyzed for significant differences with one-way ANOVA and p values recorded: RN4220-IPTG vs. RN4220 +IPTG, non-significant (ns); RN4220-IPTG vs. WY223-IPTG, p=0.0003; WY223-IPTG vs. WY223 +IPTG, p<0.0001, RN4220 +IPTG vs. WY223 +IPTG, ns. (e) SecA depletion diminishes secretion of staphylococcal proteins. Protein samples from the extracellular supernatant (S) and bacterial pellet (P) of S. aureus RN4220 (WT) and S. aureus Pspac-secA cultures grown for 3 hr in the presence or absence of 1 mM IPTG were analyzed by immunoblotting with antibodies against glycerol-ester hydrolase (αGeh) (precursor MW 76.39 kD, mature protein MW 72.26 kD), nuclease (αNuc) (precursor MW 25.12 kD, mature protein MW 18.78 kD) and sortase A (αSrtA). *Identifies unknown proteins crossreactive with αGeh.
We wondered whether SecA depletion affects the secretion of other staphylococcal proteins. Glycerol-ester hydrolase (Geh) is synthesized as a precursor with YSIRK/GXXS signal peptide motif (Lee and Iandolo, 1986). Following secretion at septal membranes into the cross-wall compartment, Geh is subsequently released into the extracellular medium (Yu and Götz, 2012). When analyzed by immunoblotting of proteins in the extracellular medium, depletion of SecA in S. aureus WY223 (Pspac-secA) caused a reduction in secreted Geh, as compared to wild-type staphylococci or S. aureus WY223 grown in the presence of IPTG (Figure 6e). Staphylococcal nuclease (Nuc), a secreted protein that contributes to the pathogenesis of human and animal infections, is synthesized as a precursor with a canonical signal peptide (Phonimdaeng et al., 1990; Shortle, 1983). The abundance of secreted Nuc was also diminished in SecA-depleted cultures of S. aureus WY223 (Figure 6e). As a control, production of sortase A in staphylococcal membranes was not affected by the depletion of SecA. Taken together, these data indicate that SecA is essential for S. aureus growth and for the secretion of precursors with canonical and YSIRK/GXXS signal peptides.
Localization of SecA and SpA precursors in staphylococci
To localize SecA within S. aureus, we generated a translational hybrid between secA and the structural gene for super-folder green fluorescent protein (gfp) (Pédelacq et al., 2006) under transcriptional control of the Ptet promoter in S. aureus WY230 (Pspac-secA, Ptet-secA:sfGFP, Figure 7a). Expression of secA:sfGFP in the Pspac-secA variant restored bacterial growth in the absence of IPTG inducer, indicating that secA-gfp is functional (Figure 7b). Growth restoration occurred in the presence and in the absence of anhydrotetracycline (ATc), suggesting that secA:sfGFP must be expressed even in the absence of the Ptet inducer (Figure 7b). Immunoblotting of staphylococcal cell extracts 3 and 6 hr following dilution into media lacking IPTG revealed that S. aureus WY230 indeed produced small amounts SecA:sfGFP in the absence of ATc (Figure 7c). In the presence of ATc inducer, the abundance of SecA-GFP was increased (Figure 7c). As expected, wild-type SecA was depleted when S. aureus WY230 was cultured for 3 or 6 hr without the IPTG inducer (Figure 7b). However, under SecA depleting conditions, even small amounts of SecA-sfGFP in S. aureus WY230 (-ATc) restored precursor processing of pulse-labeled SpA (Figure 7d). SpA precursor processing was accelerated to levels faster than wild-type when S. aureus WY230 cultures were grown in the presence of ATc (Figure 7d). Fluorescence microscopy of S. aureus WY230 stained with the membrane dye FM4-64 (red) revealed SecA-sfGFP localization to plasma membranes (Figure 7e). In dividing cells, SecA-sfGFP was found on septal and on polar membranes (Figure 7e). ATc-induced overexpression of SecA-sfGFP caused accumulation of hybrid protein throughout plasma membranes (Figure 7e). Thus, in S. aureus WY230, SecA-sfGFP is associated with all membranes and is not restricted to septal membranes.
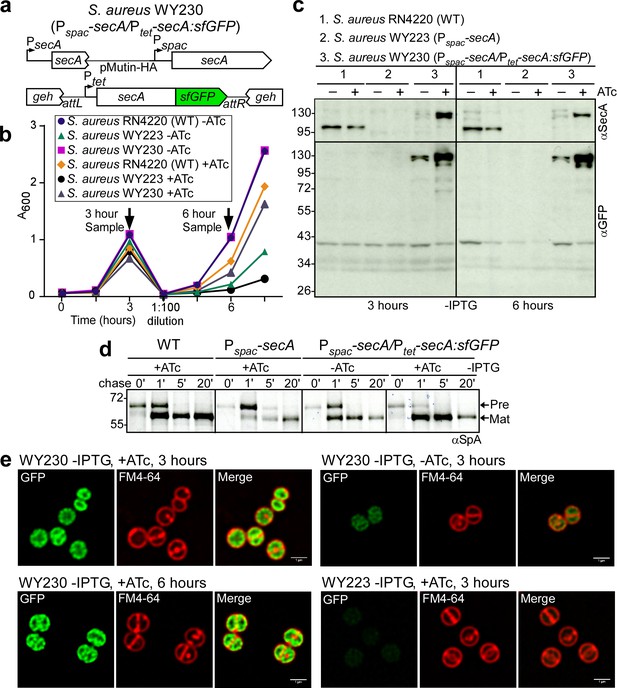
SecA localization in staphylococci.
(a) Diagram of the secA gene locus and of the pCL55-mediated att insertion site for secA-sfGFP in the staphylococcal genome. (b) Bacteria from overnight cultures of S. aureus RN4220 (WT), S. aureus Pspac-secA (WY223) and S. aureus Pspac-secA/Ptet-secA:sfGFP (WY230) grown in TSB with 1 mM IPTG were washed and suspended in fresh TSB without IPTG and with or without 0.43 µM anhydro-tetracycline (ATc); growth was monitored as increased absorbance at 600 nm (A600). After three hours, cultures were diluted 1:100 into fresh TSB without IPTG and with or without 0.43 µM ATc and incubated for further growth measurements. (c) Culture samples retrieved after 3 and 6 hr from the experiment detailed in (b) were analyzed by immunoblotting with rabbit antibodies against SecA (αSecA) and sfGFP (αGFP). (d) [35S]methionine-labeled S. aureus cultures incubated for 3 hr as described in (b) were analyzed during the 60 s pulse with radioactive methionine (0) and 1, 5 and 20 min after the addition of excess unlabeled methionine via SDS-PAGE and autoradiography of immunoprecipitated SpA. (e) Fluorescence microscopy of bacteria from S. aureus cultures incubated for 3 and 6 hr as described in (b). Bacteria were stained with the membrane dye FM4-64 (red) and analyzed for SecA-sfGFP fluorescence (green). Scale bar, 1 µm.
The envelope of trypsin-treated, paraformaldehyde-fixed S. aureus WY223 (Pspac-secA) was permeabilized with murein hydrolase and with detergent to detect intracellular precursors via microscopy with fluorescent antibody (Harry et al., 1995; Pinho and Errington, 2003). In S. aureus WY223 producing wild-type levels of SecA (Pspac-secA +IPTG), SpA precursors were localized to septal membranes (Figure 8a). In other images, SpA precursors appeared as two puncta or ring deposits at septal membranes, reminiscent of FtsZ and of the division rings that are known to accumulate at this site (Figure 8ab) (Lutkenhaus, 1993). In contrast, under SecA depleting conditions (-IPTG), SpA precursors in S. aureus WY223 were associated with polar membranes and were not localized to septal membranes (Figure 8a). These results suggest that in staphylococci with a functional secretion pathway, SpA precursor are localized to the vicinity of septal division rings. However, in cells lacking functional secretion machines, SpA precursors are located throughout the cytoplasm and cannot traffic to septal membranes.
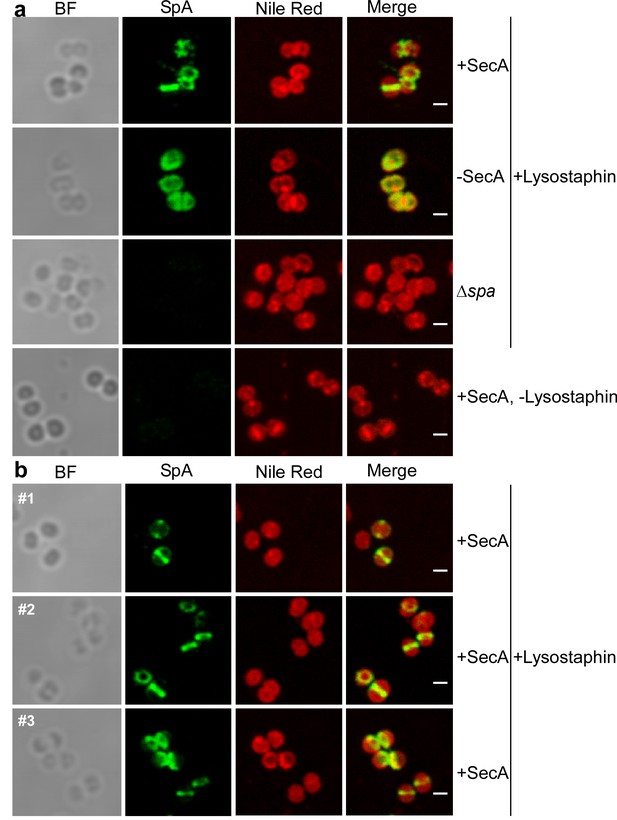
Intracellular trafficking of SpA in the presence and absence of SecA.
(a) S. aureus Pspac-secA cells were grown for 3 hr in the presence (+SecA) or absence of 1 mM IPTG (-SecA) and, alongside S. aureus ∆spa control cells, were trypsin treated to remove extracellular surface proteins and fixed with para-formaldehyde. Samples were then treated with lysostaphin (+Lysostaphin) or left untreated (-Lysostaphin), incubated with detergent and SpA-specific rabbit antibodies and Alexa Fluor 488-labeled goat-anti-rabbit-IgG (green) and with Nile red to reveal bacterial membranes. Bright-field microscopy (BF) images were acquired to reveal the contours of all bacterial cells. Scale bar, 1 µm. (b) Additional samples (#1, #2 and #3) of S. aureus Pspac-secA cells were grown in the presence of 1 mM IPTG (+SecA), trypsin treated, fixed with para-formaldehyde, lysostaphin treated, incubated with detergent and with SpA-specific antibody (green) and Nile red.
SecDF contributes to SpA secretion
The secDF gene is not essential for protein secretion and S. aureus growth, however secDF mutants exhibit diminished secretion of many precursors secreted via canonical and YSIRK-GXXS signal peptides (Quiblier et al., 2011; Quiblier et al., 2013). SecDF is a member of the resistance nodulation and cell division (RND) membrane protein family with 12-transmembrane spanning segments. SecDF functions as a membrane-integrated chaperone. Sustained by the proton motive force, SecDF catalyzes ATP-independent translocation and folding of proteins on the trans-side of the plasma membrane (Tsukazaki et al., 2011). S. aureus expresses two additional RND proteins, here designated Rnd2 (SAOUHSC_02525) and Rnd3 (SAOUHSC_02866) (Quiblier et al., 2011). The rnd2 gene is located downstream of femX, whose product tethers glycine from glycyl-tRNA to the ε-amino group of lysine in lipid II peptidoglycan precursor [C55-(PO4)2-MurNAc(L-Ala-D-iGln-L-Lys-Da-Ala-D-Ala)-GlcNac] (Rohrer et al., 1999). Rnd2 product interacts with FemB, PBP1 and PBP2 (Quiblier et al., 2011). As SecDF, FemB and PBP2 were each found crosslinked to SpAED/S18L precursors (Supplementary file 1), we asked whether secDF, rnd2 and rnd3 contribute to septal secretion of SpA. Compared to wild-type S. aureus, the ΔsecDF mutant (S. aureus WY418) accumulated SpAED precursor in bacterial cells and secreted reduced amounts of mature SpAED into the extracellular medium (Figure 9ab). S. aureus rnd2 (WY416) and rnd3 (WY400) mutants exhibited wild-type levels of SpAED secretion (Figure 9ab). A variant (WY412) lacking all three genes, ΔsecDF Δrnd23, accumulated precursors at a level similar to the ΔsecDF mutant (Figure 9ab). When analyzed for other secreted proteins, the ΔsecDF mutant secreted diminished amounts of Geh and failed to secrete Coa, whose precursor is secreted via a canonical signal peptide (Phonimdaeng et al., 1990), while the Δrnd2 and Δrnd3 variants displayed wild-type phenotypes (Figure 9ab). Thus, SecDF, but not Rnd2 and Rnd3, contributes to protein secretion in S. aureus. Immunofluorescence microscopy experiments revealed that septal secretion of SpA was diminished in the ∆secDF and ΔsecDF Δrnd23 mutants (Figure 9c). Unlike SecA-depleted cells, where SpA precursors failed to associate with septal membranes, ∆secDF and ΔsecDF Δrnd23 mutants exhibited puncta of SpA precursors and rings of low fluorescent intensity at septal membranes (Figure 9c). The diminished abundance of SpA precursors at septal membranes was restored to wild-type levels by plasmid-borne expression of secDF in ∆secDF (pSecDF) and in ΔsecDF Δrnd23 (pSecDF) staphylococci (Figure 9c). These data suggest that SecDF aids in the translocation of SpA across staphylococcal membranes but is not absolutely required for precursor targeting to septal membranes.
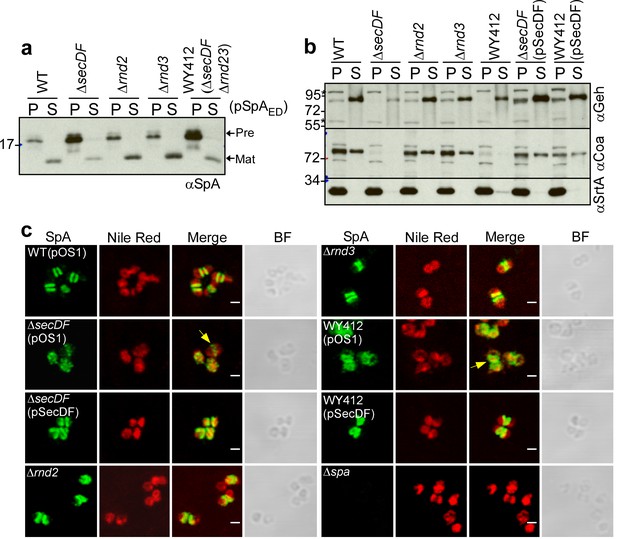
SecDF contributes to SpA secretion at septal membranes.
(a) S. aureus cultures were centrifuged to sediment the bacteria into the pellet (P) fraction and separate them from the extracellular medium (S, supernatant). Following lysostaphin digestion of bacteria, proteins in both fractions were precipitated with TCA and analyzed by immunoblotting with αSpA. (b) S. aureus cultures were fractionated as described in (a) and subjected to immunoblotting with antibodies specific for glycerol-ester hydrolase (αGeh), coagulase (αCoa) (precursor MW 71.72 kD, mature protein MW 68.96 kD) and sortase A (αSrtA). (c) Fluorescence microscopy of bacteria from cultures of S. aureus RN4220 (WT, wild-type), WY418 (∆secDF), WY416 (∆rnd2), WY400 (∆rnd3) and WY412 (∆secDF ∆rnd23) mutants with and without expression plasmid for wild-type secDF (pSecDF) as well as S. aureus SEJ1 (∆spa) as control. Bacteria were trypsin treated to remove extracellular surface proteins and fixed with para-formaldehyde. Samples were treated with lysostaphin, incubated with detergent and SpA-specific rabbit antibodies and Alexa Fluor 488-labeled goat-anti-rabbit-IgG (green) and with Nile red to reveal bacterial membranes. BF identifies the bright-field microscopy view of fluorescence microscopy images. Scale bar, 1 µm.
LtaS is required for septal localization of SpA
LtaS-mediated synthesis of lipoteichoic acid, a polyglycerol-phosphate polymer decorated with esterified D-alanyl and GlcNAc residues, is essential for S. aureus growth and cell division (Gründling and Schneewind, 2007). Earlier work generated S. aureus Pspac-ltaS, a strain with IPTG-inducible expression of lipoteichoic acid synthase (Figure 10a). In the absence of IPTG inducer, LtaS is depleted in S. aureus ANG499 (Pspac-ltaS), providing an experimental system to study the effects of LTA synthesis on septal secretion of SpA (Gründling and Schneewind, 2007). Surface proteins were removed with trypsin and staphylococci were incubated for 20 min to localize deposition of newly synthesized SpA (Figure 10bc). LtaS depletion (-IPTG) resulted in SpA deposition into polar peptidoglycan, whereas under LtaS inducing conditions (+IPTG) SpA was localized in the cross-wall (Figure 10bc). We asked whether SpA precursors are mislocalized to polar membranes under conditions of LtaS depletion. Microscopic analysis of trypsin-treated, lysostaphin- and detergent-permeabilized staphylococci revealed SpA targeting to septal membranes in wild-type (S. aureus RN4220) and in IPTG-induced S. aureus ANG499 (Pspac-ltaS)(Figure 10d). In contrast, without IPTG inducer, S. aureus ANG499 mislocalized SpA precursors to polar membranes (Figure 10d). Protein secretion and cell wall anchoring of SpA were analyzed by immunoblotting in S. aureus cultures separated into culture supernatant (S) and bacterial sediment (P, pellet) samples. These experiments revealed that LtaS depletion in S. aureus ANG499 (Pspac-ltaS, -IPTG) diminished the abundance of cell wall anchored SpA without affecting the secretion of Geh and Nuc (Figure 10e). Consistent with the immunoblotting results, LtaS depletion diminished the overall surface distribution of SpA (Figure 10f), in agreement with the hypothesis that cross-wall targeting via the YSIRK/GXXS signal peptide, but not polar secretion, is responsible for efficient surface distribution of proteins in staphylococci (Carlsson et al., 2006; DeDent et al., 2008). Together these data indicate that LtaS depletion and a block in lipoteichoic acid synthesis abolished SpA precursor trafficking to septal membranes without affecting its secretion at polar membranes.
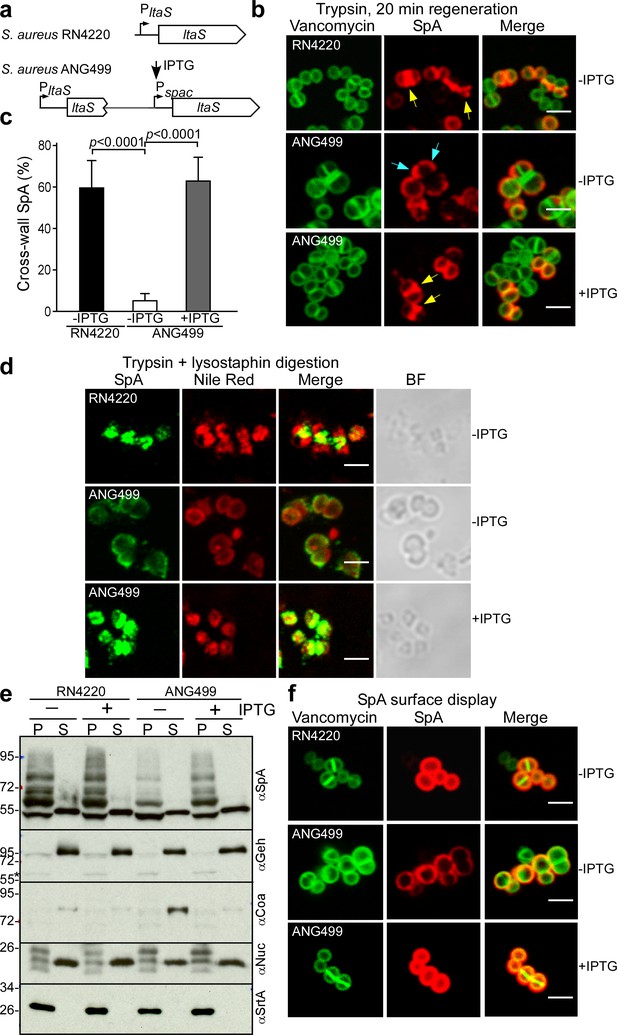
Localization of SpA secretion in LtaS-depleted S. aureus.
(a) Schematic to illustrate the ltaS locus in S. aureus RN4220 and ANG499. (b) Fluorescence microscopy with BODIPY-FL-vancomycin (green) and αSpA (red) stained samples 20 min after trypsin removal of surface proteins from the staphylococcal envelope to detect newly synthesized SpA. Scale bar, 2 µm. (c) SpA-positive staphylococci in images derived from samples in (b) were analyzed for SpA deposition at the cross wall of diplococci (n = 200). Data from three independent experiments were used to derive the mean (± SEM) and were analyzed for significant differences with one-way ANOVA for comparisons between S. aureus RN4220 (WT) and ANG499 grown with (+LtaS) and without IPTG (-LtaS). p values were recorded: RN4220-IPTG vs. ANG499-IPTG, p<0.0001; ANG499-IPTG vs. ANG499 +IPTG, p<0.0001. (d) Fluorescence microscopy to localize intracellular SpA in S. aureus strains RN4220 (WT) and ANG499 (Pspac-ltaS) grown with and without IPTG induction for 3 hr. Bacteria were trypsin treated to remove extracellular surface proteins and fixed with para-formaldehyde. Samples were then treated with lysostaphin, incubated with detergent and SpA-specific rabbit antibodies and Alexa Fluor 488-labeled goat-anti-rabbit-IgG (green) and with Nile red to reveal bacterial membranes. BF identifies the bright-field microscopy view of fluorescence microscopy images. Scale bar, 2 µm. (e) The culture supernatant (S) and bacterial pellet (P) samples of S. aureus RN4220 and ANG499 grown for three hours in the presence or absence of IPTG were immunoblotted with antibodies specific for SpA (αSpA), glycerol-ester hydrolase (αGeh), coagulase (αCoa), nuclease (αNuc) and sortase A (αSrtA). (f) Fluorescence microscopy of staphylococci to measure surface display of protein A in bacteria stained with BODIPY-FL-vancomycin (green) and αSpA (red) without trypsin treatment. Scale bar, 2 µm.
Discussion
Cell wall-anchored surface proteins with YSIRK/GXXS motif signal peptides have been identified in streptococcal and staphylococcal species (Tettelin et al., 2005; Rosenstein and Götz, 2000). Although sortase-anchored surface proteins are found in many different gram-positive bacteria, the signal peptides of surface proteins in the genus Actinomyces, Bacillus, Clostridium, and Listeria do not contain the YSIRK/GXXS motif. Common features of staphylococci and streptococci are their spherical or ovoid cell shapes and cell wall synthesis programs at septal membranes; in staphylococci this compartment is designated as the cross-wall (Giesbrecht et al., 1976; Touhami et al., 2004; Monteiro et al., 2015). Earlier work demonstrated that the YSIRK/GXXS motif of the SpA precursor is dispensable for sortase-catalyzed cell wall anchoring (Bae and Schneewind, 2003). However, precursors with YSIRK/GXXS motif signal peptides are targeted for secretion at septal membranes and sortase-mediated deposition into the cross wall compartment (Carlsson et al., 2006; DeDent et al., 2008). After completion of cross-wall synthesis, peptidoglycan splitting and cell separation, the anchored products of spa and of other genes with YSIRK/GXXS motif signal peptides are distributed over the bacterial surface (Cole and Hahn, 1962; DeDent et al., 2007). In contrast, surface proteins with canonical signal peptides are deposited by sortase into polar peptidoglycan but cannot be distributed over bacterial surfaces (Carlsson et al., 2006; DeDent et al., 2008).
Although it is clear that YSIRK/GXXS signal peptides are necessary and sufficient for septal secretion of proteins, the mechanisms supporting such trafficking were heretofore not known. We show that the YSIRK/GXXS signal peptide of SpA is cleaved between Leu12 and Gly13, separating the YSIRK sequence from the GXXS motif and from the remainder of the signal peptide. Amino acid substitutions and deletions that affect precursor cleavage and secretion map to three of the four residues (underlined) that are strictly conserved in the YSIRK/GXXS motif: Ile9, Arg10 and Ser18. Substitution of the fourth residue, Gly15, with Leu resulted in diminished abundance of precursor substrate and secreted product and in accumulation of a cleaved precursor species (Figure 1b). On the basis of these observations, we are compelled to speculate that YSIRK/GXXS motif cleavage may represent a mechanism for precursor translocation at septal membranes. For example, the YSIRK/GXXS motif may inhibit a key function of the adjacent hydrophobic core within signal peptides: promoting the membrane translocation of precursors. Such inhibitory mechanism could be relieved by a YSIRK/GXXS protease that localizes to the septal membrane. Other mechanisms of proteolytic control for YSIRK/GXXS mediated signal peptide function can also be thought of. Importantly, the discovery of two sequential proteolytic events, YSIRK/GXXS motif cleavage and the signal peptidase-mediated cut provide experimental opportunities for the testing of predictive models. SpA precursors were also cut between Gly22 and Thr23, a site that is located within the hydrophobic core of the signal peptide. Mass spectrometry analysis of the S. aureus COL secretome also identified signal peptide fragments that had been generated by cleavage in the hydrophobic core, including SpA signal peptides cleaved between Gly22 and Thr23 (Ravipaty and Reilly, 2010). The significance of signal peptide cleavage in the hydrophobic core is not known, as amino acid substitutions preventing such proteolysis have not be studied for their effect on protein secretion or membrane integrity. We presume that cleavage at Gly22/Thr23 may not be related to septal secretion. Cleavage at the hydrophobic core may enable staphylococci to remove the products of processed signal peptides from the membrane. For example, products of degradative proteolysis have been observed during processing of SpA LPXTG motif sorting signal, which is cleaved between Thr (T) and Gly (G) and within the hydrophobic core (Navarre and Schneewind, 1994).
Precursors with amino acid substitutions or deletions in the YSIRK/GXXS motif are thought to accumulate in septal membranes, however these variants typically exhibit diminished secretion and cell wall anchoring in the cross wall (DeDent et al., 2008; Yu and Götz, 2012). Our observations corroborate these findings and suggest that not all features of the YSIRK/GXXS motif are required for precursor targeting to septal membranes. We took advantage of the SpAED/S18L precursor and used affinity chromatography to purify crosslinked proteins. Several crosslinked proteins were already known to be located in septal membranes (PBP2, EzrA, LtaS), consistent with SpAED/S18L precursor accumulation in this compartment. Among the crosslinked proteins are two components of the secretion machinery, SecA and SecDF, as well as LtaS, which catalyzes the synthesis of lipoteichoic acid in septal membranes (Tsirigotaki et al., 2017; Percy and Gründling, 2014). SecA appears to bind directly to the signal peptide of SpAED/S18L. The resulting complex may even engage SecYEG translocase as well as SecDF. However, due to the S18L substitution SpAED/S18L precursors cannot efficiently enter the septal secretion pathway. It seems unlikely that LtaS may directly interact with SpAED/S18L. We think it is plausible that SpAED/S18L complexed with SecA and SecYEG at septal membranes can be crosslinked to other septal proteins.
The subcellular localization of the Sec apparatus has been examined in streptococci, enterococci and in Bacillus subtilis. A spiral pattern of Sec translocase has been reported for B. subtilis (Campo et al., 2004). In S. pyogenes, contradictory results have been reported for immunogold-labelling and electron microscopy experiments: SecA was localized to a single microdomain and also found distributed throughout the plasma membrane (Carlsson et al., 2006; Rosch and Caparon, 2004). In S. pneumoniae, SecA localization changed during cell cycle progression. In early divisional cells, SecA was predominantly localized to septal membranes, whereas during later stages of division SecA was hemispherically distributed within the region between septa and at the future equators of dividing cells (Tsui et al., 2011). Streptococcus agalactiae SecA was localized to septal membranes, whereas SecA was detected as a single microdomain in Streptococcus mutans and Enterococcus faecalis (Brega et al., 2013; Hu et al., 2008; Kline et al., 2009). We show here that S. aureus SecA is localized to the plasma membrane and is not spatially restricted to septal membranes or microdomains. This distribution is consistent with our proposed role of SecA, promoting precursor translocation at polar and septal membranes.
When studied for its contribution to septal secretion, SecDF chaperone allows large amounts of protein A to be deposited into the cross-wall peptidoglycan and promotes secretion of YSIRK/GXXS motif precursors (SpA and Geh). Nevertheless, secDF is not essential for septal targeting or secretion of SpA precursors. In contrast, cells depleted for SecA, accumulate SpA precursors that cannot traffic to septal membranes in the cytoplasm. Finally, LtaS-depleted staphylococci are unable to synthesize lipoteichoic acid and cannot direct precursors to the septal area. Instead, SpA is directed to polar membranes. We have incorporated these observations into a model whereby septal accumulation of LtaS and of lipoteichoic acids functions as a determinant for SecA-mediated targeting of SpA precursors. Following precursor cleavage at the YSIRK/GXXS motif, truncated SpA (SpA-2) is moved across the membrane, aided by the proton-motif force and by the chaperone activity of SecDF. Once translocated, SpA is cleaved by signal peptidase to generate SpA-4 and by sortase at the LPXTG motif of its C-terminal sorting signal (Navarre and Schneewind, 1994; Ton-That et al., 1999). The resulting sortase-acyl intermediate is then incorporated into cross wall peptidoglycan for distribution on the bacterial surface (Schneewind et al., 1995).
Enterococcus faecalis Eep, a membrane zinc-metalloprotease, cleaves signal peptides of lipoprotein precursors into short hydrophobic peptides that are subsequently dislodged from the membrane by an ABC transporter and function as pheromones to induce the transfer of conjugative plasmids by specific donors (An et al., 1999; Chandler and Dunny, 2008; Varahan et al., 2014). The corresponding zinc-metalloprotease in S. aureus is designated RseP (SAOUHSC_01239). However, RseP is not involved in septal secretion and cleavage of the YSIRK-GXXS signal peptides, as a deletion of the corresponding gene does not affect SpA septal secretion in the S. aureusΔSAOUHSC_01239 mutant (Figure 4—figure supplement 1).
Materials and methods
Bacterial strains and growth conditions
Request a detailed protocolE. coli strains were grown in Luria-Bertani broth (LB) or LB agar. S. aureus strains were grown in tryptic soy broth (TSB) or agar (TSA). Ampicillin (100 µg/ml) was used for plasmid selection in E. coli. Chloramphenicol was used for selection of pOS1 derivatives (10 µg/ml) and pCL55 derivatives (5 µg/ml) in S. aureus (Lee et al., 1991). Erythromycin (Erm 10 µg/ml) was used for selection of ermB marked bursa aurealis transposon mutants in S. aureus WY110 (∆spa ∆sbi) and 10 µg/ml Erm plus 1 mM isopropyl β-D-1-thiogalactopyranoside (IPTG) was used for pMutin–HA-5’secA selection in S. aureus. Expression from the Pspac promoter was induced with 1 mM IPTG. Anydrotetracycline (ATc, 200 ng/ml) was used to induce expression from the tetracycline-inducible promoter in pCL55-Ptet constructs.
Plasmids and strains
Request a detailed protocolTo avoid mutations in the spa gene, all cloning procedures were performed at 30°C. All pOS1-derivative and pCL55-derivative plasmids were constructed in E. coli DC10B (Monk et al., 2012) and transformed to S. aureus strains by electroporation (Schneewind and Missiakas, 2014). All plasmids and strain constructs were validated by DNA sequencing (Supplementary file 2). Primers used in this study are listed in Supplementary file 3. To avoid cross reaction in SpA immunoblot and purification, S. aureus WY110 (∆spa ∆sbi) was generated by transducing the sbi::ermB allele from the Phoenix library (Bae et al., 2004) into S. aureus SEJ1, that is S. aureus RN4220 carrying ∆spa. Phage transduction was performed as described previously (Schneewind and Missiakas, 2014). To construct pSpAED, primers 10 and 69 were used to amplify the spa promoter and spaED coding sequence encoding IgBDs E and D (−40 bp upstream of the transcription start site of spa to 459 bp of spa coding sequence) from chromosomal DNA of S. aureus RN4220. The PCR product was digested with EcoRI and BamHI, and ligated with plasmid pOS1 (Schneewind et al., 1993). To generate mutations and deletions within the SpA signal peptide sequence, quick-change mutagenesis was performed as follows: primers pairs (Supplementary file 3) that contain desired mutation or deletion were used to PCR amplify pSpAED. The PCR products were digested with DpnI and transformed to E. coli DC10B. Plasmid variants confirmed by DNA sequencing were transformed into S. aureus WY110. To construct pCL55-SpA and its derivatives, primer pairs 175 and 177 were used to PCR amplify the spa promoter and full-length spa coding sequence. PCR products were digested with BamHI and KpnI and ligated into pCL55 cut with the same enzymes (Lee et al., 1991). The resulting plasmid, pCL55-SpA, was used as template for PCR mutagenesis of its signal peptide mutant derivatives via quick-change mutagenesis as described above. To construct pCL55-SpASP-SasF, primer pairs 175 and 21 were used to amplify the promoter sequence of spa. Primers 22 and 23 were used to amplify coding sequence for the signal peptide sequence of sasF. Last, primers 24 and 177 were used amplify spa coding sequence for the E and D IgBDs. All three DNA fragments were ligated via SOE (splicing by overlap extension) PCR, digested with BamHI and KpnI, and then ligated into pCL55 cut with the same restriction enzymes. pCL55-derivaties were transformed into S. aureus WY110 and integrated into the chromosome at the geh locus (Lee et al., 1991). The integration was confirmed by PCR. To construct the secA depletion strain S. aureus WY223 (Pspac-secA), primers 189 and 190 were used to amplify the ribosome binding site and the first 656 bp of the secA gene. The PCR product was digested with HindIII and KpnI and ligated with pMutin–HA (Bacillus Genetic Stock Center, Columbus, OH). The resulting plasmid pMutin–HA-5’secA was transformed into RN4220 and integrated at the secA locus in the chromosome. Clones were selected on TSA supplemented with 10 µg/ml erythromycin and 1 mM IPTG. To construct pCL55-Ptet-secA:sfGFP, primers 180 and 181 were used to amplify secA full-length coding sequence together with its ribosome binding site. Primers 182 and 183 were used to amplify sfGFP gene from pCX-sfGFP (Yu and Götz, 2012). The two DNA fragments were joined together by SOE. The resulting secA:sfGFP hybrid, which contains the ‘Gly-Gly-Ala-Ala-Gly-Ala’ between SecA and sfGFP, was digested with AvrII-BglII and ligated with pCL55-Ptet (Gründling and Schneewind, 2007). pCL55-Ptet-secA:sfGFP was transformed to into S. aureus WY223 (Pspac-secA) and integrated into the chromosome at the geh locus, thereby generating S. aureus WY230 (Pspac-secA, Ptet-secA:sfGFP). Plasmid pKOR1 based allelic replacement (Bae and Schneewind, 2006) was used to generate the ∆secDF (S. aureus WY418) and ∆rnd2 (S. aureus WY416) knock-out mutants. S. aureus WY412, a mutant with ∆secDF ∆rnd23 mutations, was generated by transducing the rnd3::ermB allele from the Phoenix library into S. aureus carrying ∆secDF and ∆rnd2 mutations. To construct the complementation plasmid pSecDF, the secDF ORF and 274 bp upstream sequence were PCR amplified with primers 315 and 316, digested with EcoRI and BamHI, and ligated into pOS1 cut with the same enzymes. The resulting plasmid, pSecDF, was transformed into S. aureus strains WY418 (∆secDF) and WY412 (∆secDF ∆rnd23).
Cell fractionation and immunoblotting
Request a detailed protocolBacterial overnight cultures were diluted 1: 100 into fresh TSB and grown to OD600 0.8. One ml culture was centrifuged at 18,000 × g for 5 min in an Eppendorf tube. The culture supernatant (S) was transferred to another tube and proteins were precipitated with 10% trichloroacetic acid (TCA) on ice for 30 min. The bacterial sediment (P, pellet) was suspended in 1 ml Tris-buffer [50 mM Tris-HCl (pH 7.5), 150 mM NaCl] and incubated with 20 µg/ml lysostaphin at 37°C for 30 min. After cell lysis, proteins from the cell pellet were precipitated with 10% TCA. To localize proteins in different cellular compartments, cell fractionation was performed as follows: 1 ml culture (OD600 = 0.8) was centrifuged at 18,000 × g for 5 min in an Eppendorf tube. The supernatant was transferred to another tube and proteins were precipitated with 10% TCA (S, supernatant). The pellet was suspended in 1 ml TSM [50 mM Tris-HCl (pH 7.5), 0.5 M sucrose, 10 mM MgCl2] and incubated with 20 µg/ml lysostaphin for 10 min at 37°C. After centrifugation at 18,000 × g for 5 min, the supernatant (cell wall fraction) was transferred to another tube. The protoplast pellet was suspended in 1 ml Tris-buffer [50 mM Tris-HCl (pH 7.5), 10 mM MgCl2] and subjected to three freeze-thaw cycles in dry ice/ethanol and warm water baths. Membranes in cell lysates were sedimented by ultracentrifugation 150,000 × g for 40 min. Supernatant was transferred to another tube (cytosolic fraction), whereas the pellet (membrane fraction) was suspended in 1 ml Tris-buffer and precipitated with 10% TCA. After TCA precipitation on ice for 30 min, proteins were sedimented at 18,000 × g for 10 min, washed with ice-cold acetone, air-dried and solubilized in 100 μl 1 × SDS sample buffer [62.5 mM Tris-HCl (pH 6.8), 2% SDS, 10% glycerol, 5% 2-mercaptoethanol, 0.01% bromophenol blue]. For immunoblotting, protein samples were separated on 10, 12 or 15% SDS-PAGE and transferred to polyvinylidene difluoride (PVDF) membranes. Membranes were blocked with 5% milk for 45 min. As needed, 50 μl human IgG (Sigma) was added to 10 ml block-solution to block SpA cross-reaction. Primary antibodies were affinity-purified rabbit polyclonal antibodies against SpAKKAA (1:10,000 dilution), rabbit serum of anti-SrtA (1:20,000 dilution), rabbit serum of anti-SecA (1:10,000 dilution), rabbit serum of anti-Geh (1:10,000 dilution), polyclonal antibodies of anti-Coa (1:5000 dilution), rabbit serum of anti-Nuc (1:5000 dilution), and anti-GFP rabbit serum (1:10,000 dilution) (Invitrogen). Membranes were incubated with primary antibodies for 1 hr, washed three times for 5 min with TBST [50 mM Tris-HCl (pH 7.5), 150 mM NaCl, 0.1% Tween 20], incubated with secondary anti-rabbit IgG linked to horseradish peroxidase (HRP) for 1 hr, washed, and developed using enhanced chemiluminescence substrates. The intensity of immunoblot signals was analyzed and measured with Image J software (Schneider et al., 2012). Statistical analysis was performed using GraphPad Prism software. One-way ANOVA (Dunnett's multiple comparisons test) was used to compare the mean for each variant with the mean for SpAED wild-type (Figures 1c and 2b).
Pulse-labeling
Request a detailed protocolStaphylococcal cultures were grown to mid-log phase (OD600 0.8) in TSB and bacteria sedimented by centrifugation at 18,000 × g for 5 min. Bacterial pellets were washed twice and suspended in 1 ml minimal medium 4. [35S]methionine/cysteine (100 µl = 100 µCi Perkin Elmer) was added to bacterial suspensions, vortexed and incubated for 60 s at 37°C. 250 µl was removed and immediately mixed with 250 µl ice-cold 10% TCA to quench all metabolic activity (time 0’). Chase solution (50 µl of 2 mg/ml methionine, 2 mg/ml cysteine and 10 mg/ml casamino acids) was added to the remainder of bacterial suspension and incubated for 1, 5 and 20 min. At each time point, 250 µl bacterial suspension was removed and mixed with 250 µl ice-cold 10% TCA. TCA precipitated cells were washed with acetone, dried and suspended in 1 ml 0.5 M Tris-HCl (pH 7.0) containing 20 µg/ml lysostaphin. After lysostaphin treatment at 37°C for 1 hr, cell lysate was precipitated with 7% TCA, washed with acetone, dried, suspended in 50 µl 4% SDS, 0.5 M Tris-HCl (pH 7.5) and allowed to incubate for 30 min prior to boiling. Subsequently, samples were incubated for 1 hr with rabbit polyclonal anti-SpAKKAA antibody (Kim et al., 2010) that was 1:1000 diluted in 1 ml RIPA buffer (0.1% SDS, 0.5% deoxycholic acid, 1% Triton X-100, 50 mM Tris-HCl pH 8.0, 150 mM NaCl). Protein A sepharose (50 µl of 50% slurry, Sigma) was added to each sample and incubated for 1 hr followed by five washes with 1 ml RIPA buffer. Proteins bound to the beads were solubilized by boiling in 15 µl 2 × SDS sample buffer for 10 min and separated on 10% (SpA) or 15% SDS-PAGE (SpAED). Gels were dried on Whatman 3 M paper and autoradiographed on X-ray film for 48 hr or longer.
Purification of SpAED/S18L, Edman degradation and MALDI-TOF mass spectrometry
Request a detailed protocolOvernight bacterial cultures of S. aureus WY110 (pSpAED or its derivatives) were diluted 1:100 into 4 liters of TSB and grown to OD600 2. Staphylococci were sedimented by centrifugation at 8,000 × g for 10 min. Bacteria were suspended in 30 ml of Tris-buffer, 0.5 (vol/vol) 0.1 mm sterilized glass beads were added and peptidoglycan was broken with 15 × 1 min pulses in a bead-beating instrument (MP Biomedicals). Samples were centrifuged at 7,000 × g for 10 min to sediment glass beads. The supernatant was transferred to another tube and centrifuged at 33,000 × g for 1 hr at 4°C. The membrane sediment was suspended in 30 ml RIPA buffer and incubated for 1 hr with rotation. RIPA extract was centrifuged at 33,000 × g for 1 hr at 4°C. The supernatant was removed and subjected to affinity chromatography. Two ml 50% suspension of IgG sepharose (GE Healthcare) was loaded onto each column. The column bed was washed once with 7 ml 0.1 M glycine (pH 3.0), twice with 14 ml 50 mM Tris-HCl (pH 7. 5) and once with 10 ml RIPA buffer. RIPA membrane extracts were loaded onto the column followed by two washes with 14 ml RIPA buffer and once with 10 ml 50 mM Tris-HCl (pH 7. 5). Proteins were eluted by adding four times 1 ml 0.1 M glycine (pH 3.0) to the column and immediately neutralizing the eluate with 25 µl of 1.5 M Tris (pH 8.8). For Edman degradation, the purified SpAED precursors were 10-fold concentrated via Amicon Ultra-0.5 ml Centrifugal Filters (10 kD cut off). Proteins were separated on 15% SDS-PAGE, electro-transferred to PVDF and stained with Coomassie-Brilliant Blue. Bands of interest were excised and subjected to Edman degradation (Alphalyse, Inc, CA, USA). For MALDI-TOF mass spectrometry analysis, 1 µl of SpAED sample was mixed with 1 µl of 10 mg/ml sinapic acid, dried on the Bruker MTP 384 plate, and examined in a Bruker Autoflex Speed MALDI-TOF mass spectrometer in the linear positive-ion mode using peptide standards for calibration.
Crosslinking of SpAED precursor
Request a detailed protocolOvernight cultures of S. aureus WY110 (∆spa ∆sbi, pSpAED/S18L) and S. aureus WY110 (∆spa ∆sbi, pSpAED/SP-SasF) were each diluted 1: 100 into 4 L TSB and grown to OD600 2. Formaldehyde (0.9%, methanol free) was added to the bacterial culture and incubated for 20 min with shaking. Cross-linking was quenched by adding 400 ml ice-cold 0.125 M glycine and rotating the sample for 10 min. Staphylococci were sedimented by centrifugation at 8,000 × g for 10 min. Bacteria were suspended in 30 ml of 50 mM Tris-HCl (pH 7.5), 150 mM NaCl, and washed twice in the same buffer. Sterilized 0.1 mm glass beads 0.5 (vol/vol) were added and peptidoglycan broken with 15 × 1 min pulses in a bead-beating instrument (MP Biomedicals). Samples were centrifuged at 7000 × g for 10 min to sediment glass beads. The supernatant was transferred to another tube and centrifuged at 33,000 × g for 1 hr at 4°C. The membrane sediment was suspended in 30 ml 50 mM Tris-HCl (pH 7. 5), 2% n-dodecyl β-D-maltoside (DDM) and incubated at 4°C overnight. Samples were subjected to ultracentrifugation at 150,000 × g for 40 min. The supernatant was subjected to affinity chromatography on IgG sepharose affinity purification as described above. Eluate was concentrated via Amicon Ultra-0.5 ml 10 kD Centrifugal Filters and mixed with equal volume of 2 × SDS sample buffer. To reverse the cross-linking, samples were either boiled at 95°C for 20 min or, as a control, incubated at 60°C (no reversal of cross-linking). Proteins in all samples were separated on 12% SDS-PAGE and bands of interest excised as indicated in Figure 4 and subjected to protein identification and semi-quantitative analysis at the Harvard University Taplin Mass Spectrometry Facility (Supplementary file 1).
SecA depletion and SecA-sfGFP induction
Request a detailed protocolOvernight cultures of S. aureus RN4220 (WT), S. aureus WY223 (Pspac-secA) and S. aureus WY230 (Pspac-secA, Ptet-secA:sfGFP) were grown in TSB and 1 mM IPTG. Overnight cultures were washed twice with an equal volume of TSB and diluted 1:100 into fresh TSB with or without 1 mM IPTG and with or without 200 ng/ml ATc. After 3 hr growth at 37°C, cultures were diluted into fresh TSB with or without IPTG or ATc and subjected to further growth at 37°C. Growth was monitored by sampling cultures at timed intervals and measuring optical density. One ml bacterial culture was removed after 3 hr (prior to the second 1:100 dilution) and after 6 hr (3 hr after the second 1:100 dilution). Samples were processed for protein secretion and immunoblotting assays or analyzed by fluorescence microscopy. A similar procedure was performed for LtaS depletion using S. aureus ANG499 (Pspac-ltaS). Samples from the LtaS depletion experiments were analyzed after 3 hr growth with or without 1 mM IPTG.
Fluorescence microscopy
View detailed protocolTo observe SpA targeting to the cross-wall, 2 ml mid-log phase S. aureus culture (OD600 0.8) were centrifuged at 18,000 × g for 5 min, supernatant removed, bacteria washed once in 2 ml PBS and suspended in 1 ml PBS containing 0.5 mg/ml trypsin (Sigma). After incubation at 37°C for 1 hr, staphylococci were washed twice with PBS, suspended in fresh TSB containing 2.5 mg/ml soybean trypsin inhibitor (Sigma) and incubated at 37°C for 20 min with rotation. 250 µl of the cell suspension was removed and immediately mixed with fixation solution (2.5% paraformaldehyde and 0.006% glutaraldehyde in PBS). The cells were fixed for 20 min at room temperature, washed three times with PBS and applied to poly-L-lysine coated 8-well glass slides (MP Biomedicals) for 5 min. Excess and non-adherent cells were washed away with PBS. Immobilized cells were blocked with 3% BSA in PBS for 45 min and incubated with SpA-specific mouse hybridoma monoclonal antibody 5A10 (Kim et al., 2010) (diluted 1:4000 in 3% BSA) for 1 hr. Cells were washed eight times with PBS and further incubated in the dark with Alexa Fluor 647 conjugated anti-mouse IgG (1:500 in 3% BSA) (Invitrogen, Carlsbad, CA) for 1 hr. Cells were washed 10 times with PBS and incubated with 1 µg/ml BODIPY-FL vancomycin (ThermoFisher) for 10 min in the dark followed by washing five times with PBS. A drop of SlowFade Gold reagent (Molecular Probes) was applied to samples before sealing coverslips with nail polish. Fluorescent images were visualized and captured on a Leica SP5 Tandem Scanner Spectral 2-Photon Confocal microscope with 100 × oil objective. Identical settings and exposure times were applied to all samples.
To image SpA display on the staphylococcal surface, 1 ml of mid-log phase S. aureus cultures were centrifuged at 18,000 × g for 5 min and supernatant removed. Bacteria were washed once in 2 ml PBS and suspended in 1 ml PBS and mixed with fixation solution. Cells were fixed for 20 min at room temperature, washed three times with PBS and applied to poly-L-lysine coated 8-well glass slides (MP Biomedicals) for 5 min, stained with vancomycin and αSpA antibodies and analyzed by fluorescence microscopy.
To localize intracellular SpA, 2 ml of mid-log phase staphylococcal cultures were centrifuged at 18,000 × g for 5 min and supernatant removed. Bacteria were washed once in 2 ml PBS and suspended in 1 ml PBS, 0.5 mg/ml trypsin (Sigma). After incubation at 37°C for 1 hr, staphylococcal cells were washed twice with PBS and fixed with fixation solution. The cells were fixed for 15 min at room temperature and 30 min on ice, washed three times with PBS and suspended in 1 ml GTE buffer [50 mM glucose, 20 mM Tris-HCl (pH 7.5), 10 mM EDTA]. Lysostaphin (10 µg/ml) was added and 50 µl cell suspensions were immediately applied to poly-L-lysine coated 8-well glass slides and incubated for 1 min. Non-adherent cells were removed and PBS, 0.2% Triton X-100 was applied to samples for 10 s. Excessive liquid was aspirated and slides were air-dried. Dried slides were immediately dipped in methanol at −20°C for 5 min, and in acetone at −20°C for 30 s and then allowed to dry completely. Afterwards, the cells on the slides were re-hydrated with PBS for 5 min, blocked with 3% BSA, stained with the membrane dye Nile red (Sigma) and rabbit antibodies specific for SpA followed by Alexa-Fluor-488 conjugated goat-anti-rabbit-IgG and analyzed by fluorescence microscopy as described above.
To visualize the sub-cellular localization of SecA-sfGFP, samples from 3 and 6 hr growth cultures were removed as described above. Bacteria were sedimented by centrifugation and washed twice in PBS. Cells were stained with 1 µg/µl FM4-64FX (Molecular Probes) for 10 min in the dark and were then fixed with fixation solution for 20 min. After washing twice with PBS, cells were applied to poly-L-lysine coated coverslips, and incubated for 5 min. After removing excess bacterial cells, SlowFade Gold reagent was added to the samples and the coverslips were sealed onto glass slides. Samples were visualized under Leica SP8 3D, 3-color Stimulated Emission Depletion (STED) laser scanning confocal microscope with 100×/1.45 oil objective. Images were captured with identical settings. Deconvolution images with identical parameters were generated by using a HyVolution module installed on the microscope.
All the images were analyzed in Image J software (Schneider et al., 2012). To quantify the frequency of SpA cross-wall localization at 20 min regeneration after trypsin digestion, numbers of diplococci and numbers of cross-wall localized SpA were counted manually using the cell counter tool in Image J. Diplococci were defined as two daughter cells that had divided and formed a cross-wall but had not yet separated. Cross-wall localized SpA signals were defined as lines at the cross-wall of diplococci. Diplococci were counted in vancomycin stained images and cross-wall localized SpA was counted in merged images. The frequency was determined by dividing cross-wall localized SpA by the number of diplococci. An example of the counting method is displayed in the Source file to Figure 2e. At least two random images were acquired per sample for each experiment. Three or more independent experiments were performed and data from more than 200 diplococci were analyzed for statistically significant differences using one-way ANOVA with Dunnett's multiple comparison test comparing staphylococci expressing wild-type and spa variants (Figure 2e). In Figure 6c and Figure 10c the Tukey's multiple comparison test was used to analyze differences between multiple groups.
Data availability
All data generated or analysed during this study are included in the manuscript and supporting files. Source data files have been provided for Figure 2.
References
-
Analysis of signals for secretion in the staphylococcal protein A geneThe EMBO Journal 4:3901–3906.
-
Identification and characterization of a determinant (eep) on the Enterococcus faecalis chromosome that is involved in production of the peptide sex pheromone cAD1Journal of Bacteriology 181:5915–5921.
-
Subcellular sites for bacterial protein exportMolecular Microbiology 53:1583–1599.https://doi.org/10.1111/j.1365-2958.2004.04278.x
-
The giant protein Ebh is a determinant of Staphylococcus aureus cell size and complement resistanceJournal of Bacteriology 196:971–981.https://doi.org/10.1128/JB.01366-13
-
Distribution of protein A on the surface of Staphylococcus aureusJournal of Bacteriology 189:4473–4484.https://doi.org/10.1128/JB.00227-07
-
"Protein A" from S. aureus. I. Pseudo-immune reaction with human gamma-globulinJournal of Immunology 97:822–827.
-
Adhesion, invasion and evasion: the many functions of the surface proteins of Staphylococcus aureusNature Reviews Microbiology 12:49–62.https://doi.org/10.1038/nrmicro3161
-
LytN, a murein hydrolase in the cross-wall compartment of Staphylococcus aureus, is involved in proper bacterial growth and envelope assemblyJournal of Biological Chemistry 286:32593–32605.https://doi.org/10.1074/jbc.M111.258863
-
secD, a new gene involved in protein export in Escherichia coliJournal of Bacteriology 169:1286–1290.https://doi.org/10.1128/jb.169.3.1286-1290.1987
-
On the morphogenesis of the cell wall of staphylococciInternational Review of Cytology 44:225–318.https://doi.org/10.1016/S0074-7696(08)61651-4
-
Characterization of the Escherichia coli SecA signal peptide-binding siteJournal of Bacteriology 194:307–316.https://doi.org/10.1128/JB.06150-11
-
Nontoxigenic protein A vaccine for methicillin-resistant Staphylococcus aureus infections in miceThe Journal of Experimental Medicine 207:1863–1870.https://doi.org/10.1084/jem.20092514
-
FtsZ ring in bacterial cytokinesisMolecular Microbiology 9:403–409.https://doi.org/10.1111/j.1365-2958.1993.tb01701.x
-
Cell shape dynamics during the staphylococcal cell cycleNature Communications 6:8055.https://doi.org/10.1038/ncomms9055
-
Lipoteichoic acid synthesis and function in gram-positive bacteriaAnnual Review of Microbiology 68:81–100.https://doi.org/10.1146/annurev-micro-091213-112949
-
Engineering and characterization of a superfolder green fluorescent proteinNature Biotechnology 24:79–88.https://doi.org/10.1038/nbt1172
-
SecD and SecF facilitate protein export in Escherichia coliThe EMBO Journal 13:554–561.
-
Type I signal peptidase and protein secretion in Staphylococcus aureusJournal of Bacteriology 194:2677–2686.https://doi.org/10.1128/JB.00064-12
-
Cell wall sorting signals in surface proteins of gram-positive bacteriaThe EMBO journal 12:4803–4811.
-
Genetic manipulation of Staphylococcus aureusCurrent protocols in microbiology 32:Unit 9C.3..https://doi.org/10.1002/9780471729259.mc09c03s32
-
NIH Image to ImageJ: 25 years of image analysisNature Methods 9:671–675.https://doi.org/10.1038/nmeth.2089
-
Multiple essential roles for EzrA in cell division of Staphylococcus aureusMolecular Microbiology 80:542–555.https://doi.org/10.1111/j.1365-2958.2011.07591.x
-
Anchor structure of staphylococcal surface proteins. A branched peptide that links the carboxyl terminus of proteins to the cell wallThe Journal of Biological Chemistry 272:22285–22292.https://doi.org/10.1074/jbc.272.35.22285
-
Anchor structure of staphylococcal surface proteins. IV. inhibitors of the cell wall sorting reactionThe Journal of Biological Chemistry 274:24316–24320.https://doi.org/10.1074/jbc.274.34.24316
-
Atomic force microscopy of cell growth and division in Staphylococcus aureusJournal of Bacteriology 186:3286–3295.https://doi.org/10.1128/JB.186.11.3286-3295.2004
-
Protein export through the bacterial Sec pathwayNature Reviews Microbiology 15:21–36.https://doi.org/10.1038/nrmicro.2016.161
-
Geometry of cell division in Staphylococcus aureusJournal of Bacteriology 129:343–350.
-
Towards a comparative anatomy of N-terminal topogenic protein sequencesJournal of Molecular Biology 189:239–242.https://doi.org/10.1016/0022-2836(86)90394-3
Article and author information
Author details
Funding
National Institute of Allergy and Infectious Diseases (AI038897)
- Olaf Schneewind
Deutsche Forschungsgemeinschaft (YU 181/1-1)
- Wenqi Yu
National Institute of Allergy and Infectious Diseases (AI052474)
- Olaf Schneewind
The funders had no role in study design, data collection and interpretation, or the decision to submit the work for publication.
Acknowledgements
We thank Vytas Bindokas (Microscopy Core Facility, University of Chicago) for assistance with microscopy and members of our laboratory for experimental advice and discussion. This work was supported by National Institute of Allergy and Infectious Diseases grants AI038897 and AI052474. WY acknowledges support from German Research Foundation (DFG) Fellowship (award YU 181/1–1).
Version history
- Received: December 5, 2017
- Accepted: May 5, 2018
- Accepted Manuscript published: May 14, 2018 (version 1)
- Version of Record published: May 21, 2018 (version 2)
Copyright
© 2018, Yu et al.
This article is distributed under the terms of the Creative Commons Attribution License, which permits unrestricted use and redistribution provided that the original author and source are credited.
Metrics
-
- 3,288
- views
-
- 412
- downloads
-
- 22
- citations
Views, downloads and citations are aggregated across all versions of this paper published by eLife.
Download links
Downloads (link to download the article as PDF)
Open citations (links to open the citations from this article in various online reference manager services)
Cite this article (links to download the citations from this article in formats compatible with various reference manager tools)
Further reading
-
- Microbiology and Infectious Disease
In the Firmicutes phylum, GpsB is a membrane associated protein that coordinates peptidoglycan synthesis with cell growth and division. Although GpsB has been studied in several bacteria, the structure, function, and interactome of Staphylococcus aureus GpsB is largely uncharacterized. To address this knowledge gap, we solved the crystal structure of the N-terminal domain of S. aureus GpsB, which adopts an atypical, asymmetric dimer, and demonstrates major conformational flexibility that can be mapped to a hinge region formed by a three-residue insertion exclusive to Staphylococci. When this three-residue insertion is excised, its thermal stability increases, and the mutant no longer produces a previously reported lethal phenotype when overexpressed in Bacillus subtilis. In S. aureus, we show that these hinge mutants are less functional and speculate that the conformational flexibility imparted by the hinge region may serve as a dynamic switch to fine-tune the function of the GpsB complex and/or to promote interaction with its various partners. Furthermore, we provide the first biochemical, biophysical, and crystallographic evidence that the N-terminal domain of GpsB binds not only PBP4, but also FtsZ, through a conserved recognition motif located on their C-termini, thus coupling peptidoglycan synthesis to cell division. Taken together, the unique structure of S. aureus GpsB and its direct interaction with FtsZ/PBP4 provide deeper insight into the central role of GpsB in S. aureus cell division.
-
- Microbiology and Infectious Disease
The agr quorum-sensing system links Staphylococcus aureus metabolism to virulence, in part by increasing bacterial survival during exposure to lethal concentrations of H2O2, a crucial host defense against S. aureus. We now report that protection by agr surprisingly extends beyond post-exponential growth to the exit from stationary phase when the agr system is no longer turned on. Thus, agr can be considered a constitutive protective factor. Deletion of agr resulted in decreased ATP levels and growth, despite increased rates of respiration or fermentation at appropriate oxygen tensions, suggesting that Δagr cells undergo a shift towards a hyperactive metabolic state in response to diminished metabolic efficiency. As expected from increased respiratory gene expression, reactive oxygen species (ROS) accumulated more in the agr mutant than in wild-type cells, thereby explaining elevated susceptibility of Δagr strains to lethal H2O2 doses. Increased survival of wild-type agr cells during H2O2 exposure required sodA, which detoxifies superoxide. Additionally, pretreatment of S. aureus with respiration-reducing menadione protected Δagr cells from killing by H2O2. Thus, genetic deletion and pharmacologic experiments indicate that agr helps control endogenous ROS, thereby providing resilience against exogenous ROS. The long-lived ‘memory’ of agr-mediated protection, which is uncoupled from agr activation kinetics, increased hematogenous dissemination to certain tissues during sepsis in ROS-producing, wild-type mice but not ROS-deficient (Cybb−/−) mice. These results demonstrate the importance of protection that anticipates impending ROS-mediated immune attack. The ubiquity of quorum sensing suggests that it protects many bacterial species from oxidative damage.