Optogenetic dissection of descending behavioral control in Drosophila
Figures
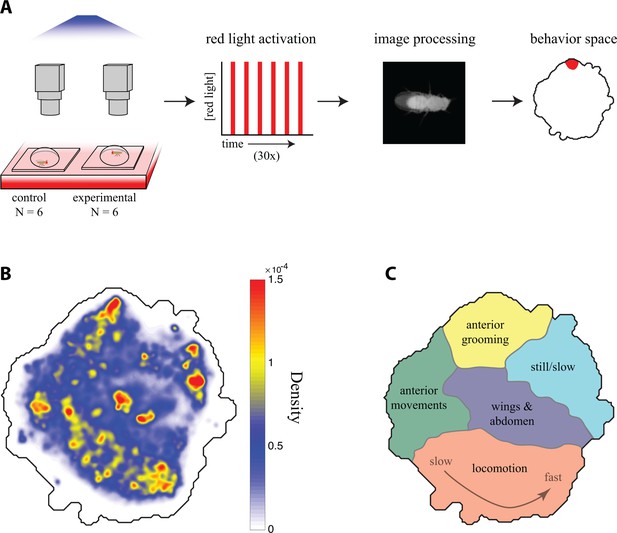
Descending neuron phenotyping pipeline and behavior space.
(A) The red light activation rig. Six no retinal control flies and six retinal fed experimental flies were mounted in parallel in individual 3 cm diameter plexiglass bubbles on top of three custom light boards with constant 850 nm infrared light and variable 617 nm red light. The red LEDs were repeatedly turned off and on for 45 and 15 s, respectively. Each fly was filmed at 150 × 150 pixel, 100 fps resolution by a single camera. Video data was then aligned and processed, and the line was characterized for its occupancy in the descending neuron behavior space with respect to red light activation and controls. (B) A 2D representation of behaviors in the descending neuron video dataset was generated by applying a probability density function to all the embedded data points (scale bar), which was then convolved with a Gaussian (σ = 1.5) (C) Localization of various behaviors within the descending neuron behavior space seen in (B), based on human curation of watershedded regions in the space (Figure 1—figure supplement 2, Videos 1–6). Note that as locomotion behaviors move from left to right along the bottom of the map, the fly’s running speed increases.
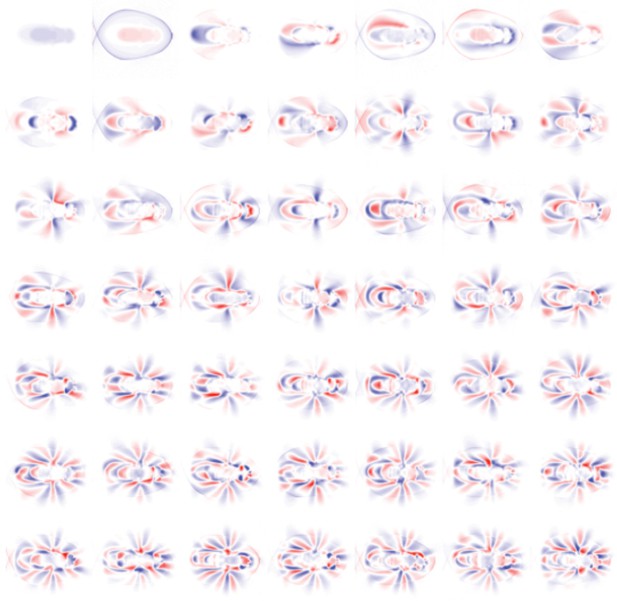
Postural eigenmodes used to build the descending neuron behavior space (Figure 1B).
https://doi.org/10.7554/eLife.34275.003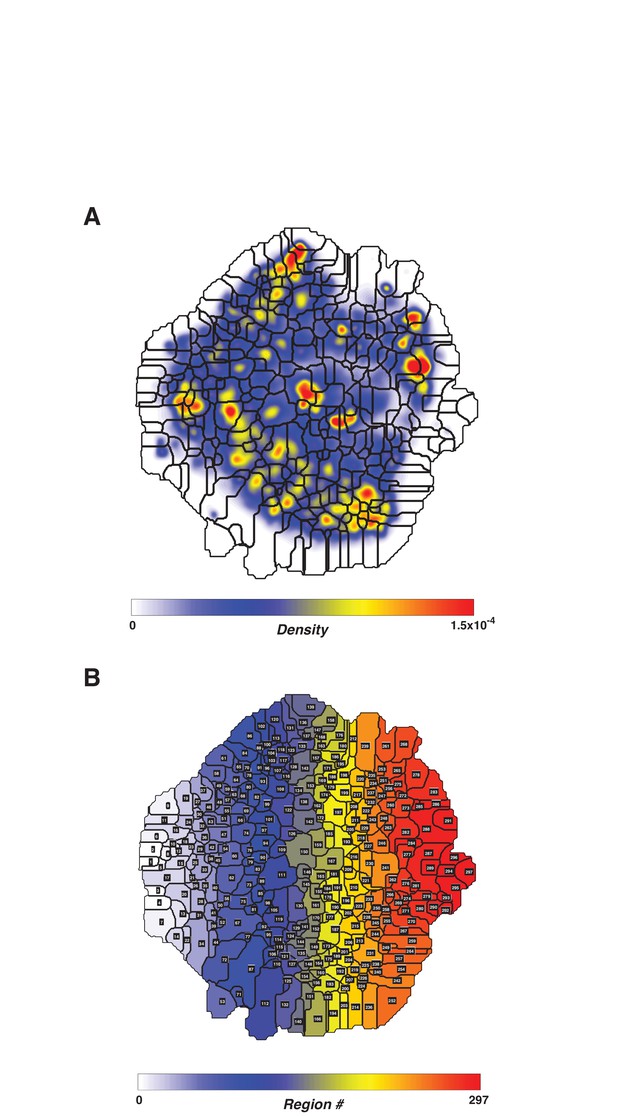
Watershedded regions in the descending neuron behavior space.
(A) A watershedding algorithm was used on the behavior space in Figure 1B to identify local density maxima, which represent stereotyped behaviors. (B) Numbered watershedded regions. These correspond to videos underlying all the watershedded regions (Video 1 through S6) which were examined to create the human curated version of the behavior space in Figure 1C.
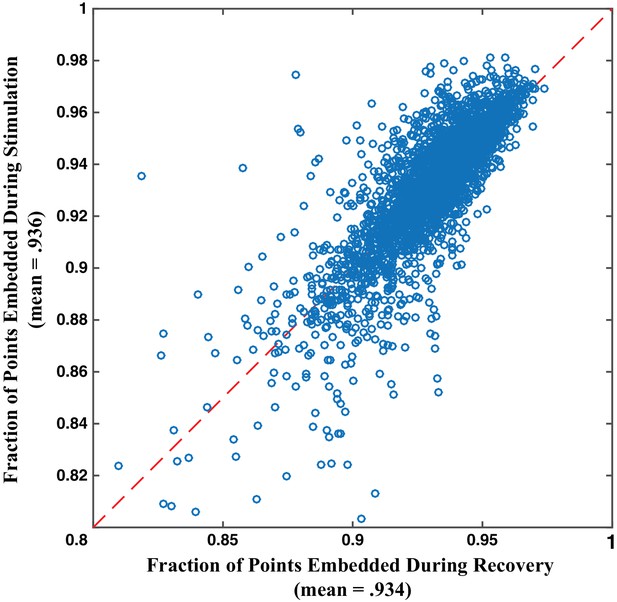
Fraction of video data points for each movie embedded in the behavior spce, during the red light stimulus window (Y axis) and during the recovery period (X axis).
For most movies, between 90% and 95% of frames embedded, with very little difference between when the red light is on and when the red light is off, indicating that activated behaviors are well represented in the behavior space. Failure to embed is usually indicative of imaging flaws, for example when part of the fly was out of the field of view of the camera.
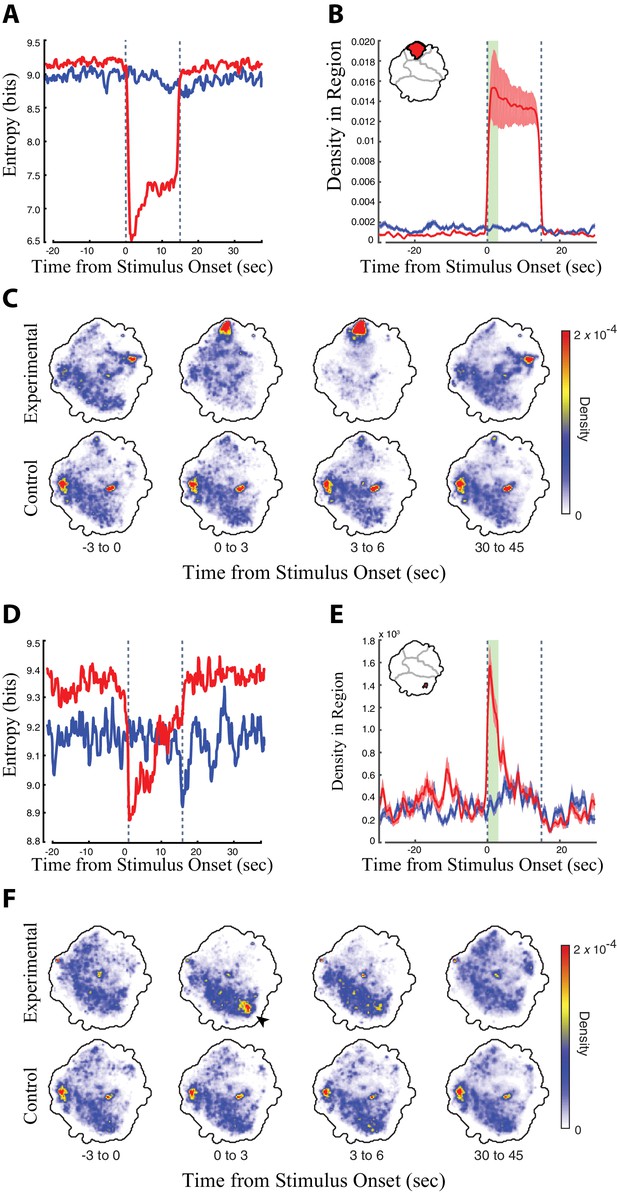
Analysis of the head grooming DNg07 and DNg08 line (SS02635) and the transient fast-running DNg25 line (SS01602).
(A, D) Entropy of the distribution of the retinal fed experimental flies (red) and non-retinal fed control flies (blue) in the behavior space relative to the timing of red light stimulus onset (red light turned on at t = 0 s, the time of red light activation is indicated by dashed lines). The behavior space for experimental flies shows reduced entropy when the flies perform a specific set of behaviors, because flies shift from the full range of normal fly behaviors to a subset of red light-activated behaviors. (B, E) Average density ± the standard deviation in the head grooming map region indicated in red (inset, upper right) in experimental flies (red) and controls (blue) relative to red light activation. The head grooming region was calculated as the region in the map that experienced a statistically significant shift in density in experimental flies but not controls when comparing the first 3 s (green bar) of the activation period to the last 15 s of the recovery period (Wilcoxon rank sum test, p<0.05, using the Dunn–Šidák correction for multiple hypotheses). (C, F) Average density in the map over a series of 3 s windows (calculated from six animals, 30 trials each). Red and blue indicate regions of high and low density, respectively. The time ‘before’ stimulus onset is the average of 30 time periods between stimulations. We found an increase in the amount of ‘Idle/Slow’ dynamics for experimental flies in the interstitial times between stimulations. Thus, the differences between experimental and control animals in the time ‘before’ stimulation most likely reflects this increase in the amount of Idle/Slow behavior for experimental animals in the time between stimulation.
-
Figure 2—source data 1
Analysis of all the descending neuron split-GAL4 lines.
The line by line analysis shown here follows the model of the selected examples described in Figure 2 of the main text. Also available at: http://www.biology.emory.edu/Berman/files/FigureS5_Cande_et_al.pdf.
- https://doi.org/10.7554/eLife.34275.014
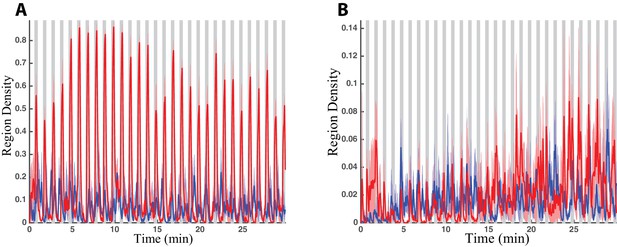
Density in experimental (red) and control (blue) animals in the regions defined in Figure 2A and D (and B, this figure, respectively).
Densities shown in Figure 2B and E were averaged for six animals over 30 min. Gray bars indicate periods of red light activation.
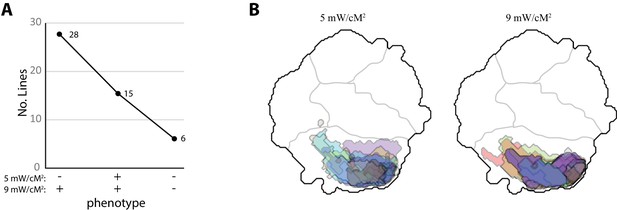
Choosing the red light intensity levels.
(A) While most lines were screened at 5 mW/cm[2], 49 lines that had noor only a weak phenotype at this light level were repeated at 9 mW/cm2 and a higher retinal concentration. Forty-three of these lines acquired a phenotype or a strengthened statistical significance of the previously observed phenotype under these conditions. No lines lost their phenotype at the higher intensity. (B) 10 transient locomotion lines, overlaid in the same behavior space in different colors, were repeated at the lower and higher light intensities and retinal concentrations. The boundaries of the significant regions in the behavior space shift slightly between treatments, but overall the phenotypes remain largely unchanged.
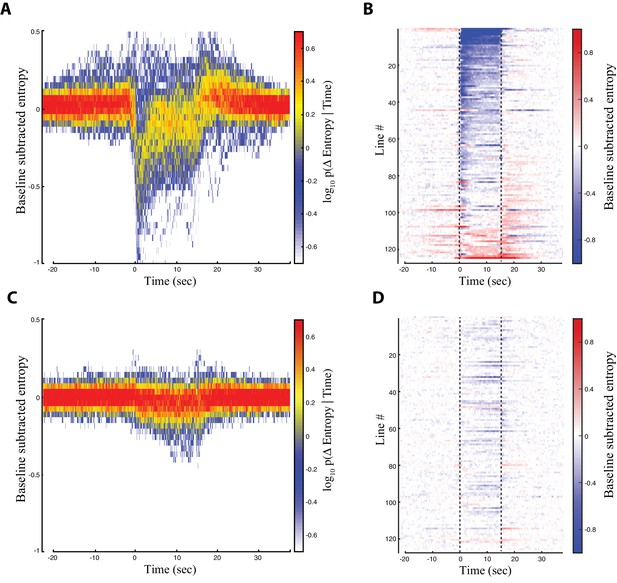
Entropy for all the descending neuron split-GAL4 lines.
Trials were aligned such that the onset of red light activation occured at t = 0 s. The red light was turned off at t = 15 s. (A) Baseline subtracted entropy (Y-axis) versus time (X-axis) for all experimental animals and all trials. Colors indicate a probability distribution describing the entropy of experimental animals at a given time in the trial. (B) Entropy levels of experimental animals over the course of the aligned trials (X-axis) shown by line (Y-axis). Warm and blue colors indicate high and low entropy, respectively. (C) and (D) Same as (A) and (B), but for the control animals. Lines are presented in the same order as in (B) and (D).
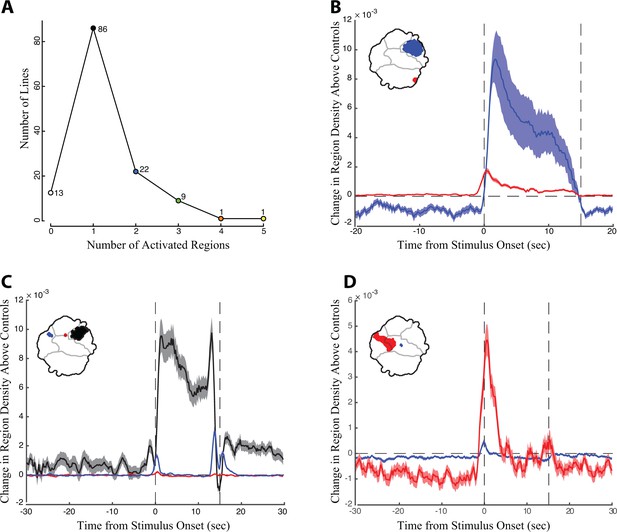
The timing of density shifts in descending neuron lines that occupy multiple behavior space regions.
(A) Most lines (86) increase density in only one region of the behavior space upon red light activation. However, some lines occupy multiple regions in the behavior space. (B–D) Examples of lines that occupy multiple discontinuous regions upon red light activation. Time is indicated on the X-axis, with the red light turned on at T = 0 s and off at 15 s. Change in density in the color coded regions in the experimental animals above the controls is indicated on the Y-axis. (B) Line SS01540 targeting descending neuron DNp09; (C) SS02542 targeting descending neuron DNb01; (D) SS01049 targeting descending neuron DNp10. In many cases, as shown here, we observed an increase in region density that appears to occur immediately before the stimulus onset. This increase in density probably results from temporal smoothing of approximately 1 s that is introduced through the wavelet transformation of the behavioral analysis and not due to behavioral anticipation of the light stimulus.
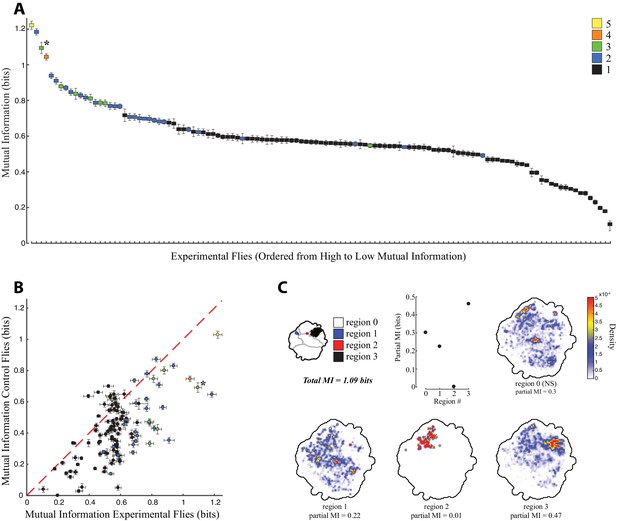
Mutual information between behaviors performed before and during red-light activation.
(A) Mutual information between pre-stimulation and post-stimulation behavior for experimental flies, calculated using the density of the flies in the behavior space at t = −1.5 to −0.5 s prior to red light activation versus density at t = 0 to 1.0 s after red light activation. Y-axis indicates the mutual information, X-axis is all the lines in order of most to least mutual information, error bars indicate the standard deviation. Lines are color coded by the number of significant regions produced by red light activation, the key is indicated in the upper right, line SS02542 is indicated by an asterisk. (B) Mutual information between pre-stimulation and post-stimulation behavior of experimental flies (X-axis) plotted against the same quantity for the non-retinal fed control flies (Y-axis), with the same color coding as (A). Most experimental animals display stronger mutual information than control flies, implying that descending neuron activation makes fly behavior more dependent on prior state than in control flies. (C) Partial mutual information for SS02542, showing the density at t = −1.5 to 0.5 that has mutual information with the different regions of the behavior space after red light activation indicated in the key in the upper left portion of the figure.
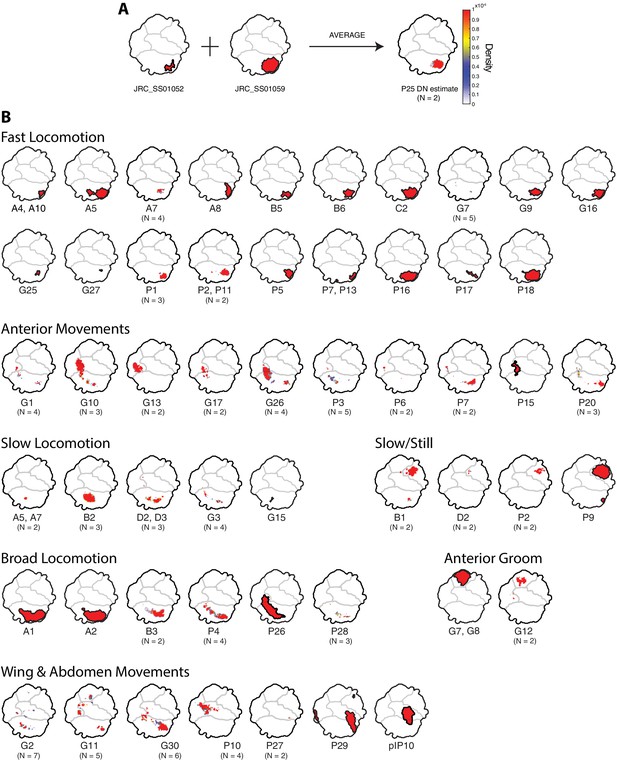
Averages of representative lines for individual descending neurons.
Descending neurons are organized according to the region of the behavior space that they activate (Figure 1). (A) Example illustrating the averaging of two lines to produce an estimated phenotype. Colors indicate the degree to which particular regions are represented on average. Red regions are highly represented, blue less so, and white not at all. (B) Phenotypes for 53 of the 58 descending neurons in the collection, plus pIP10 (von Philipsborn et al., 2011). Some descending neurons are represented by a single clean line, others by averaging multiple clean lines. For averages, the number of lines are indicated under the descending neuron name.
Videos
Video maps of the behavioral space for anterior movements indicated in Figure 1C.
Each movie shows a sequence of highlighted regions in the space, followed by a selection of 64 sequences of translationally and rotationally aligned fly behavior from that region. This selection is performed at random (amongst all sequences lasting at least 0.2 s within that region), showing how the raw videos are translated into the map. Because of this randomness, there are occasional image processing errors, cases where the fly goes out of the camera range, and instances of flies writhing upside-down. Note, however, that most of these sequences are sequestered to particular locations within the space, and that no descending neuron line predicts an increase in activity within these regions.
Video maps of the behavioral space for anterior grooming indicated in Figure 1C.
Each movie shows a sequence of highlighted regions in the space, followed by a selection of 64 sequences of translationally and rotationally aligned fly behavior from that region. This selection is performed completely at random (amongst all sequences lasting at least 0.2 s within that region), showing how the raw videos are translated into the map. Because of this randomness, there are occasional image processing errors, cases where the fly goes out of the camera range, and instances of flies writhing upside-down. Note, however, that most of these sequences are sequestered to particular locations within the space, and that no descending neuron line predicts an increase in activity within these regions.
Video maps of the behavioral space for still/slow behavior indicated in Figure 1C.
Each movie shows a sequence of highlighted regions in the space, followed by a selection of 64 sequences of translationally and rotationally aligned fly behavior from that region. This selection is performed completely at random (amongst all sequences lasting at least 0.2 s within that region), showing how the raw videos are translated into the map. Because of this randomness, there are occasional image processing errors, cases where the fly goes out of the camera range, and instances of flies writhing upside-down. Note, however, that most of these sequences are sequestered to particular locations within the space, and that no descending neuron line predicts an increase in activity within these regions.
Video maps of the behavioral space for wing and abdomen movements indicated in Figure 1C.
Each movie shows a sequence of highlighted regions in the space, followed by a selection of 64 sequences of translationally and rotationally aligned fly behavior from that region. This selection is performed completely at random (amongst all sequences lasting at least 0.2 s within that region), showing how the raw videos are translated into the map. Because of this randomness, there are occasional image processing errors, cases where the fly goes out of the camera range, and instances of flies writhing upside-down. Note, however, that most of these sequences are sequestered to particular locations within the space, and that no descending neuron line predicts an increase in activity within these regions.
Video maps of the behavioral space for locomotion indicated in Figure 1C.
Each movie shows a sequence of highlighted regions in the space, followed by a selection of 64 sequences of translationally and rotationally aligned fly behavior from that region. This selection is performed completely at random (amongst all sequences lasting at least 0.2 s within that region), showing how the raw videos are translated into the map. Because of this randomness, there are occasional image processing errors, cases where the fly goes out of the camera range, and instances of flies writhing upside-down. Note, however, that most of these sequences are sequestered to particular locations within the space, and that no descending neuron line predicts an increase in activity within these regions.
Effect of optogenetic stimulation on descending neurons G7 and G8.
This movie displays two different flies from the same line (SS02635) for 10 s before and 10 s during optogenetic stimulation . Red lights on is indicated by the text ‘RED LIGHT’ appearing in the lower right. Note the change in behavior immediately following stimulation.
Behavioral space dynamics during optogenetic stimulation for descending neurons G7 and G8 (line SS02635).
Movies show the behavioral space changes from before stimulation, at the onset of stimulation, during stimulation, and after stimulation. Each movie shows the space, averaged over six experimental flies and all 30 LED cycles, for 5 s before, the 15 s during, and 5 s after the onset of stimulation (t = 0). Each frame in the video is created by finding all behavioral space positions within a 0.5 s window surrounding the displayed time and convolving each of these points with a two-dimensional gaussian of width 3 (the full movie is of height and width 210 in arbitrary units).
Behavioral space dynamics during optogenetic stimulation for descending neuron G25 (line SS01602).
These movies show the behavioral space changes from before stimulation, at the onset of stimulation, during stimulation, and after stimulation. Each movie shows the space, averaged over six experimental flies and all 30 LED cycles, for 5 s before, the 15 s during, and 5 s after the onset of stimulation (t = 0). Each frame in the video is created by finding all behavioral space positions within a 0.5 s window surrounding the displayed time and convolving each of these points with a two-dimensional gaussian of width 3 (the full movie is of height and width 210 in arbitrary units).
Behavioral space dynamics during optogenetic stimulation for descending neuron G9 (line SS01540).
These movies show the behavioral space changes from before stimulation, at the onset of stimulation, during stimulation, and after stimulation. Each movie shows the space, averaged over six experimental flies and all 30 LED cycles, for 5 s before, the 15 s during, and 5 s after the onset of stimulation (t = 0). Each frame in the video is created by finding all behavioral space positions within a 0.5 s window surrounding the displayed time and convolving each of these points with a two-dimensional gaussian of width 3 (the full movie is of height and width 210 in arbitrary units).
Behavioral space dynamics during optogenetic stimulation for descending neuron B1 (line SS02542).
These movies show the behavioral space changes from before stimulation, at the onset of stimulation, during stimulation, and after stimulation. Each movie shows the space, averaged over six experimental flies and all 30 LED cycles, for 5 s before, the 15 s during, and 5 s after the onset of stimulation (t = 0). Each frame in the video is created by finding all behavioral space positions within a 0.5 s window surrounding the displayed time and convolving each of these points with a two-dimensional gaussian of width 3 (the full movie is of height and width 210 in arbitrary units).
Behavioral space dynamics during optogenetic stimulation for descending neuron P10 (line SS01049).
These movies show the behavioral space changes from before stimulation, at the onset of stimulation, during stimulation, and after stimulation. Each movie shows the space, averaged over six experimental flies and all 30 LED cycles, for 5 s before, the 15 s during, and 5 s after the onset of stimulation (t = 0). Each frame in the video is created by finding all behavioral space positions within a 0.5 s window surrounding the displayed time and convolving each of these points with a two-dimensional gaussian of width 3 (the full movie is of height and width 210 in arbitrary units).
Additional files
-
Transparent reporting form
- https://doi.org/10.7554/eLife.34275.025