A twist defect mechanism for ATP-dependent translocation of nucleosomal DNA
Figures
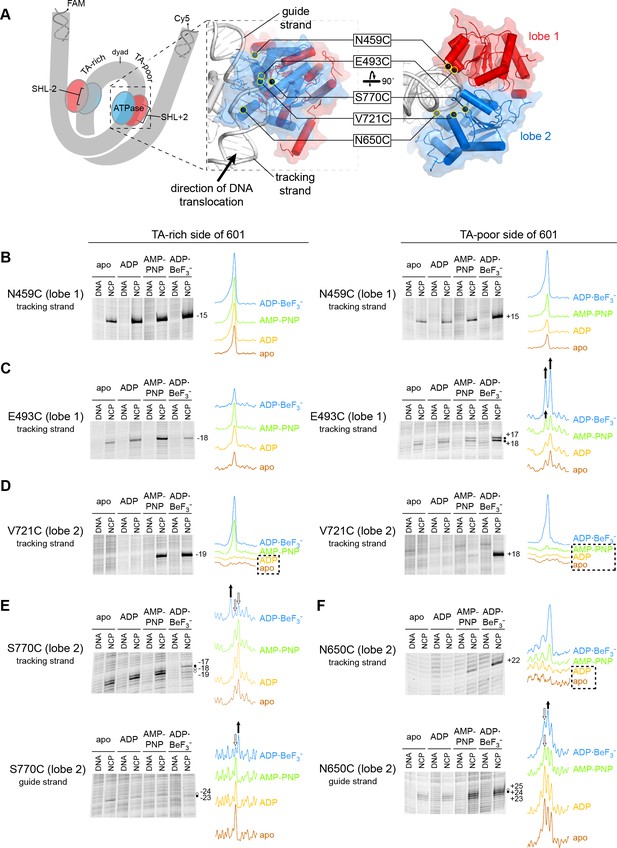
Interactions between the ATPase motor of Chd1 and nucleosomal DNA are sensitive to both nucleotide state and DNA sequence.
(A) Overview of the Chd1 ATPase motor on the nucleosome. A cartoon schematic (left) shows the superhelical wrap of nucleosomal DNA, with an ATPase motor (blue/red) at each of the superhelix location (SHL) 2 sites on either side of the nucleosome. The expanded views on the right show the molecular surface of the ATPase motor in complex with nucleosomal DNA, based on a cryoEM complex (Farnung et al., 2017). The five positions individually substituted with cysteine for APB cross-linking are highlighted with yellow circles, and the tracking and guide strands of DNA are indicated. The PyMOL visualization package (RRID:SCR_000305) was used for molecular rendering. (B-F). Representative data showing cross-linking to nucleosomal DNA on either the TA-rich side (left) or TA-poor side (right) of the 601 sequence, in different nucleotide conditions. Identical cross-linking reactions were performed with 300-450 nM Chd1 variant with either 150 nM canonical 601 nucleosomes with 40 bp of flanking DNA on each side ('NCP') or 150 nM naked DNA ('DNA'), to ensure observed cross-links were nucleosome-specific. Bands that increase or decrease in cross-linking efficiency for ADP·BeF3– compared to other conditions are indicated with black or white circles, respectively. Scans of each lane are shown as intensity plots to the right of each gel, with arrows highlighting bands that showed relative increases (black) or decreases (white) for ADP·BeF3– conditions. Dotted boxes highlight nucleotide conditions where cross-links were severely reduced or not detected. Numbering refers to the distances (nt) of cross-linked sites from the 601 dyad (positive numbers for TA-poor side and negative numbers for TA-rich side). These data are representative of 4 or more experiments. Extended gel images are shown in Figure 1—figure supplement 3.
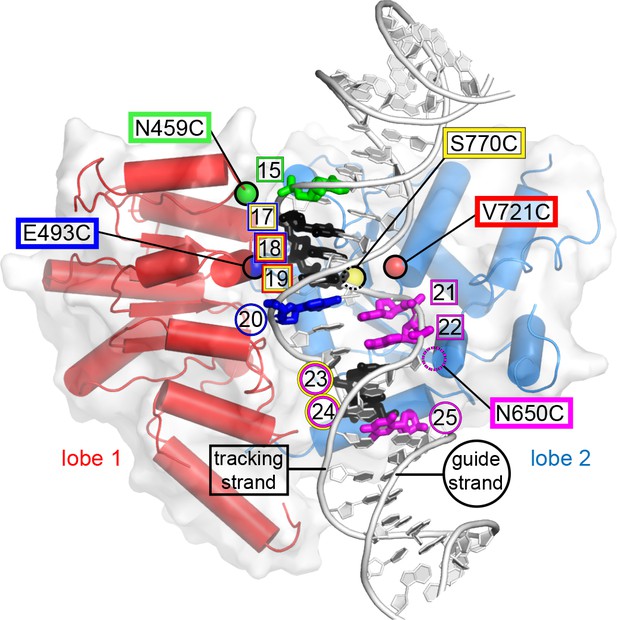
Site-specific cross-linking visualized on the Chd1-nucleosome structure.
This image shows the ATPase motor of Chd1 bound to nucleosomal DNA at SHL2 in the cryoEM structure 5O9G (Farnung et al., 2017), captured in an ADP·BeF3– state. Each residue used for site-specific cross-linking is highlighted with a colored sphere at the Cα position. Residue N650 was not built in the cryoEM structure (dotted magenta circle), and its position was therefore estimated based on superimposing the Chd1 crystal structure 3MWY (Hauk et al., 2010). DNA nucleotides are colored according to cross-links from each cysteine-substituted site. Nucleotides cross-linked by multiple sites are colored black, with colored circles (guide strand) or squares (tracking strand) indicating the origin of cross-linking. Cross-linking sites shown here represents a compilation of both TA-rich and TA-poor sides of the Widom 601 nucleosome. The PyMOL visualization package (RRID:SCR_000305) was used for molecular rendering.

DNA sequences of 601 variants used in this study.
Sequence information for the core 145 bp of the canonical and variant 601 positioning sequences used in this study (5’ to 3’). Periodic TA steps are indicated with gray dotted boxes, and colored segments highlight sequence elements that are altered in 601 variants, corresponding to the cartoons in Figure 4. The red box indicates the inserted A/T bp for the variant 601[insert(+1) TA-rich]. These core segments were made into double-labeled Cy5/FAM 40N40 nucleosomes using primers that matched flanking DNA in the original Widom 601 plasmid: 5’-/Cy5/AGTTCATCCCTTATGTGATGGACCCTATAC on the TA-rich (left) side, and 5’-/6FAM/ATCCGACTGGCACCGGCAAGGTCGCTGTTC on the TA-poor (right) side.
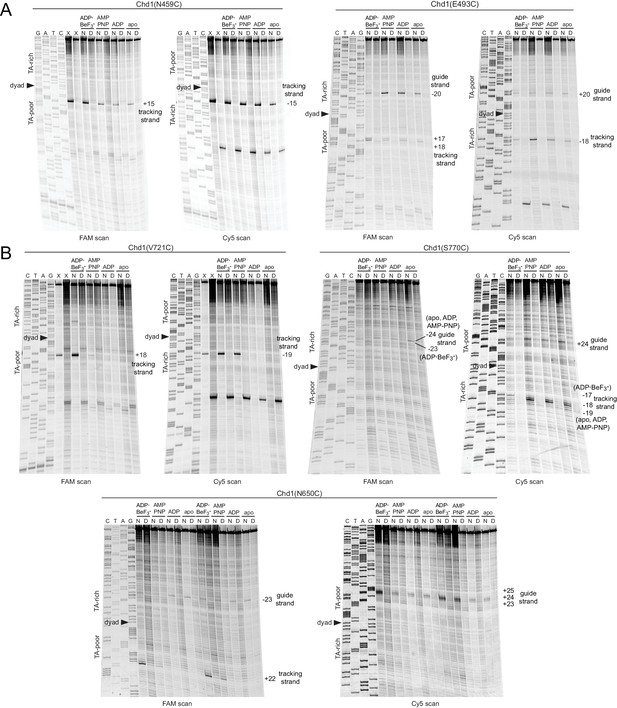
Extended gel images of Chd1 cross-linking reactions.
Scans of urea denaturing gels, shown in Figure 1, for cross-linking reactions performed with cysteine substitutions in ATPase lobe 1 (A) or lobe 2 (B). Each reaction was carried out with either 40N40 nucleosomes (N) or naked DNA (D) with the canonical Widom 601 sequence. Numbering refers to the distance (nt) from the 601 dyad.
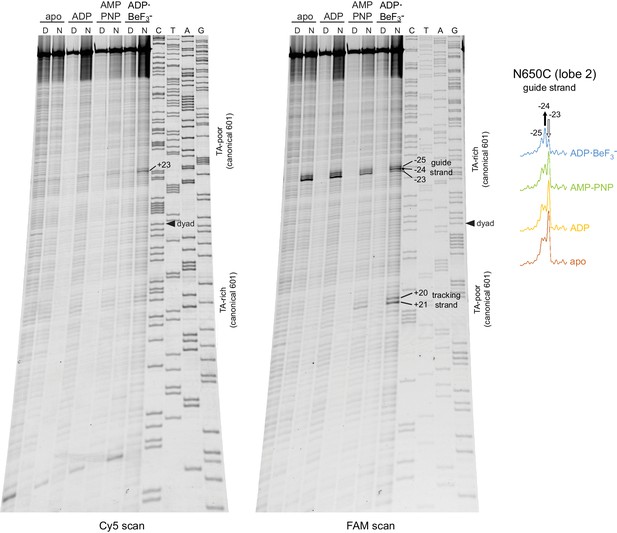
Chd1(N650C) cross-linking on 601[swap SHL2.5/3.5] nucleosomes yielded a distinct nucleotide-dependent pattern.
Altering the Widom 601 sequence affects the cross-linking pattern of the Chd1 ATPase motor. The major cross-linked species for the 601[swap SHL2.5/3.5] nucleosome (−23 to −25 on the guide strand) were on the opposite side compared to the canonical 601 (+23 to +25 on the guide strand). This difference corresponds with the swap of 24–39 bp segments on either side of the dyad. In addition to switching sides, cross-linking to the 601[swap SHL2.5/3.5] also displayed a different nucleotide- dependent pattern. For this nucleosome, cross-linking in ADP·BeF3– was enhanced at position −24 and diminished one nt closer to the dyad (−23) compared to the other nucleotide states. This contrasts with the canonical 601, which also showed stronger cross-linking in ADP·BeF3– at +24 compared to other nucleotide states, but had decreased cross-linking farther from the dyad at +25 (Figure 1).
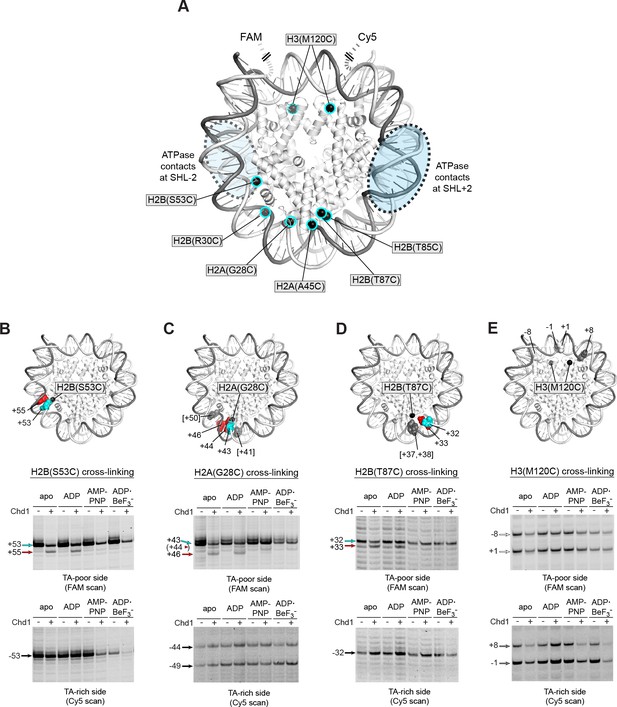
Chd1 binding in apo and ADP conditions shifts nucleosomal DNA on the TA-poor side of the 601 sequence.
(A) Molecular representation of a 601 nucleosome (Makde et al., 2010), indicating sites of cysteine substitution for site-specific cross-linking. Blue dotted ovals indicate the regions bound by the Chd1 ATPase motor at each SHL2 site (Farnung et al., 2017; Nodelman et al., 2017; Sundaramoorthy et al., 2018). (B) Histone H2B(S53C) cross-linking reactions in the presence and absence of Chd1. PyMOL representation shows cross-links on the TA-poor side of the nucleosome that occur for the nucleosome alone (cyan) and in the presence of Chd1 (red). Cross-linking was performed using 150 nM canonical 601 nucleosomes with 40 bp flanking DNA on each side (40N40), in the presence or absence of 600 nM Chd1. Cross-linked products were separated on urea denaturing gels and visualized by FAM or Cy5 fluorescence. Numbering refers to the distances of cross-linked sites from the 601 dyad (bp). (C) Histone H2A(G28C) cross-linking reactions, performed as described in B. In the PyMOL representation, sites of cross-linking that occur on the complementary strand are indicated by gray spheres with numbering in square brackets (see Figure 2—figure supplement 2B for +41 and +50 cross-linking sites). (D) Histone H2B(T87C) cross-linking reactions, performed as described in B. As for (C), cross-links that occur on the complementary strand [+37/+38] are shown as gray spheres in the PyMOL representation (Figure 2—figure supplement 2C). (E) Histone H3(M120C) cross-linking reactions, performed as described in B. All cross-linking experiments are representative of 3 or more experiments. Extended gel images are shown in Figure 2—figure supplement 2D.
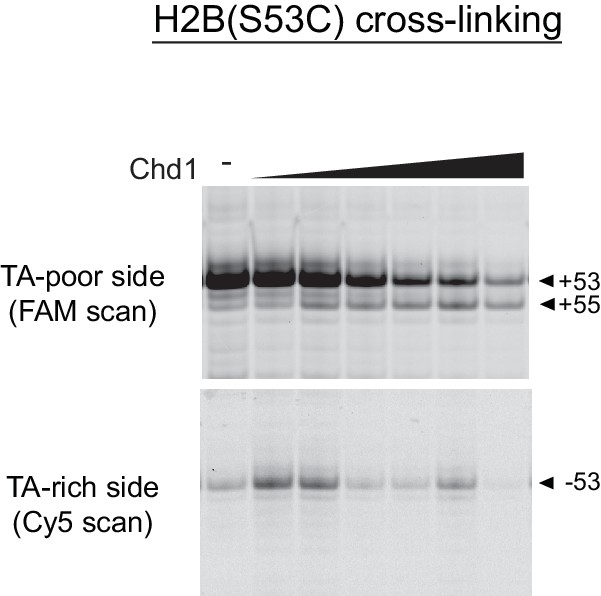
Titration of Chd1 for H2B(S53C) cross-linking reaction.
Increasing amounts of Chd1 produces a novel H2B(S53C) cross-link on the TA-poor side of the 601. In nucleotide-free conditions, Chd1 was added to final concentrations of 50, 150, 300, 600, 1200, and 2400 nM in the presence of 150 nM 40N40 nucleosome.
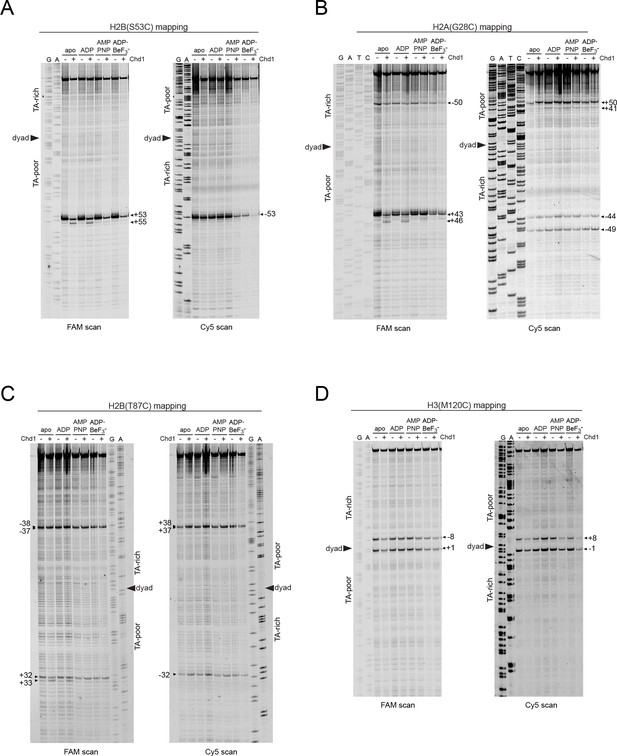
Extended gel images for histone mapping of Widom 601 nucleosomes.
Scans of urea denaturing gels, shown in Figure 2B–E for cross-linking reactions using 40N40 nucleosomes (canonical 601) containing H2B(S53C) (A), H2A(G28C) (B), H2B(T87C) (C), or H3(M120C) (D). Numbering represents the distance (nt) from the 601 dyad. Each reaction contained 600 nM Chd1 and 150 nM nucleosomes.
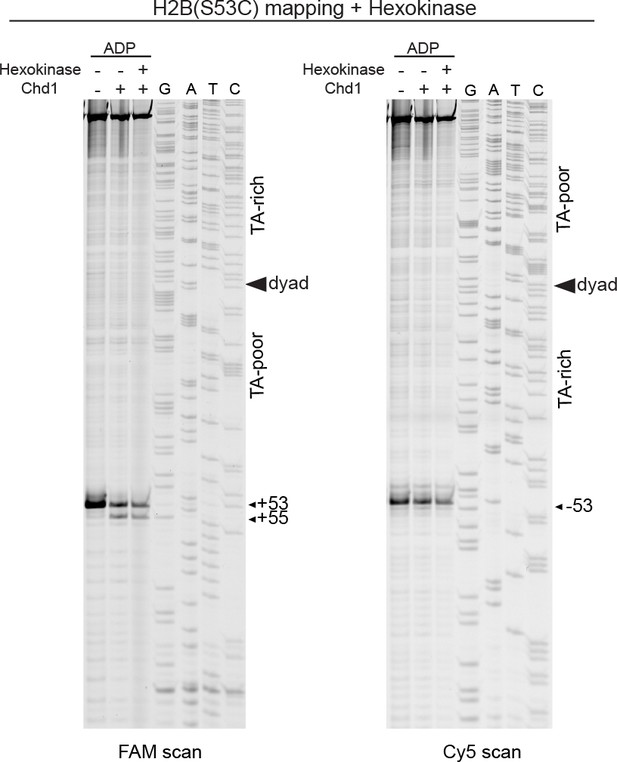
Treatment with hexokinase confirms that ADP is sufficient for supporting a Chd1-dependent shift in H2B(S53C) cross-linking.
Scans of urea denaturing gels for H2B(S53C) cross-linking reactions with 40N40 nucleosomes (canonical Widom 601) in the presence and absence of Chd1. ADP stocks used for this experiment were either untreated or pretreated with hexokinase and glucose to remove any contaminating ATP.
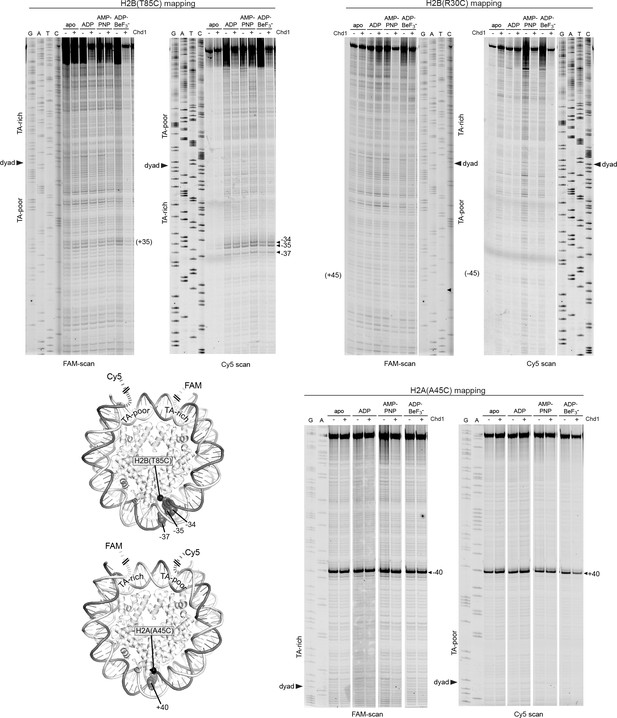
Histone mapping with other single cysteine histone variants.
Scans of urea denaturing gels for cross-linking reactions of 40N40 nucleosomes containing H2B(R30C), H2B(T85C), or H2A(A45C) variants. Note that the PyMOL representation of the H2B(T85C) cross-linking sites is flipped over to show the TA-rich side. Numbering represents observed or expected (parentheses) cross-links from the dyad.
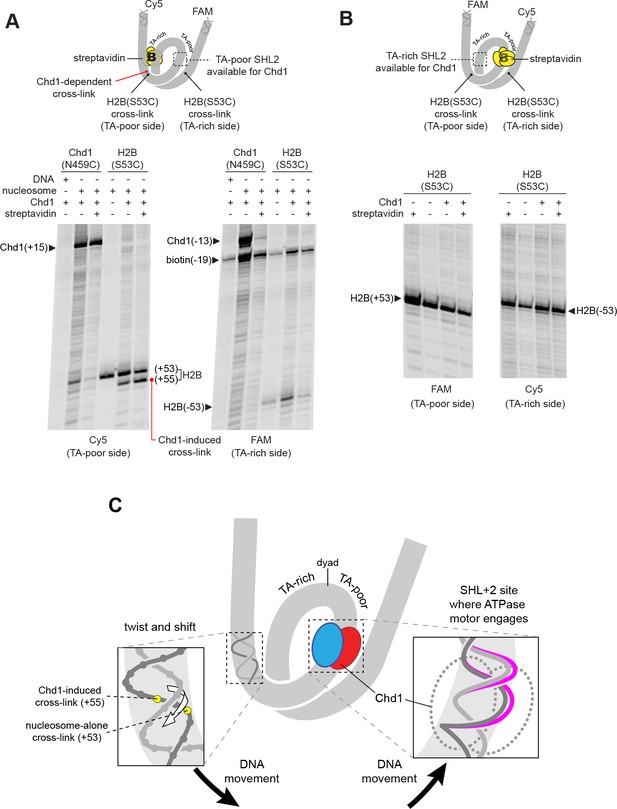
The Chd1-dependent shift in histone-DNA cross-linking correlates with Chd1 binding to the adjacent SHL2, on the same DNA gyre of the nucleosome.
(A) The presence of a biotin/streptavidin block at the TA-rich SHL–2 site does not diminish the Chd1-dependent shift in H2B(S53C) cross-links on the TA-poor side. The nucleosome substrate for these experiments was 19-[+biotin]601-29, with a biotin moiety 19 nt from the dyad on the TA-rich SHL–2 site. Note that for this nucleosome, the FAM and Cy5 labels are on opposite sides compared to all other 601 nucleosomes used in this study. Chd1(N459C) cross-linking experiments monitored Chd1 binding at each SHL2, and H2B(S53C) experiments followed the Chd1-dependent shift in histone-DNA cross-links on the TA-poor side. The apparent shift in Chd1(N459C) cross-linking site on the biotinylated strand (labeled as −13) was due in part to slower migration caused by the biotin moiety on the DNA fragment, and may not represent a true change in cross-linking position. The gels are representative of three or more experiments, and extended gel images are shown in Figure 3—figure supplement 1A. (B) The presence of a biotin moiety at the TA-poor SHL+2 site prevents the Chd1-dependent shift in H2B(S53C) cross-linking on the TA-poor side. The nucleosome substrate for these experiments was 40-601[+biotin]-19, with a biotin moiety 19 nt from the dyad on the TA-poor SHL+2 site. The absence of the H2B(S53C) shift likely stems from poor binding due to the biotin, as shown in Figure 3—figure supplement 2. The gels are representative of three or more experiments, and extended gel images are shown in Figure 3—figure supplement 1B. (C) A model for how Chd1 binding at SHL+2 may be coupled to shifts in DNA cross-linking.
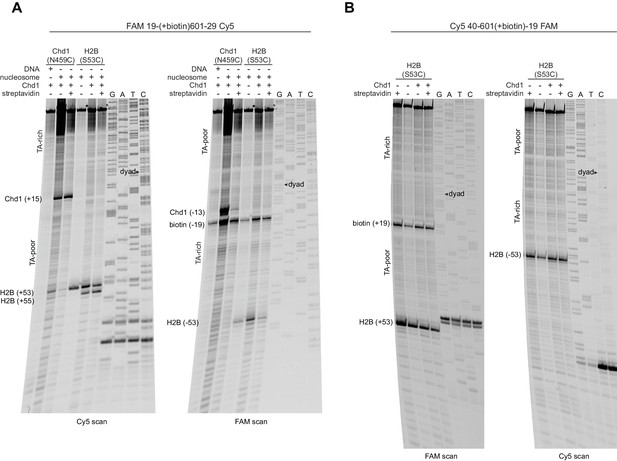
Extended gel images for H2B(S53C) cross-linking experiments with biotinylated 601 nucleosomes.
Scans of urea denaturing gels, shown in Figure 3, for reactions using nucleosomes that contained a biotin moiety 19 nt from the dyad. (A) Experiment using 19-(+biotin)601–29, which was biotinylated on the TA-rich side, as shown in Figure 3A. (B) Experiment using 40-601(+biotin)−19, which was biotinylated on the TA-poor side, as shown in Figure 3B. These experiments are representative examples of three or more independent experiments.
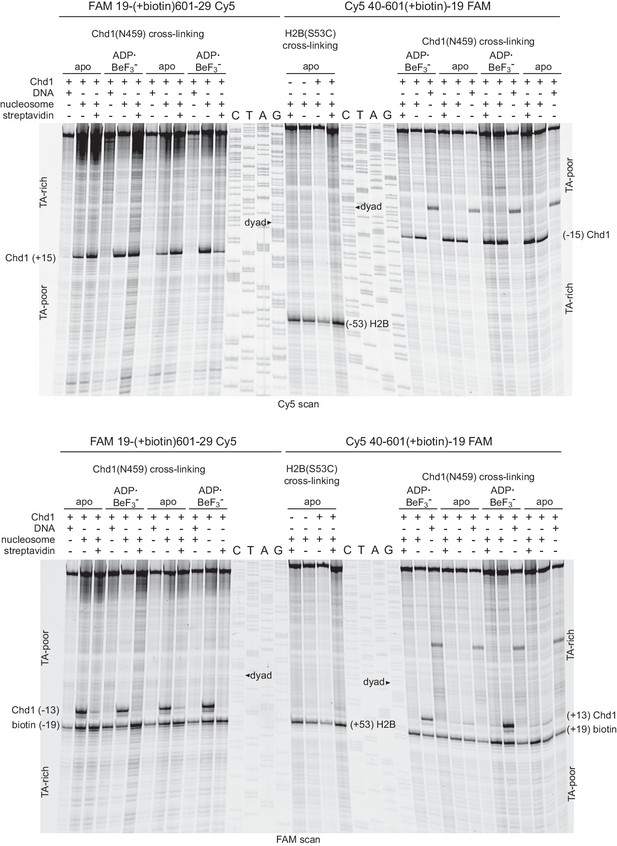
A biotin moiety on the TA-poor SHL2 interferes with Chd1 binding, independently of streptavidin.
Shown are duplicate experiments for Chd1(N459C) cross-linking, using nucleosomes with a biotin on the TA-rich SHL–2 (19-[+biotin]601–29, left) or the TA-poor SHL+2 (40-601[+biotin]−19, right). For both of these nucleosomes, biotin is 19 nt from the dyad on the tracking strand, and the numbers of the construct name indicate the lengths of DNA flanking the core 601 sequence. Note that the FAM label is on the same side as the biotin, so the TA-rich and TA-poor sides appear in opposite orientations for these two nucleosomes for each scan. The Cy5 scan (top) shows that in the absence of a biotin moiety, Chd1(N459C) cross-links strongly at the TA-rich and TA-poor SHL2 sites. In contrast, the FAM scan (bottom) shows a marked decrease in Chd1(N459C) cross-linking with biotin on the TA-poor side in apo but not ADP·BeF3– conditions. The middle lanes show a replicate experiment monitoring H2B(S53C) cross-linking for the 40-601[+biotin]−19 construct, where the biotin at the TA-poor SHL2 prevents a Chd1-induced shift.
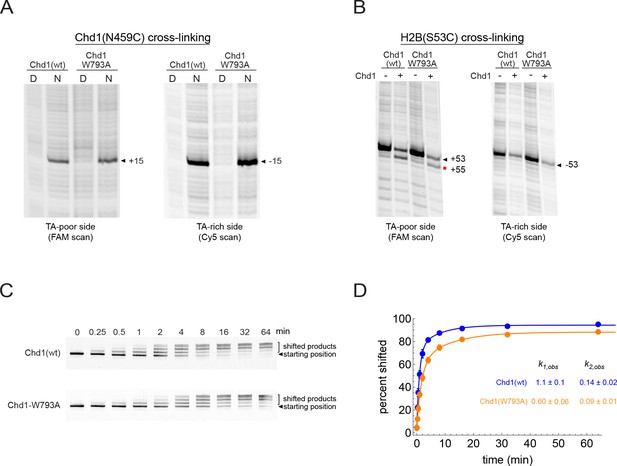
Mutation of a conserved tryptophan (W793A) in the ATPase core of Chd1 only modestly slows the rate of nucleosome sliding and does not interfere with the two nt shift of H2B(S53C).
(A) The W793A mutation does not interfere with ATPase binding at SHL2. Shown are cross-linking reactions for Chd1(N459C) with and without the W793A mutation. Cross-linking was performed without nucleotide. No differences in cross-linking are apparent. (B) The W793A mutation does not disrupt the Chd1-dependent shift in H2B(S53C) cross-linking on the TA-poor side. These cross-linking reactions were carried out with the double mutant variant Chd1(N495C/W793A) under apo conditions. (C) Native gel sliding assay, showing repositioning of nucleosomes over time by wildtype Chd1 and a Chd1(W793A) variant. In this experiment, 50 nM Chd1 was incubated with 150 nM 0N80 nucleosome in the presence of 2.5 mM ATP and 5 mM MgCl2. (D) Quantitation and fitting of native gel sliding data shown in C. Colored circles are the averages of five replicates, with standard deviations shown as error bars (often obscured markers). Data were fit to a double exponential, and for the faster rate, Chd1(W793A) was approximately 2-fold slower than wild type Chd1.
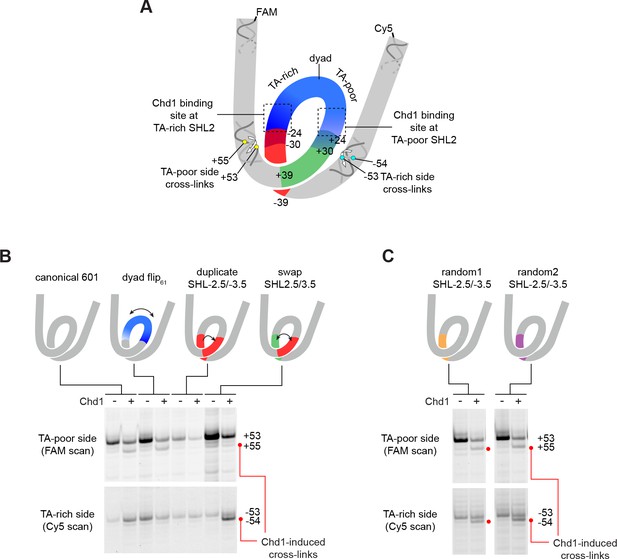
The sequence of DNA 24–39 bp from the dyad can restrict or permit shifts in H2B(S53C) cross-linking upon Chd1 binding.
(A) Schematic representation of the nucleosome, highlighting sequence elements of the canonical Widom 601 sequence that are altered in 601 variants. (B) The 24–39 bp segment of the canonical 601 is responsible for allowing or blocking Chd1-dependent shifts in H2B(S53C) cross-linking. Shown are cross-linking experiments for the canonical Widom 601 and three 601 variants, carried out with 150 nM 40N40 nucleosomes and 600 nM Chd1 under apo conditions. Schematic cartoons indicate the region of 601 altered for each nucleosome variant. (C) Replacing the 24–39 bp segment on the TA-rich side of the 601 with random DNA sequences allows Chd1 binding (apo condition) to stimulate a shift in H2B(S53C) cross-linking on the TA-rich side. Each gel in B and C is representative of 4 or more experiments. The DNA sequences for all 601 variants are given in Figure 1—figure supplement 2. Similar experiments to those carried out in B and C but in other nucleotide conditions, are shown in Figure 4—figure supplement 1.
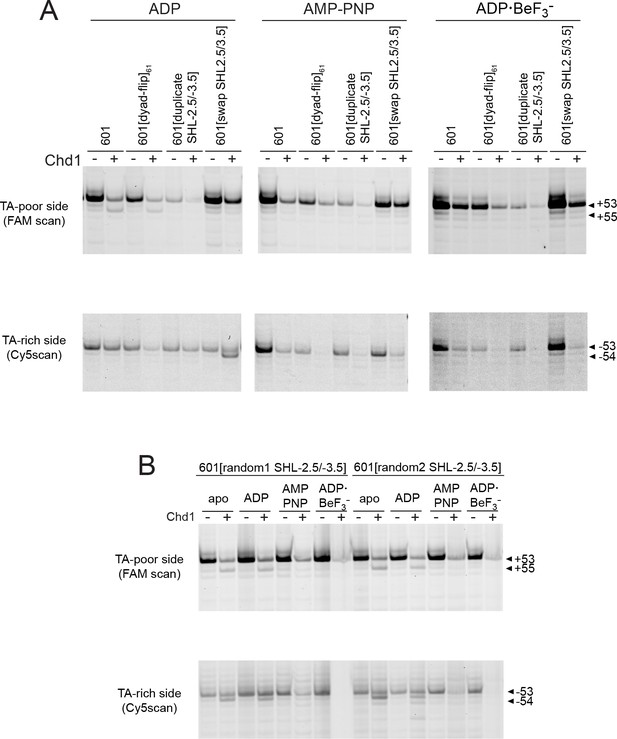
Effects of Chd1 binding in different nucleotide states on H2B(S53C) histone mapping of 601 variants.
Histone H2B(S53C) mapping reactions carried out in the presence or absence of 600 nM Chd1 in ADP, AMP-PNP, and ADP·BeF3– conditions. Gels are representative of 4 or more experiments, and correspond to the 601 variants given in Figure 4B (A) or Figure 4C (B).
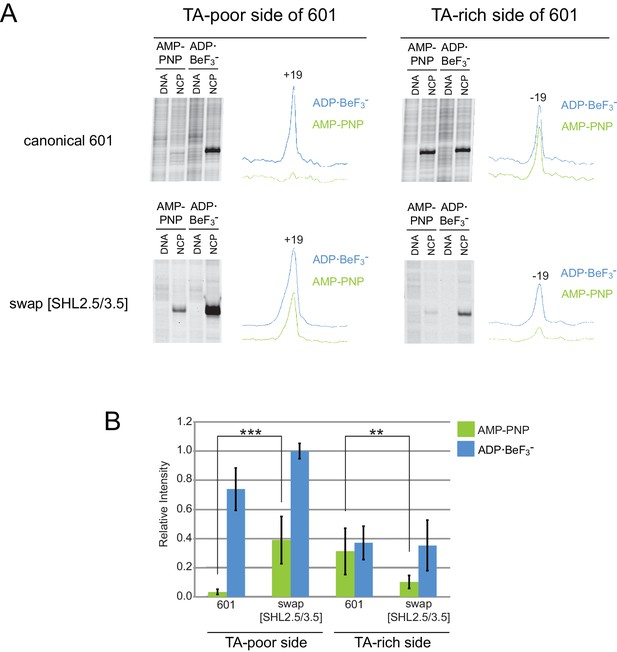
Distinct TA-poor and TA-rich Chd1 cross-linking patterns are transposed on the 601[swap SHL2.5/3.5] nucleosome.
(A) Chd1(V721C) cross-linking reactions on the Widom 601 and 601[swap SHL2.5/3.5] nucleosomes in AMP-PNP and ADP·BeF3– conditions. The Widom 601 nucleosome has reduced cross-linking on the TA-poor side when compared to the TA-rich side, whereas the 601[swap SHL2.5/3.5] nucleosome reverses this trend with diminished cross-linking on the TA-rich side compared to the TA-poor side. Each trace represents the intensity profile of the corresponding NCP gel lane, obtained using ImageJ. (B) Quantification of Chd1(V721C) cross-linking data. The area of each peak was calculated in ImageJ (RRID: SCR_003070), scaled according to background peaks, and normalized against a 601[swap SHL2.5/3.5] sample in ADP·BeF3– . Error bars signify the standard deviations from three or more experiments. The differences in Chd1 cross-linking in AMP-PNP for canonical 601 versus 601[swap SHL2.5/3.5] are statistically significant (** p-value < 0.01, *** p-value ≤ 0.001).
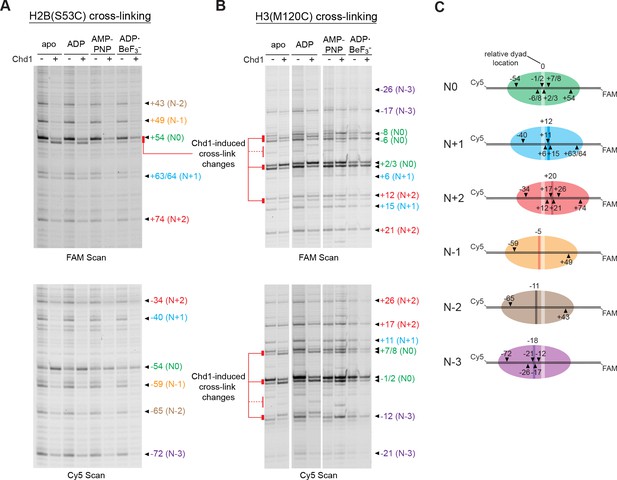
Chd1 binding alters histone-DNA contacts of 5S nucleosomes in a nucleotide-dependent manner.
(A) Mapping interactions of histone H2B(S53C) with 5S nucleosomal DNA. Histone cross-linking was performed in the presence or absence of 600 nM Chd1, using a sample that was enriched in centered nucleosomes. Cross-linked products were separated on urea denaturing gels and visualized by FAM or Cy5 fluorescence. Numbering of each cross-linking site is relative to the dyad of the dominant centered species, called N0 (green). Cross-links predicted to come from the same nucleosome species are given matching colors. Prominent Chd1-induced changes in histone cross-linking are highlighted by red bars. (B) Mapping interactions of histone H3(M120C) with 5S nucleosomal DNA. Cross-linking reactions were performed similarly to those described in A. (C) Schematic representations of 5S octamer positions. Cross-links observed for H2B(S53C) and H3(M120C) are indicated with black triangles pointing to the labeled DNA strand (FAM or Cy5) where they were observed. The dyad position of the enriched N0 species is indicated by a light stripe, while the dyads for the other species are shown as dark stripes, with the approximate number of nt differing from N0 given above each stripe. The 5S sequence is given in Figure 5—figure supplement 1.

Sequence and dominant centered location of 5S nucleosomes.
The approximated dyad of the dominant species, named N0, is considered to be 0. The DNA wrapped around the histone core is highlighted in gray. Since site-specific crosslinking used here only reports on internal histone-DNA contacts, the ambiguity of where the DNA wrapping begins is shown by the fading gray highlight.
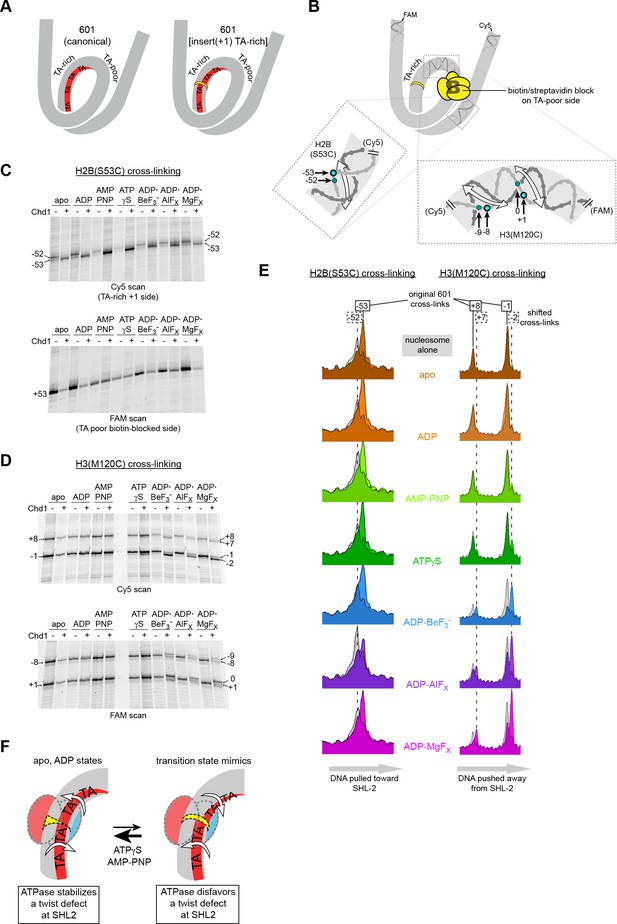
Chd1 binding induces a nucleotide-dependent twist in nucleosomal DNA at SHL2.
(A) Schematic view of TA steps on the TA-rich side of the 601, which are phased in the canonical Widom 601 (left) and expected to be out-of-phase upon addition of a single bp at position 22 (yellow highlight, right). (B) Experimental design for monitoring histone-DNA contacts of the 601[insert(+1) TA-rich] nucleosomes. The presence of biotin/streptavidin at position 19 on the TA-poor side of the nucleosome will block Chd1 binding to SHL+2. Cross-linking of H2B(S53C) and H3(M120C) will report on the effects of Chd1 binding to SHL–2. In the expanded views, filled blue circles indicate the locations of cross-links as observed on the Cy5-labeled strand. For the H2B(S53C) view, the hatched blue circle denotes cross-links observed due to the +1 bp insertion on the TA-rich side as shown in (C), whereas for the H3(M120C) view, the hatched circles show the new dyad cross-links that appear with Chd1 bound in the presence of transition state analogs as shown in (D). Note that this design represents information gained from two different nucleosomes, each with a biotin/streptavidin block but with unique cross-linking sites. (C) The presence of Chd1 shifts entry DNA of 601[insert(+1) TA-rich] nucleosomes toward SHL–2 in all nucleotide conditions. Histone mapping reactions for biotinylated H2(S53C) nucleosomes plus streptavidin were carried out in the presence and absence of Chd1. The numbers indicate the cross-linking positions relative to the dyad, as observed for the canonical Widom 601 sequence. (D) Chd1 binding changes the position of DNA around the dyad of 601[insert(+1) TA-rich] nucleosomes in a nucleotide-dependent fashion. Cross-linking reactions were performed with biotinylated H3(M120C) nucleosomes in the presence of streptavidin. Gels shown in (C) and (D) are representative of four or more experiments. (E) Chd1 alters the distance between histone-DNA cross-links in a nucleotide dependent manner. Shown are intensity plots of each gel lane shown for Cy5 scans in (C) and (D). Nucleosome alone lanes are shown in gray, overlaid with the positions of cross-links observed for each nucleotide-bound state of Chd1 as colored peaks. The dotted lines indicate the positions of cross-links that are shifted 1 bp from those observed for the canonical 601, and correspond to the hatched blue circles shown in (B). The shift in cross-linking of H2B(S53C) from −52 to −53 corresponds with movement of entry DNA toward SHL–2, whereas the shift in H3(M120C) cross-links from +8/–1 to +7/–2 corresponds with movement of dyad DNA away from SHL–2. (F) A cartoon interpretation of Chd1-dependent changes in nucleosomal DNA.
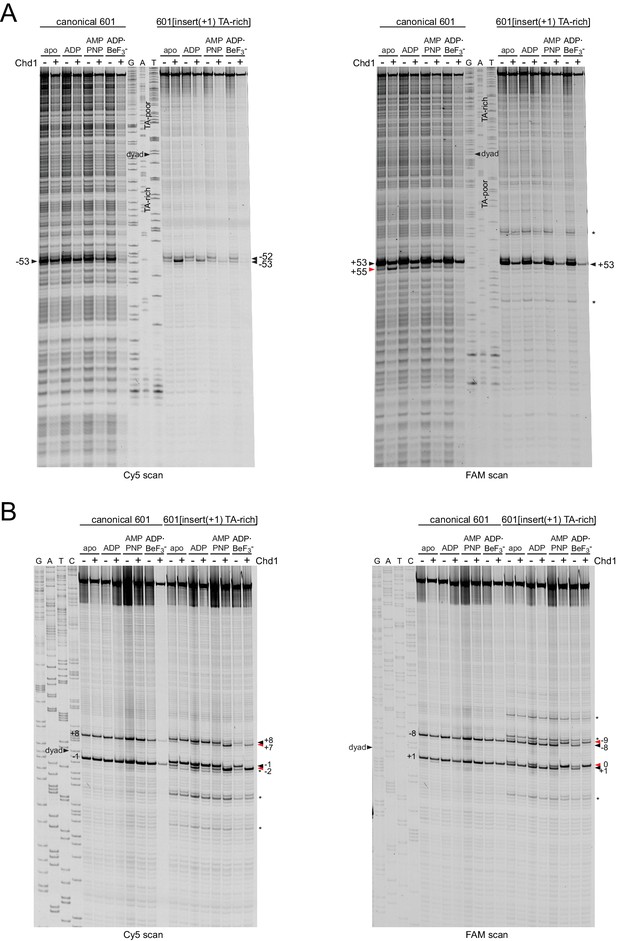
Chd1 binding shifts DNA at the dyad of 601[insert(+1) TA-rich] in a nucleotide-dependent fashion.
Shown are histone cross-linking reactions for H2B(S53C) (A) and H3(M120C) (B) for canonical and 601[insert(+1) TA-rich] nucleosomes. Note that unlike the nucleosomes shown in Figure 6, these did not have a biotin/streptavidin block. Chd1-dependent changes in histone cross-linking are highlighted by red triangles. Cross-links to nucleosomes that are positioned off of the expected 601 dyad are marked with an asterisk (*).
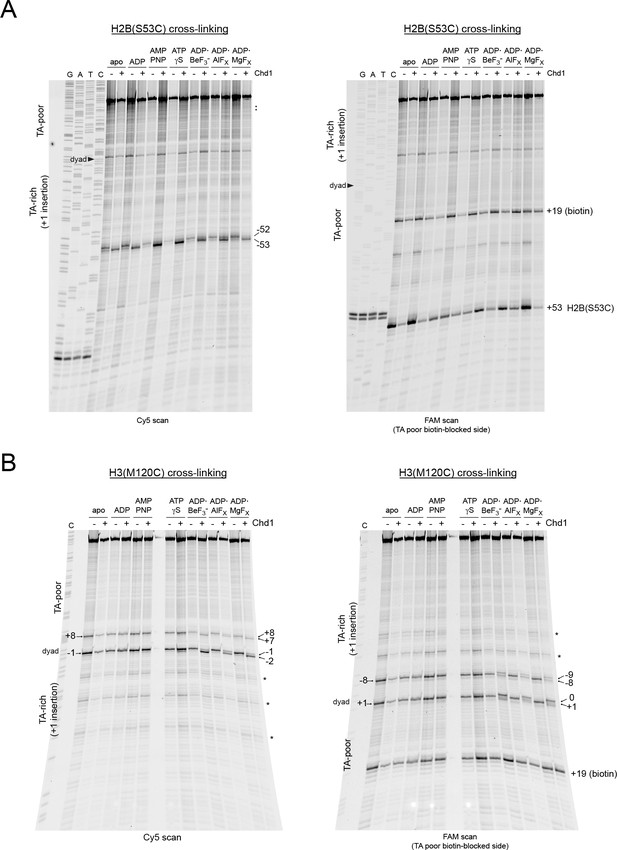
Extended gel images of H2B and H3 mapping reactions of 601[insert(+1) TA-rich] nucleosomes containing a biotin/streptavidin block.
Scans of urea denaturing gels, shown in Figure 6, for histone cross-linking reactions of Cy5-40N19-FAM nucleosomes made with the 601[insert(+1) TA-rich] template and with a biotin at +19 on the TA-poor side. (A) Cross-linking reactions using H2B(S53C) nucleosomes, performed plus and minus Chd1 in the presence of streptavidin. (B) Cross-linking reactions using H3(M120C) nucleosomes, performed plus and minus Chd1 in the presence of streptavidin. These gels are representative of four or more experiments. Cross-links to nucleosomes that are positioned off of the expected 601 dyad are marked with an asterisk (*).
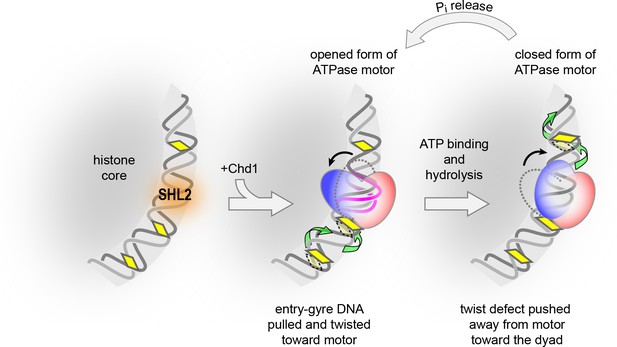
A model for DNA translocation on the nucleosome.
Binding of the ATPase motor in an open state stabilizes a twist defect at SHL2, which pulls entry-side DNA toward the remodeler in a corkscrew-like motion. The closed state of the motor attained during ATP hydrolysis is incompatible with the twist defect at SHL2, forcing the extra DNA toward the dyad. Cycling of the ATPase motor therefore both creates twist defects and stimulates twist diffusion around the histone core.
Additional files
-
Transparent reporting form
- https://doi.org/10.7554/eLife.34100.026