Kasugamycin potentiates rifampicin and limits emergence of resistance in Mycobacterium tuberculosis by specifically decreasing mycobacterial mistranslation
Figures
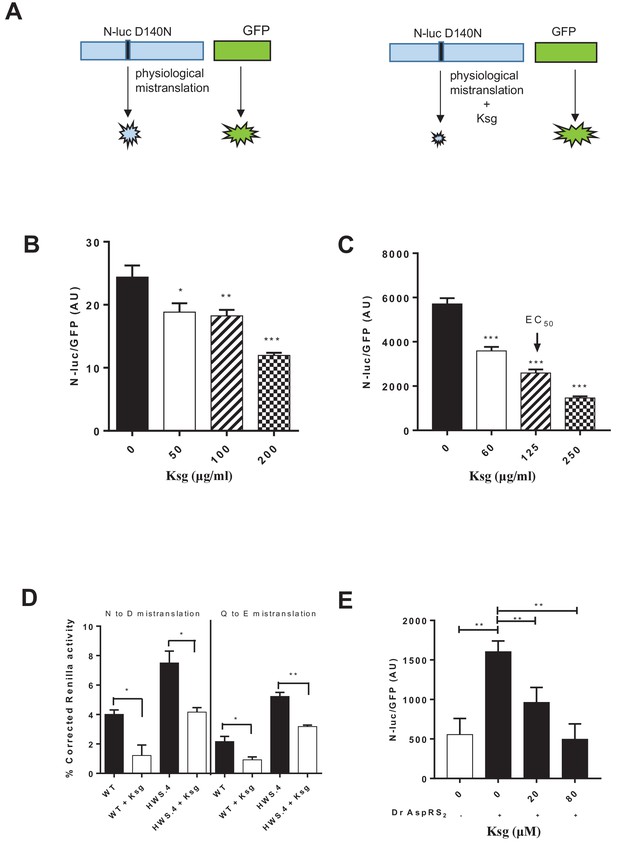
Kasugamycin decreases mycobacterial mistranslation due to the indirect tRNA aminoacylation pathway.
(A) Schematic of the Nluc-luciferase/GFP gain-of-function reporter to measure mistranslation. Kasugamycin (Ksg) decreases asparagine-to-aspartate mistranslation in both Msm (B) and Mtb (C) in a dose-dependent manner (see Materials and methods). (D) Kasugamycin (50 µg/ml) reduces asparagine-to-aspartate and glutamine-to-glutamate mistranslation – measured using a Renilla-FF luciferase dual reporter (see Materials and methods and Su et al., 2016) in a strain (HWS.4 – M. smegmatis-gatA-V405D) with a specific defect in the indirect tRNA aminoacylation pathway. (E) An E. coli cell-free translation system spiked with a non-discriminatory aspartyl synthetase used in conjunction with the Nluc-GFP mistranslation reporter shows that kasugamycin can specifically decrease translational error from misacylated Asp-tRNAAsn in a dose-dependent manner. *p<0.05, **p<0.01, ***p<0.001 by Student’s t-test.
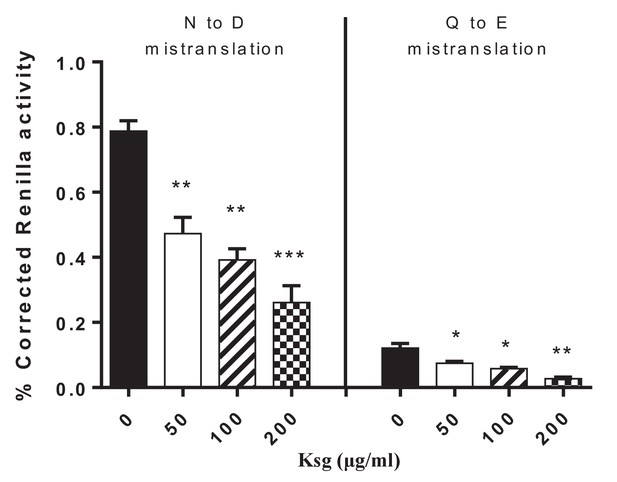
Kasugamycin decreases asparagine to aspartate and glutamine to glutamine mycobacterial mistranslation.
Mistranslation measured by gain-of-function Renilla-Firefly dual reporter as previously described: see Materials and methods and (Su et al., 2016). *p<0.05, **p<0.01, ***p<0.001 by Student’s t-test.
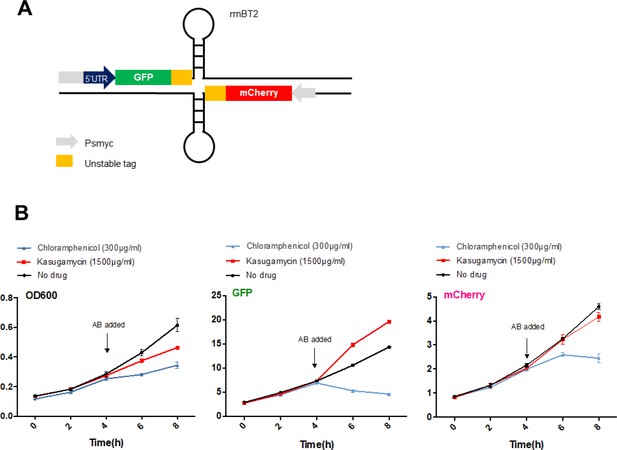
High-dose kasugamycin does not significantly inhibit translation of a canonical or leaderless transcript in M. smegmatis at doses much higher than required to reduce mistranslation.
A strain of M. smegmatis was transformed with an episomal plasmid expressing gfp from the promoter Psmyc (canonical promoter with 5’ UTR) and a leaderless version of Psmyc driving mCherry (A – schematic of the reporter). Chloramphenicol inhibited translation of both transcripts, whereas kasugamycin at 1500 µg/ml failed to significantly attenuate translation from either transcript. AB, antibiotics.
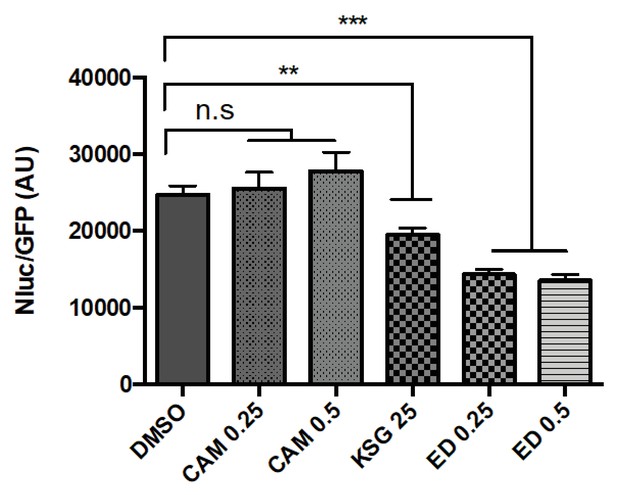
The 30S initiation inhibitors edeine and kasugamycin decrease Asp-tRNAAsn mistranslation rates.
Mistranslation was measured in Msm by the Nluc/GFP reporter as in Figure 1(A–C). SubMIC concentrations of antibiotics – chloramphenicol (CAM – 0.25–0.5 µg/ml), kasugamycin (Ksg, 25 µg/ml) and edeine (ED – 0.25–0.5 µM) were used in the assay. **p<0.01, ***p<0.001, n.s. no significant difference by Student’s t-test.
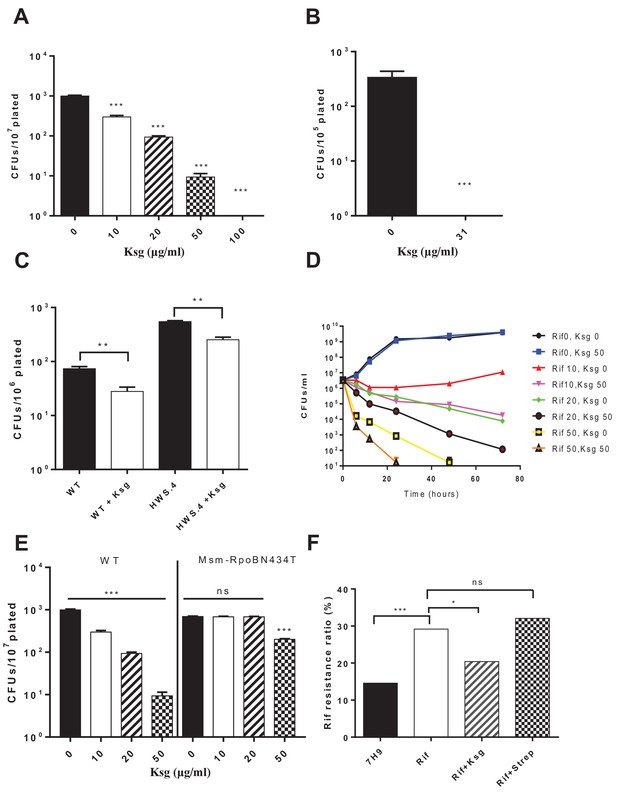
Kasugamycin enhances in vitro killing of mycobacteria by rifampicin by specifically reducing mistranslation generated by the indirect tRNA aminoacylation pathway.
Ksg decreases plating rifampicin tolerance (Su et al., 2016) in both Msm (A) and Mtb (B) and an Msm strain with a specific defect in the indirect tRNA aminoacylation pathway (C) as well as (D) increases killing of Msm by rifampicin in axenic culture. (E) A strain (M. smegmatis-RpoB-N434T) with a single point mutation in rpoB is less tolerant to rifampicin because it is unable to mistranslate via the indirect pathway a single asparagine residue critical for rifampicin binding (Su et al., 2016). This strain is, however, relatively resistant to kasugamycin’s effects on rifampicin tolerance. *p<0.05, **p<0.01, ***p<0.001 by Student’s t-test. (F) Pre-treatment of Msm cultures with low-dose rifampicin (1 µg/ml) increases likelihood of rifampicin resistance upon subsequent challenge with high-dose rifampicin (100 µg/ml). Addition of Ksg but not streptomycin in pre-treatment abolishes the increased likelihood of rifampicin resistance. *p<0.05, ***p<0.001, ns = not significant by Fisher’s exact test.
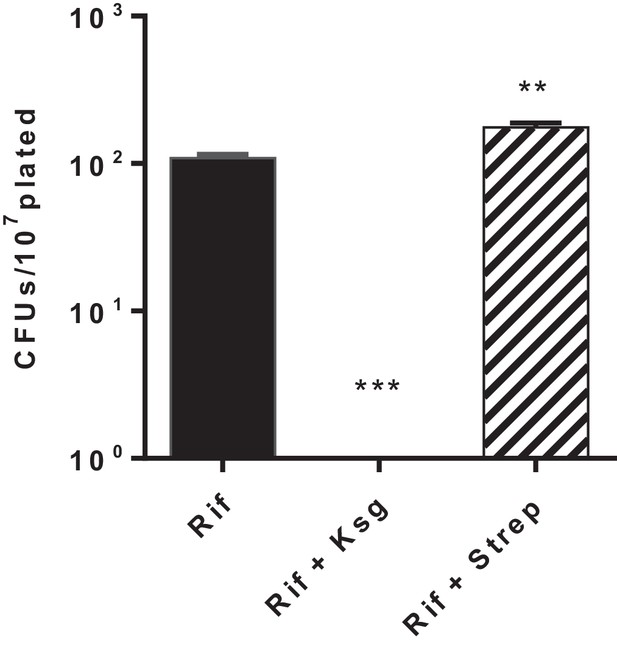
Kasugamycin but not streptomycin decreases Msm rifampicin plate tolerance.
Cells were plated on rifampicin-agar ± kasugamycin (50 µg/ml) or streptomycin (0.2 µg/ml) or LB alone. Survival fraction on rifampicin was measured after 5–7 days. **p<0.01, ***p<0.001 by Student’s t-test.
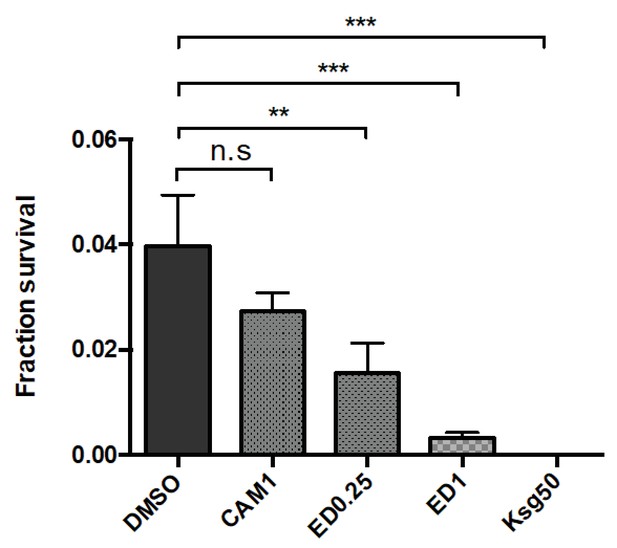
The initiation inhibitors edeine and kasugamycin decrease Msm rifampicin plate tolerance.
Cells were plated on rifampicin-agar (25 µg/ml) ±DMSO or other antibiotics as shown (edeine, ED 0.25–1 µM, chloramphenicol, CAM 1 µg/ml and kasugamycin, Ksg, 50 µg/ml). The translation inhibitors at the concentration used did not affect plating efficiency when used as a solitary agent. Survival fraction (compared with no antibiotic) was measured after 5–6 days. **p<0.01, ***p<0.001, n.s. no significant difference by Student’s t-test.
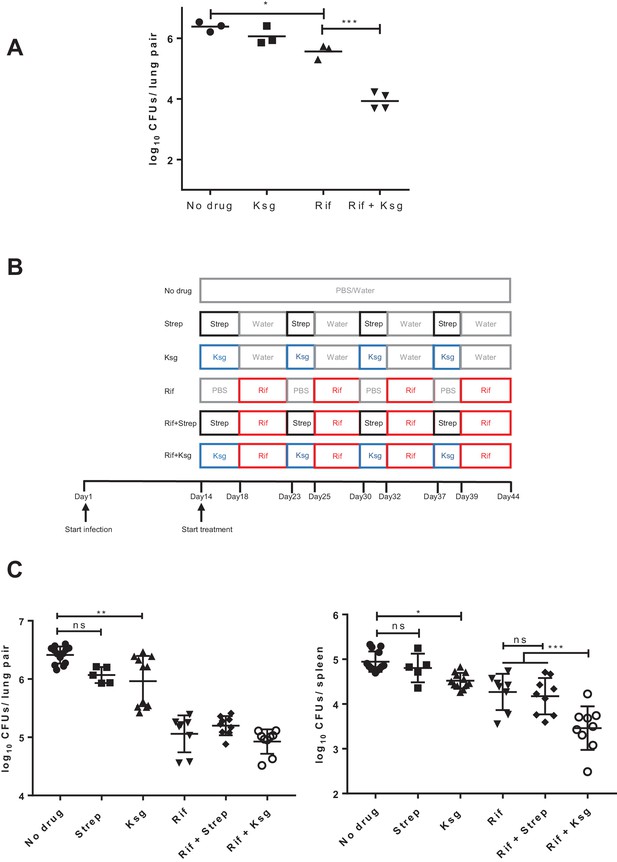
Kasugamycin enhances in vivo killing of M.tuberculosis by rifampicin.
(A) Lung burden of Mtb-infected mice treated for 2 weeks with rifampicin (10 mg/kg), Ksg (400 mg/kg) or combination of the two. (B) Schematic of the sequential Ksg (400 mg/kg)/rifampicin (10 mg/kg) or Streptomycin (3 mg/kg)/rifampicin (10 mg/kg) dosing schedule. (C) Lung (left panel) or spleen (right panel) burden of Mtb-infected mice treated for 4 weeks as per the schedule in (B). Each data point represents bacterial organ burden from a single mouse. *p<0.05, **p<0.01, ***p<0.001, ns = not significant by one-way ANOVA followed by Tukey’s post-hoc multi-comparison correction. Only key comparators are shown for clarity, complete statistical analysis is shown in Supplementary file 2.
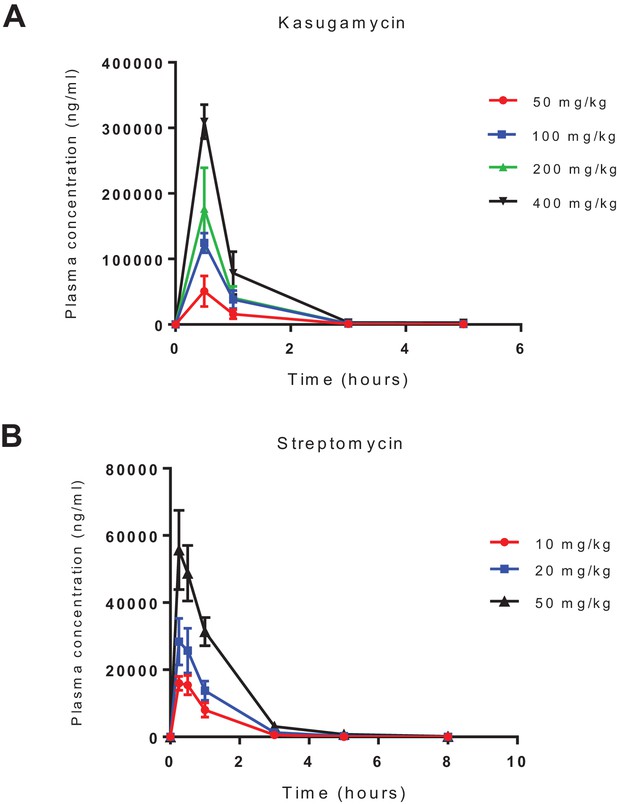
Pharmacokinetics of intra-peritoneal administration of kasugamycin and streptomycin.
Kasugamycin (top) and streptomycin were given by intra-peritoneal injection and plasma concentration measured by liquid chromatography mass-spectrometry.
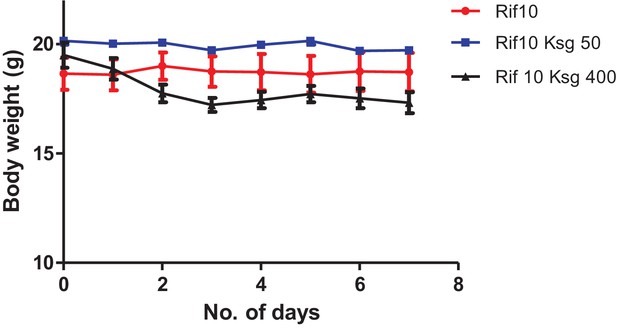
Kasugamycin-rifampicin co-administration is toxic to mice at high doses.
Mice were given ksg (intra-peritoneal injection) and rifampicin (oral gavage) daily at the indicated doses. Weight was measured daily.
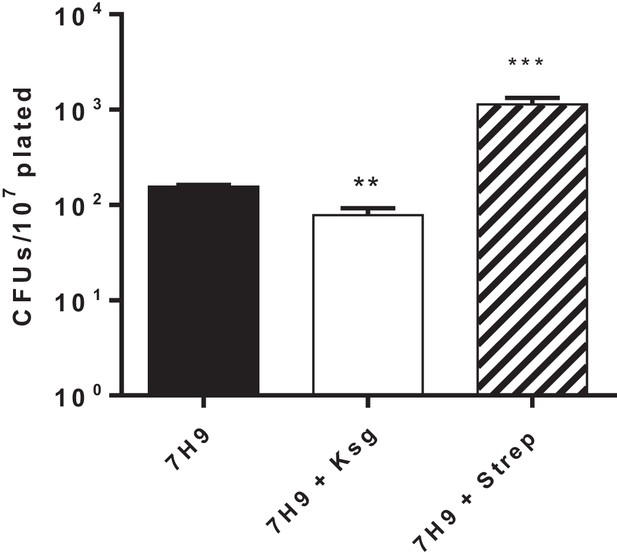
Kasugamycin but not streptomycin pre-treatment increases rifampicin susceptibility in Msm.
Msm cultures were pre-treated with ksg (50 µg/ml) or streptomycin (0.2 µg/ml) for 3 hr. Cells were then spun down, washed, resuspended and plated on rifampicin-agar or LB. Colonies were counted after 7 days.
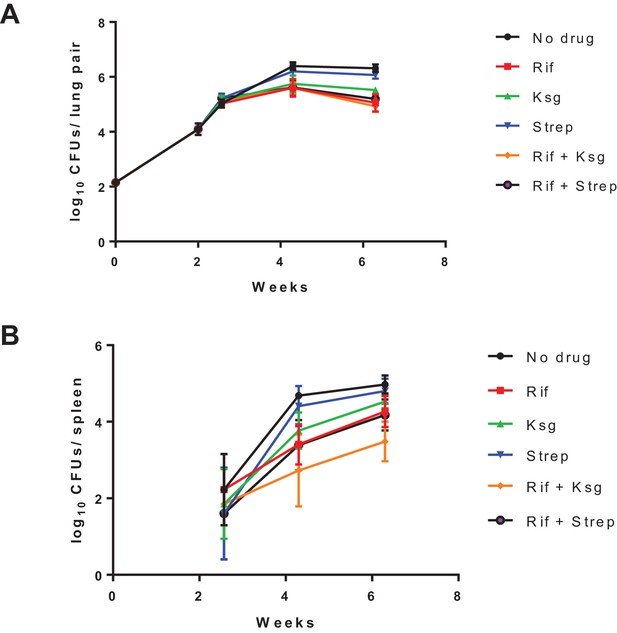
Organ burden of Mtb-infected mice treated as per the protocol in Figure 2B.
Organ burdens in lungs (left panel) and spleen (right panel) at indicated time-points shown. The data for the last point (4 weeks’ treatment) is shown in Figure 2C.
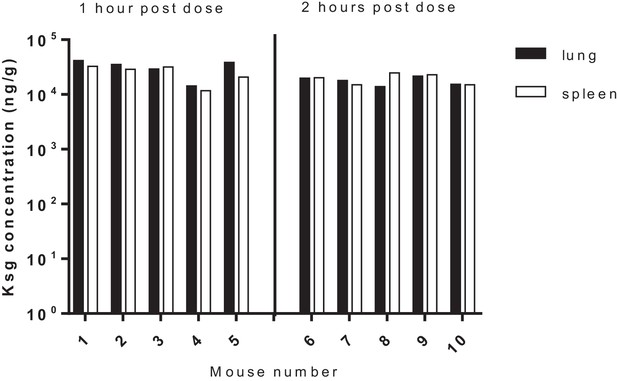
Tissue (lung/spleen) pharmacokinetics of kasugamycin following intra-peritoneal administration.
Tissue homogenates were used for PK analysis.
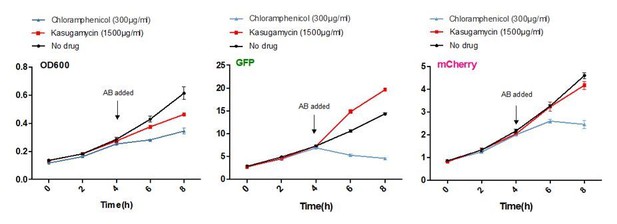
Kasugamycin does not significantly inhibit translation of a canonical or leaderless transcript in M. smegmatis at doses much higher than required to reduce mistranslation.
A strain of M. smegmatis was transformed with an episomal plasmid expressing gfp from the promoter Psmyc (canonical promoter with 5’ UTR) and a leaderless version of Psmyc driving mCherry. Chloramphenicol inhibited translation of both transcripts, whereas kasugamycin at 1500 µg/ml failed to significantly attenuate translation from either transcript.
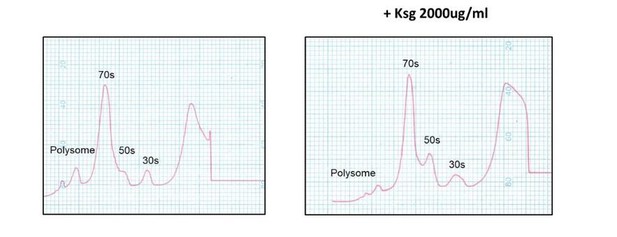
Kasugamycin does not cause formation of 61S mycobacterial ribosomes.
Ribosomal profiles of M. smegmatis ribosomes generated by sucrose gradient density centrifugation +/- kasugamycin treatment prior to isolation.
Additional files
-
Supplementary file 1
Minimum inhibitory concentration (MIC) and Cmax/MIC (maximal plasma concentration divided by MIC) of compounds used in the in vivo study.
- https://doi.org/10.7554/eLife.36782.016
-
Supplementary file 2
Detailed statistical analysis of data presented in Figure 2C
- https://doi.org/10.7554/eLife.36782.017
-
Supplementary file 3
Oligonucleotides used in this work
- https://doi.org/10.7554/eLife.36782.018
-
Transparent reporting form
- https://doi.org/10.7554/eLife.36782.019