Tuning site-specific dynamics to drive allosteric activation in a pneumococcal zinc uptake regulator
Figures
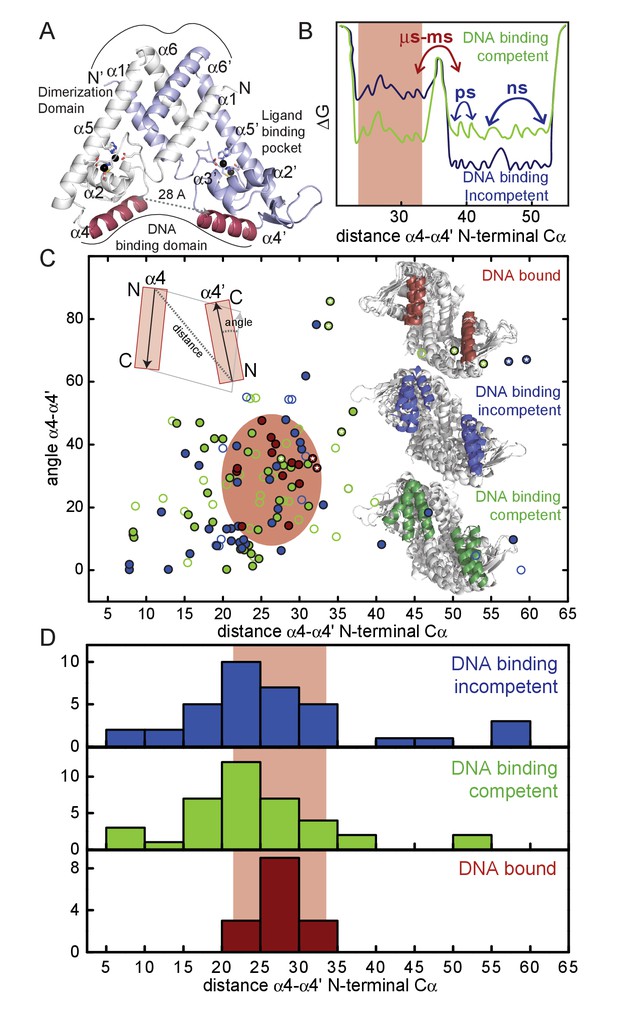
(A) Ribbon representation of dimeric Zn(II)-bound AdcR, with one protomer shaded white and the other shaded light blue (PDB code: 3tgn; Guerra et al., 2011).
The two Zn(II) ions in each protomer are represented by spheres, and coordinating ligands are shown in stick representation. The DNA binding helices are shaded red. (B) Simplified free energy diagram showing the DNA binding competent (green) and DNA binding incompetent (blue) states with the relative population of two distinct conformations: compatible with DNA binding (red rectangle, α4-α4’ distance between DNA binding helices, ≈30 Å) and incompatible with DNA binding (larger α4-α4’ distances). In this free energy diagram, the DNA binding-incompetent state has a comparatively higher population of the conformation incompatible with DNA binding relative to the DNA binding-competent state. (C) The α4-α4’ distance distribution plotted against the DNA-binding inter-helical α4-α4’ orientation distribution for all the reported MarR crystal structures (see Table 1 and Figure 1—source data 1 for details) in the allosterically DNA binding competent conformation (green), a DNA binding incompetent conformation (blue) and in the DNA-bound (red) conformation. Filled circles represent states that have been assigned based on DNA binding data, while for the hollow circles the DNA binding properties were assigned taking into account the conformational state in the crystal structure (i.e., reduced, ligand bound) and the degree of sequence similarity to other MarR repressors. The structures for ZitR and AdcR have been highlighted with a white star. The inferred conformational space occupied by the DNA-bound conformation in all MarR regulators (Table 1) is shaded in red oval. Ribbon representations of the molecules in each conformation are shown in the inset, as well as a scheme of how the distances and angles were measured. (D) Histogram plot of the α4-α4’ distance (see panel C) extracted from 136 different crystal structures of MarR repressors in the DNA binding incompetent, DNA binding competent and DNA-bound conformations.
-
Figure 1—source data 1
Table of details on MarR proteins structures: PDB ID, reference for the structure, protein state and ligand bound (if any), DNA binding competence classification, organism, Methods used for determining the DNA binding properties, DNA binding constant (Ka), reference for the DNA binding constant, residues in the α1-α2 loop obtained from pymol secondary sequence assignment, minimal distance between the α4-α4’ helices, and angle between the α4-α4’ helices.
- https://doi.org/10.7554/eLife.37268.004
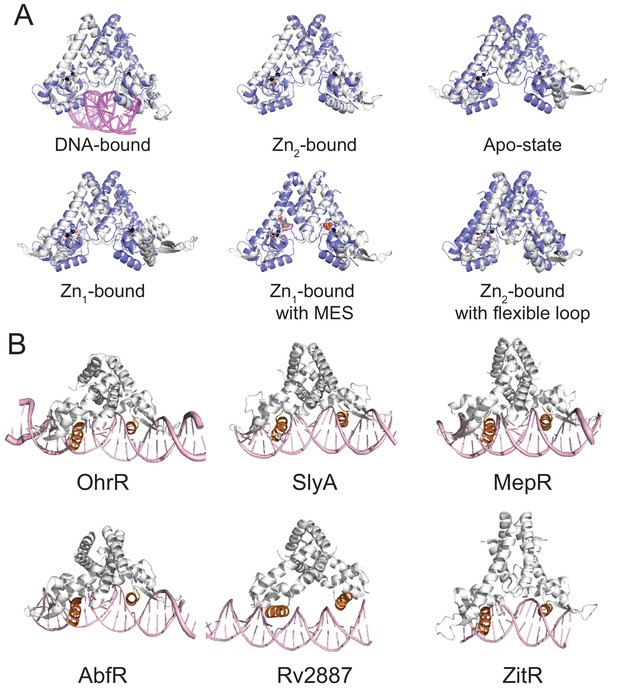
Structural comparison ZitR and AdcRs with other MarR family repressors.
(A) Structural comparison (global superposition) between AdcR (3tgn, shaded slate in all panels. (Guerra et al., 2011) and L. lactis ZitR (Zhu et al., 2017a) in the different allosteric states (DNA-bound PDB codes, 5yi2, 5yi3; ZnII2-bound, 5hyx; Apo-state, 5yi1; Zn1-bound PDB ID 5yhy, 5yl0; ZnII2-bound alternative state with a MES molecule in Zn site 1, 5yhz; Zn2-bound from Group A Streptococcus pyogenes AdcR (Sanson et al., 2015) with flexible loop, 5jls, 5lju). (B) Structural comparisons of various MarR family repressors in the DNA-bound states. (B. subtilis OhrR, PDB code, 1z9c; S. enterica SlyA, 3q5f; S. aureus MepR, 4lln; S. epidermis AbfR, 5hlg; M. tuberculosis Rv2887, 5hso; L. Lactis ZitR, 5yi2.
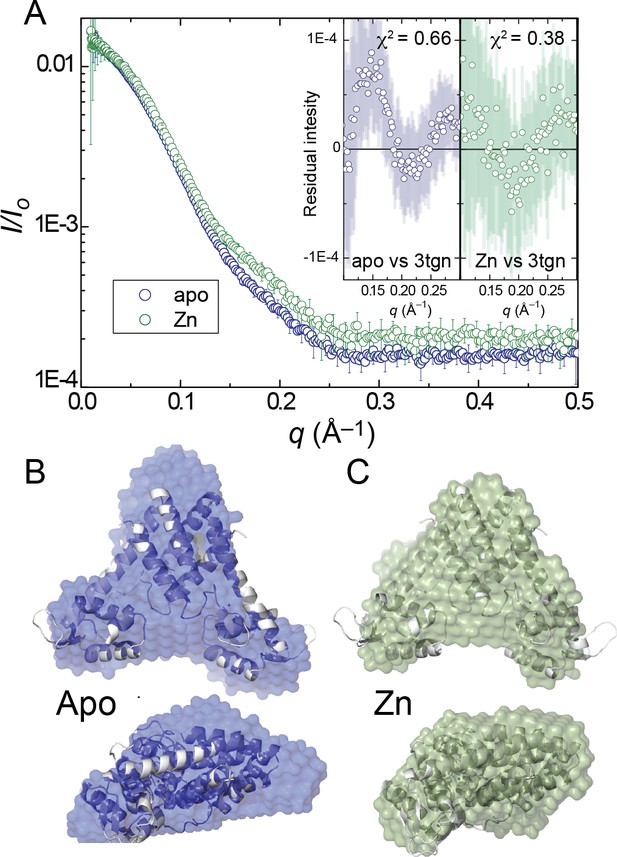
(A) Small angle X-ray scattering (SAXS) curve of AdcR in apo- and Zn2-states.
Insets present the residual intensity and χ2 estimated for the calculated scattering profile of the previously published AdcR-Zn2 structure (PDB: 3tgn) in comparison with the scattering profiles of AdcR of apo and Zn2-states (Guerra et al., 2011). Best-fit DAMMIF ab initio model (Franke and Svergun, 2009) for apo- (B) (blue) and ZnII2-states (C) (green), aligned with the ribbon representation of the ZnII2 structure (Figure 1A, PDB: 3tgn). The corresponding Guinier, Kratky and pairwise distribution histogram plots are shown in Figure 2—figure supplement 1, along with the fitting parameters.
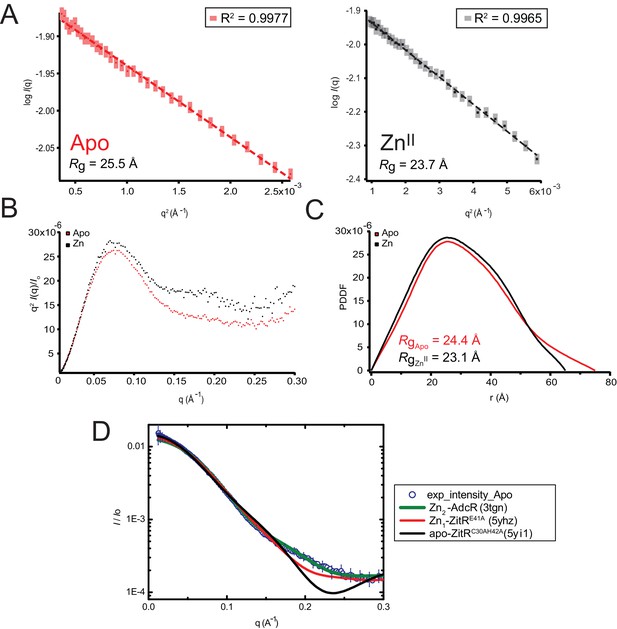
Small angle X-ray scattering (SAXS) analysis of AdcR in the apo and Zn-binding states.
(A) The Guinier region with linear fit of the scattering curve of the apo AdcR state (red) and Zn-binding state (black). Radius of gyration (Rg) of each state is presented at the low left corner. Note that scattering intensity is in arbitrary units. The linear fit of Guinier region is shown with its calculated R value. (B) Kratky plot of apo AdcR (red) and Zn-binding (black) state, normalized with I(0). (C) Pair distance distribution function (PDDF) of apo AdcR (red) and Zn-AdcR (black) states. The end-to-end distance (Dmax) of apo state is 65 Å and Dmax of the Zn-binding state is 75 Å. Rg of each state obtained from PDDF is also presented. (D) calculated scattering profiles of crystal structures of apo ZitRC30AH42A (5yi1), Zn1-bound ZitRE41A (5yhz) (Zhu et al., 2017a) and Zn-binding state of AdcR (3tgn) compared to the experimental scattering profile of apo-AdcR.
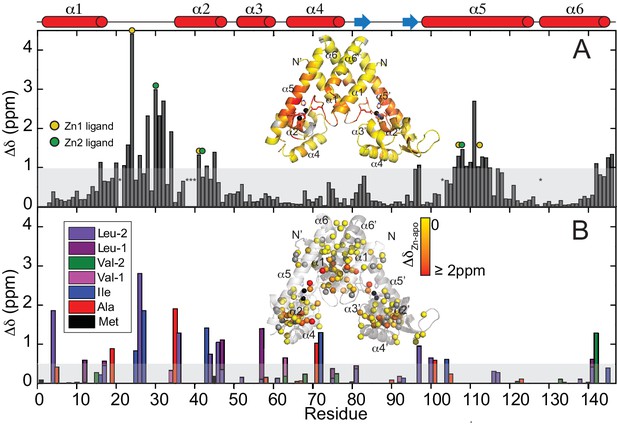
Chemical shift perturbation (CSP) maps for ZnII binding to to AdcR.
(A) Backbone CSPs. CSPs of the sterospecifically assigned methyl groups at pH 5.5, 50 mM NaCl, 35°C. (B) Both these CSPs are painted on the ribbon representation of the structure of ZnII2 AdcR. The shaded bar in each case represents one standard deviation from the mean perturbation. Site 1 and site 2 ligands in the primary structure in panel A are denoted by the yellow and green circles, respectively; the asterisks at residue positions 21 and 38 – 40 indicate no assignment in the apo-state (see materials and methods), while asterisks mark residue positions 103 and 128 for prolines. Insets show the CSP values painted onto the 3tgn structure.
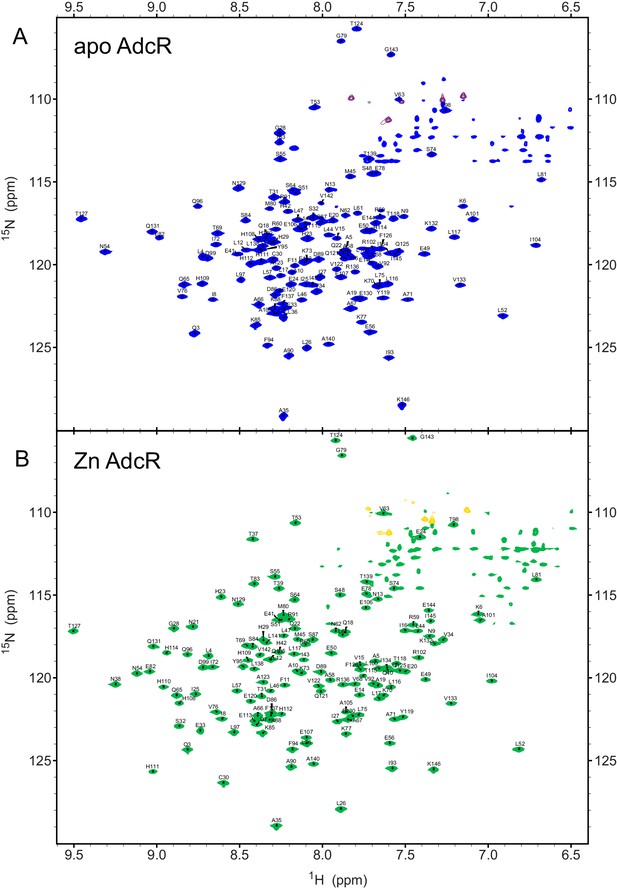
Backbone 1H-15N TROSY spectra showing the assignments of (A) apo WT AdcR and (B) ZnII WT AdcR.
https://doi.org/10.7554/eLife.37268.009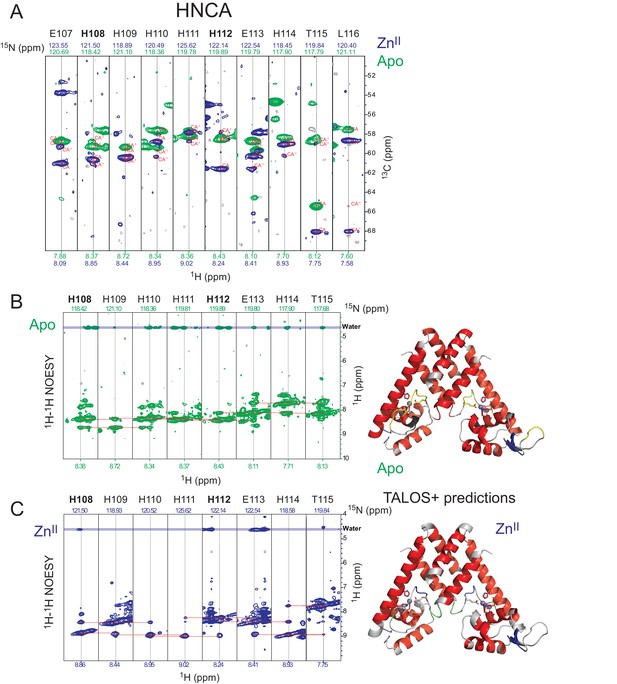
NMR analysis of the α5 helix in AdcR.
(A) Sequential residue-specific connectivities that link the chemical shifts of the 13Cα resonances in the α5 helix (E107-L116; H108, H112 ZnII ligands in bold) from an HNCA experiment. (B) (C) 1H,15N NOESY-HSQC strips obtained from the same region of the α5 helix in the apo- (B) and ZnII2 (C) states. Right, TALOS +predictions in the apo- (top) and ZnII2 (bottom) states. Despite fully α-helical predictions, the apo-state is characterized by weaker i, i + 2 NH-NH correlations, and stronger NOEs (as solvent exchange crosspeaks) to water, relative to the ZnII state. This is consistent with a more highly dynamic α5 helix in the apo-state.
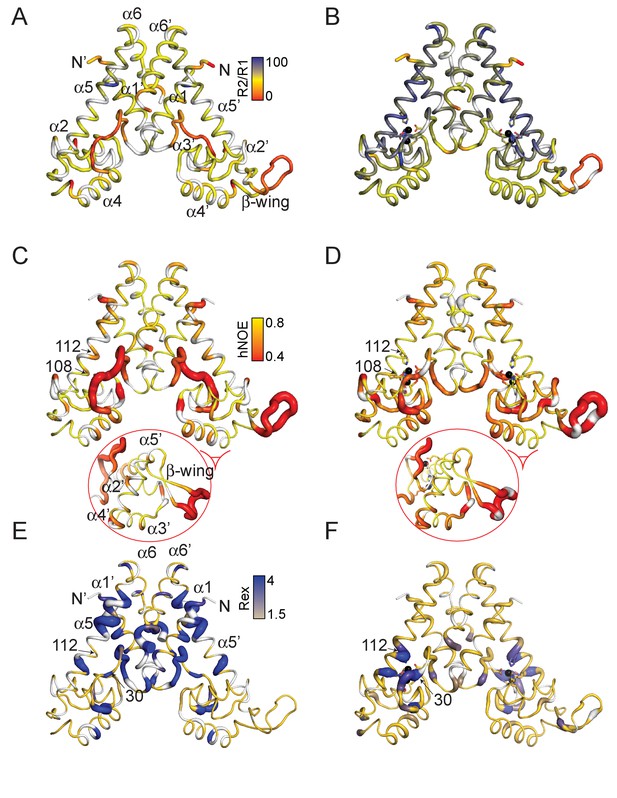
Dynamical characterization of the apo- (A) (C) (E) and ZnII2 (B) (D) (F) AdcR conformational states.
Backbone 1H-15N amide R2/R1 for apo- (A) and ZnII2 AdcR (B) painted onto the 3tgn structure (Guerra et al., 2011). Heteronuclear NOE analysis of apo- (C) and ZnII2 (D) AdcR with the values of the 15N-{1H}-NOE (hNOE) painted onto the 3tgn structure. Values of Rex determined from HSQC 15N-1H CPMG relaxation dispersion experiments at a field of 600 MHz for the apo- (E) and ZnII2 (F) AdcRs (see Figure 4—figure supplement 3 for complete data sets). Similar results were obtained at 800 MHz. ZnII ions are shown as black spheres and residues excluded due to overlap are shown in gray. The width of the ribbon reflects the value represented in the color bar.
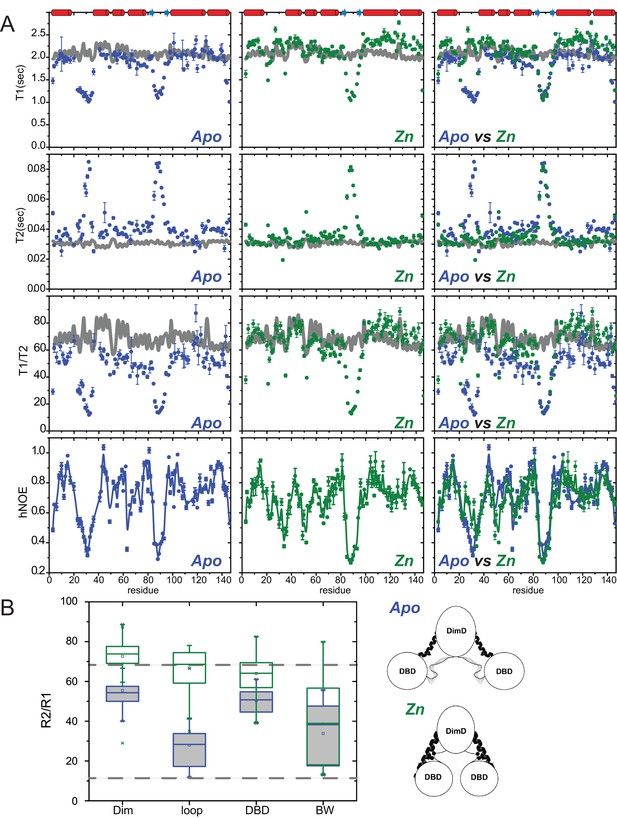
Backbone 15N spin relaxation analysis of AdcR.
(A) Experimental values of relaxation times and associated uncertainties for WT AdcR in apo-state (left, blue), ZnII2-bound state (middle, green) and overlay of both allosteric states (right) at 800 MHz with respect to the protein sequence from top to bottom: T1, T2, T1/T2 and hNOE. The position of the secondary structure elements is shown above the top panel. The grey line in the T1, T2, T1/T2 panels represent the predicted values obtained from hydroNMR for the ZnII2-AdcR crystal structure (3tgn). (B) Average backbone amide 1H-15N relaxation parameters R2/R1 for the apo- (blue boxes) and ZnII2- (green boxes) states of the AdcR homodimer in different regions of the molecule: DimD, dimerization domain (residues 5 – 20, 101 – 144); Zn-loop (α1-α2 loop, residues 21 – 37); DBD, DNA binding domain (residues 38 – 101, excluding the β-wing; β-wing (residues 81 – 101). The dashed line around 68 represent the average value predicted from hydroNMR for the ZnII2-AdcR crystal structure, while the dashed line at three represent the value obtained from the DNA binding domain on its own. Cartoon representations of the data shown in panel (B) in which the two linkers that connect the two domains (middle of the α5-helix, dark coil; α1-α2 loop, light pencil) are more dynamic in the apo-state. Note that residues analogous to the α1-α2 loop in AdcR are not observed in the crystal structure of the apo-state of L. lactis ZitR (Zhu et al., 2017b) (see Figure 1—figure supplement 1A), consistent with these findings in solution in apo-AdcR.
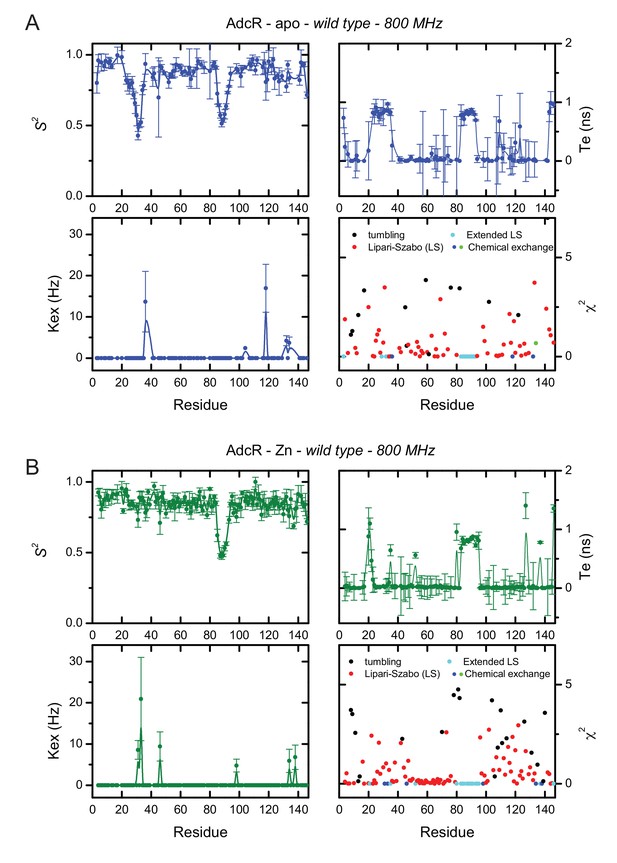
Dynamic parameters obtained for (A) apo- and (B) ZnII2-WT AdcR using tensor 2.
These parameters assume an anisotropic rotational diffusion tensor: (top, left) amide order parameter, S2, (top, right) internal correlation time, (bottom, left) phenomenological chemical exchange contribution, (bottom, right) error function for the corresponding model determined for each residue.
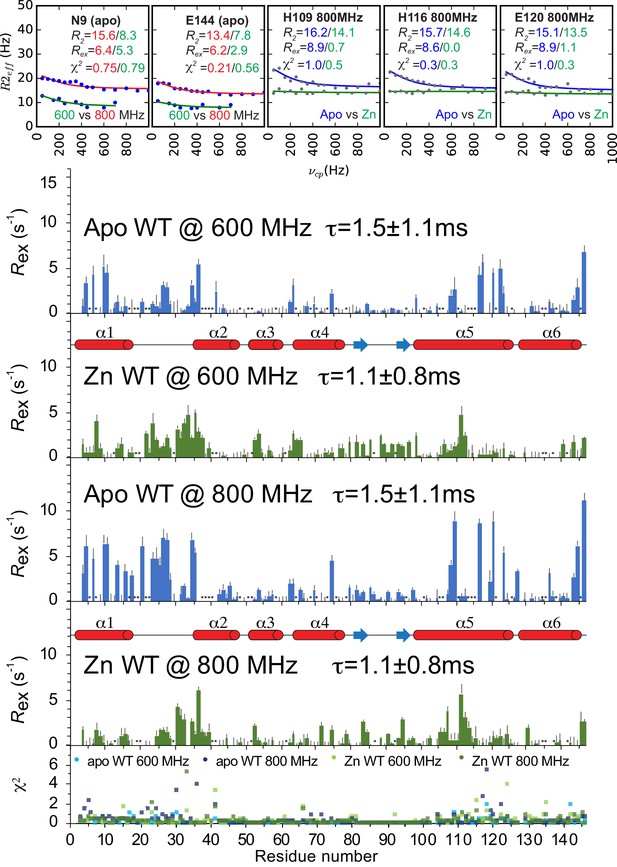
Backbone (NH) relaxation dispersion analysis of AdcR.
Representative raw relaxation dispersion curves obtained for the indicated backbone (NH) used to obtain Rex at 600 MHz and 800 MHz. Rex for both allosteric states are shown for data acquired at 600 and 800 MHz, as indicated. All the residues excluded due to overlap are shown with an asterisk (Backbone Apo: 5, 7, 16, 18, 19, 22, 48, 50, 51, 58, 64, 68, 70, 73, 75, 78, 95, 100, 105, 106, 110, 113, 114, 115, 121, 125, 130, 135, 138, 145; Backbone Zn-bound: 12, 18, 19, 29, 40, 41, 51, 61, 86, 92, 134, 135, 137, 141, as are the unassigned residues 21,37–40 for apo and the prolines 103,128 for both states). Other residues are omitted due to poor overall fit, as indicated by χ2, shown in the bottom panel. Error bars show error of the fit for Rex.
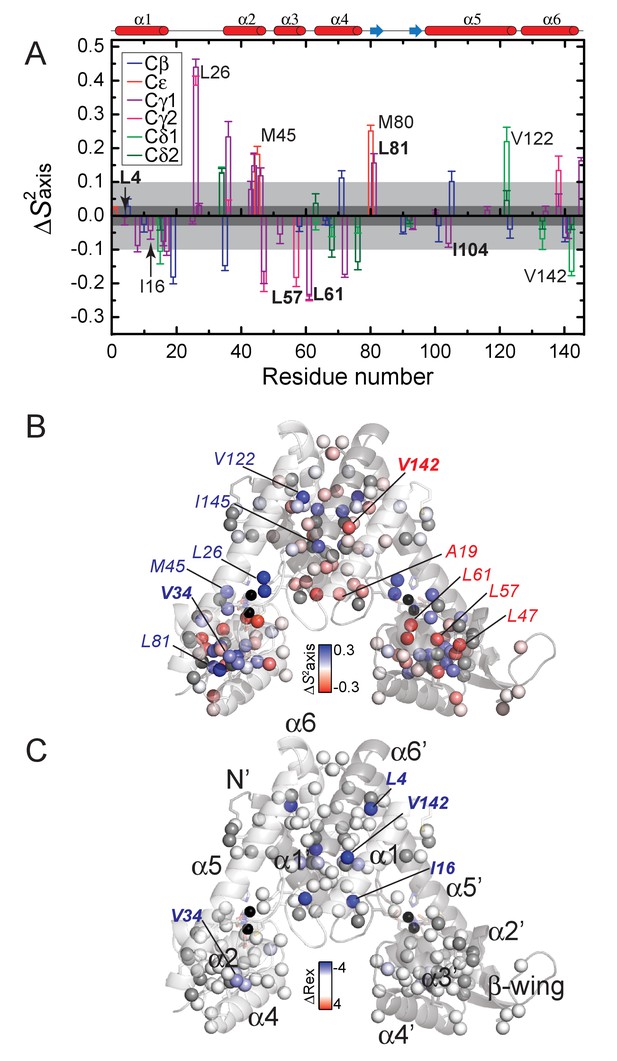
Effect of ZnII binding to AdcR on the site-specific stereospecifically assigned methyl group axial order parameter.
(A) Difference in axial order parameter (∆S2axis = S2axisZn–S2axisapo) between apo- and ZnII2-states, with the specific type of methyl group color-coded as indicated: Cβ, Ala; Cε, Met; Cγ1, Cγ2, Val; Cδ1, Cδ2, Leu. The dark shaded region shows no significant difference between apo- and Zn-II2-bound states and the lighter shaded region represents the cutoff for ‘dynamically active’ residues. S2axis (B) and Rex (C) plotted as ∆S2axis (S2axisZn – S2axisapo) and ∆Rex (RexZn – Rexapo) values, respectively, mapped onto the structure of ZnII2 AdcR (3tgn). A ∆S2axis <0 indicates that the methyl group becomes more dynamic in the ZnII2-bound state, while ∆Rex <0 indicates quenching of motion on the µs-ms timescale in the in the ZnII2-bound state. See Figure 5—figure supplements 1 and 2 for a graphical representation of all S2axis and Rex values in each conformation from which these differences were determined, respectively. Residues harboring methyl groups that show major dynamical perturbations on ZnII binding are highlighted, with selected residues subjected to methyl substitution mutagenesis (Figure 6).
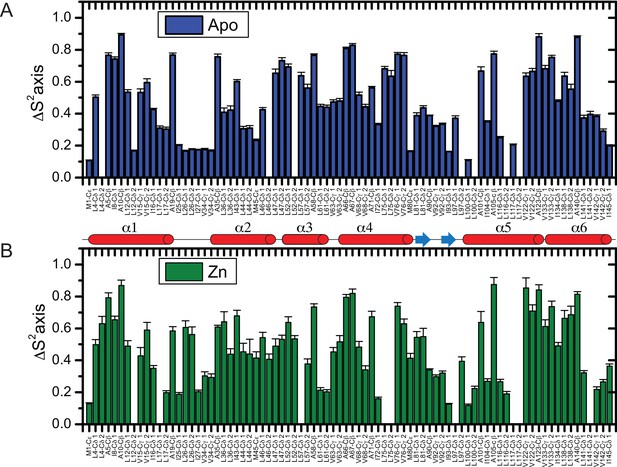
Methyl group axial order parameter analysis of AdcR.
Stereospecific methyl group axial order parameters, S2axis, for apo-(A) and ZnII2- (B) AdcRs as measured at 600 MHz (similar results were obtained at 800 MHz; data not shown). Errors in S2axis were obtained by Monte Carlo simulation where a Gaussian-distributed random number generator was used to generate simulated datasets by generating random values for the ratios at each delay value according to a distribution dictated by the error propagated from spectral noise (Source code 1).
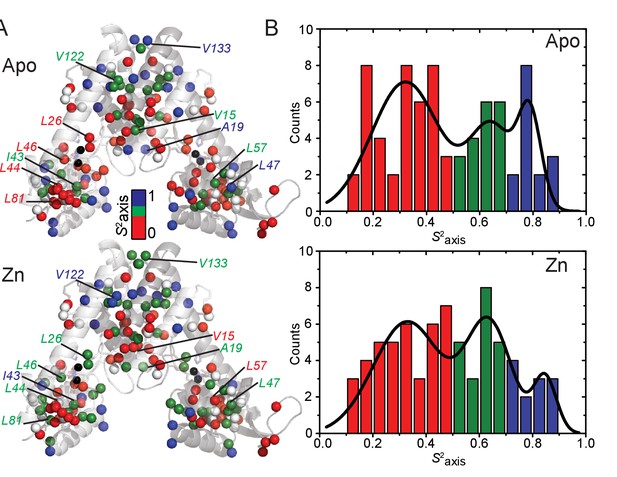
Absolute values of methyl group order parameters, S2axis on the methyl-bearing residues.
(A) Absolute values, and (B) Histogram plot of S2axis from fitting the apo (top) and ZnII2 (bottom) states in panel (A) calculated according to (Marlow et al., 2010).
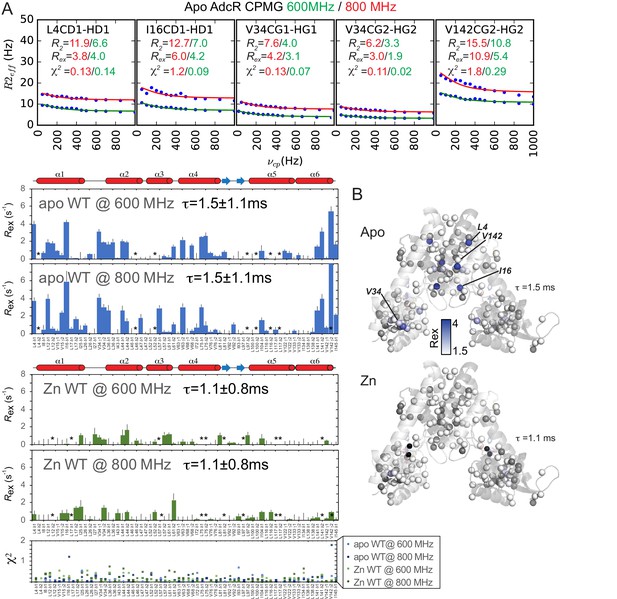
Methyl group relaxation dispersion analysis of AdcR.
Representative raw relaxation dispersion curves obtained for the indicated methyl group used to obtain Rex at 600 MHz and 800 MHz (A). Rex for both allosteric states at 600 and 800 MHz are shown in panels below. All the residues excluded due to overlap are shown with an asterisk (sidechain apo: L4-δ2, L46-δ2, L52-δ2, L97-δ2, L100-δ2, L116-δ2, L117-δ2; sidechain Zn-bound: L12-δ2, L17-δ1,L57-δ1, L75-δ1, L75-δ2, L81-δ2, L97-δ1, L117-δ1, L117-δ2, L141-δ2). V142-γ2 is also marked with an asterisk and used with caution for apo at 800 MHz due to poor overall fit, as indicated by χ2, shown in the bottom panel. Error bars for Rex show error of the fit for Rex. Panel B shows the Rex values for the apo and Zn-bound states plotted on the 3tgn structure.
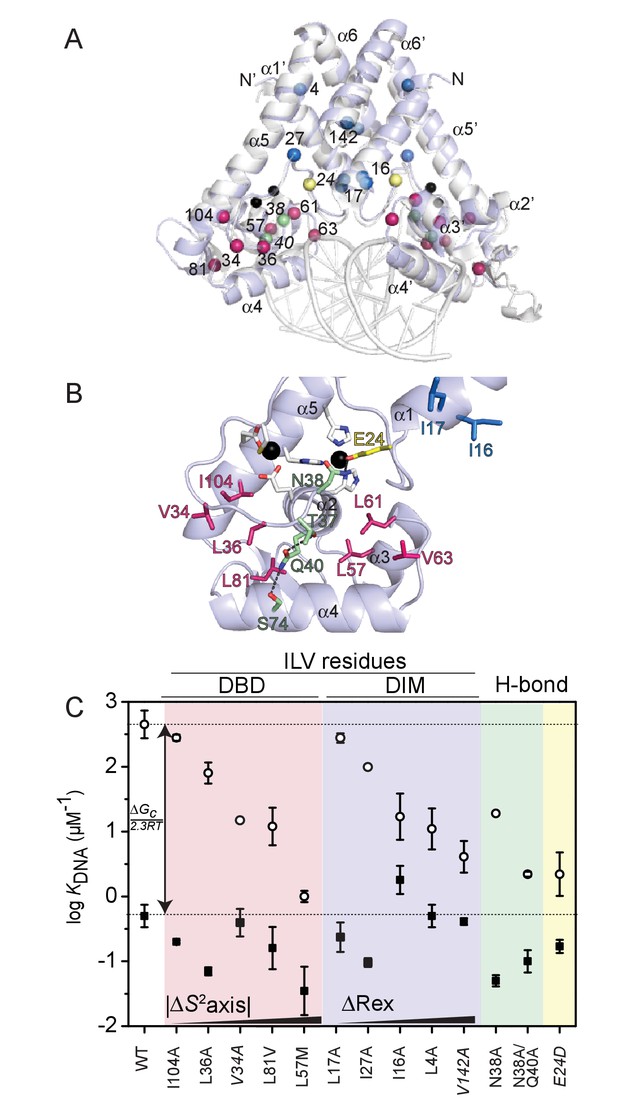
Graphical summary of the functional properties of AdcR methyl substitution and hydrogen bonding mutants.
(A) Cα positions of the residues targeted for methyl substitution mutagenesis in the DNA binding domain (DBD) (red spheres) and in the dimerization domain (DIM) (blue spheres); other residues targeted for substitution in the hydrogen-bonding pathway (N38, Q40; green spheres) and zinc ligand E24 (yellow spheres) highlighted on the structure of the ZnII2 ZitR-DNA operator complex (Zhu et al., 2017c); ZnII ions (black spheres). (B) Zoom of the DNA binding domain (DBD) of one of the two ZnII2-bound AdcR protomers highlighting the residues targeted for mutagenesis (methyl substitution mutants, red stick; hydrogen-bonding pathway mutants, green stick; zinc ligand E24, yellow stick), with the helical elements (α1-α5) indicated. (C) Coupling free energy analysis for all AdcR mutants highlighted using the same color scheme as in panels A and B. DBD, DNA-binding domain; DIM, dimerization domain; H-bond, hydrogen binding mutants. KDNA for apo-AdcRs are shown in fill circles; KDNA for ZnII2 -AdcRs are shown in hollow circles. Lower horizontal line, KDNA for wild-type apo-AdcR; upper horizontal line, KDNA for wild-type ZnII2 AdcR, for reference. The trend in ∆S2axis and ∆Rex is qualitatively indicated (see Table 2). These residues are conserved to various degrees in AdcR-like repressors (Figure 6—figure supplement 5).
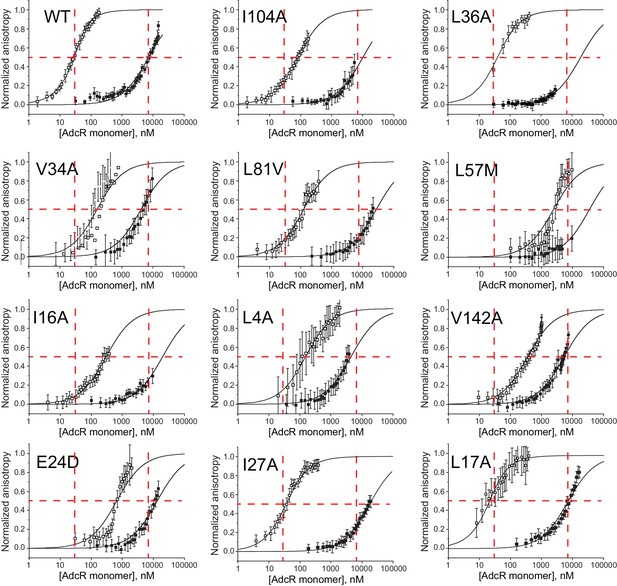
Representative DNA operator binding isotherms obtained for wild-type (WT) AdcR and selected AdcR mutants in the apo- and ZnII2-states.
The continuous lines through each set of data correspond to nonlinear least squares fit to a 1:1 non-dissociable AdcR dimer binding model, with parameters compiled in Supplementary file 1-Table S1, and ∆Gc shown graphically in Figure 6C (main text). The red vertical and horizontal lines represent the AdcR monomer concentrations that correspond to 50% DNA-saturation points for the wild-type AdcR under the same solution conditions, presented as a guide only. Conditions: 10 mM Hepes, pH 7.0, 0.23 M NaCl, 1 mM TCEP (chelexed), 10 or 20 nM DNA, 25.0°C with 1.0 mM EDTA (for apo).
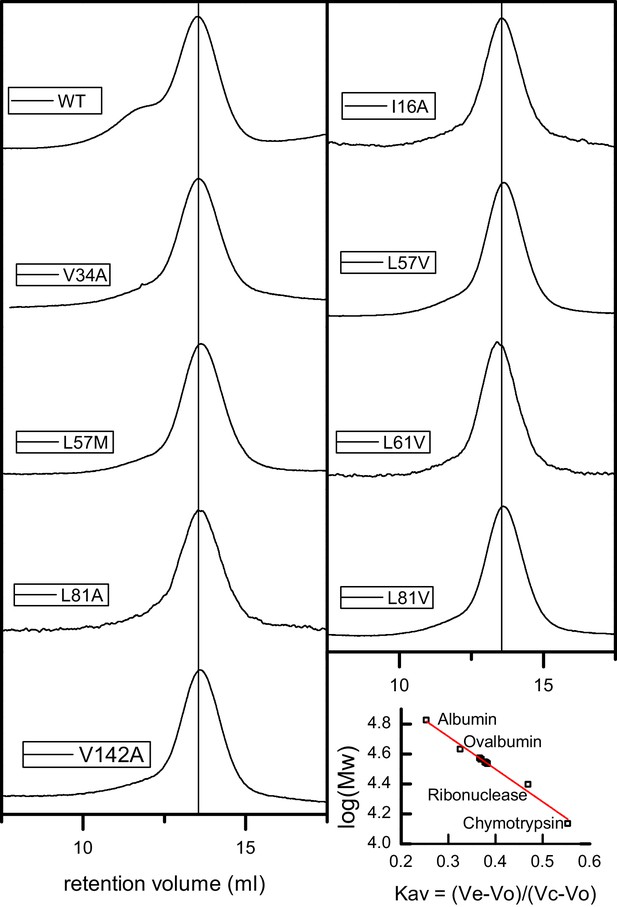
Gel filtration chromatograms for AdcR variants in the apo-state.
Lower right, calibration curve with standards (empty squares) and AdcR variants (filled squares).
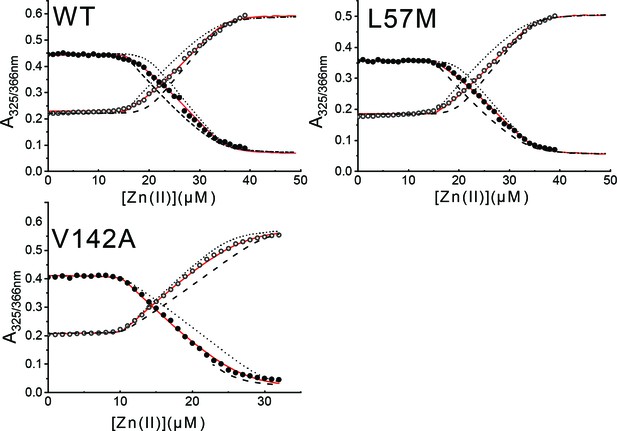
Representative ZnII-binding isotherms obtained from a titration of apo (metal-free) wild-type AdcR or a mutant AdcR and mag-fura-2 (mf2) with ZnSO4.
ZnII binding parameters for these and other AdcRs are compiled in Supplementary file 1-Table S1. Open symbols represent the 505 nm emission resulting from 324 nm excitation; filled symbols, 366 nm excitation; red lines represent data fit; black lines represent simulated curves corresponding to one order of magnitude lower (solid) or higher (dashed) KZn3 and KZn4. Experiments were conducted in triplicate for each AdcR variant.
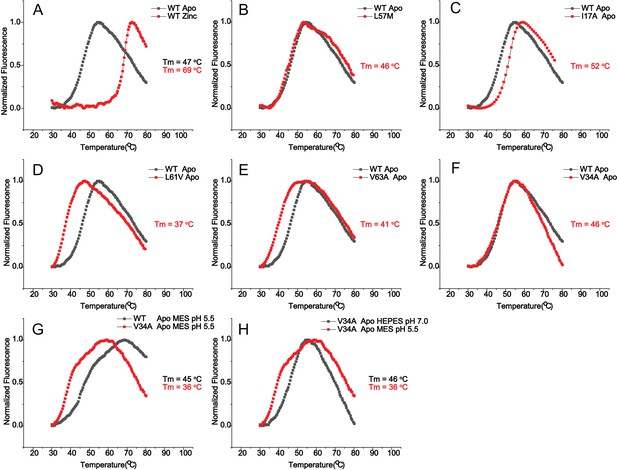
Differential scanning fluorimetry (DSF) of AdcR and mutants Representative DSF plots acquired with SYPRO orange.
(A) Wild-type AdcR in apo- (black) and ZnII2-states (red). (b–f) wild-type AdcR in the apo state overlaid with selected mutants in the apo state. (g) Isotopically labeled wild-type AdcR overlaid with isotopically labeled V34A AdcR, both at pH 5.5. (h) V34A AdcR at pH 7.0 overlaid with isotopically labeled V34A AdcR mutants, at pH 5.5. Tm values from triplicate measurements are compiled in Supplementary file 1-Table S2.
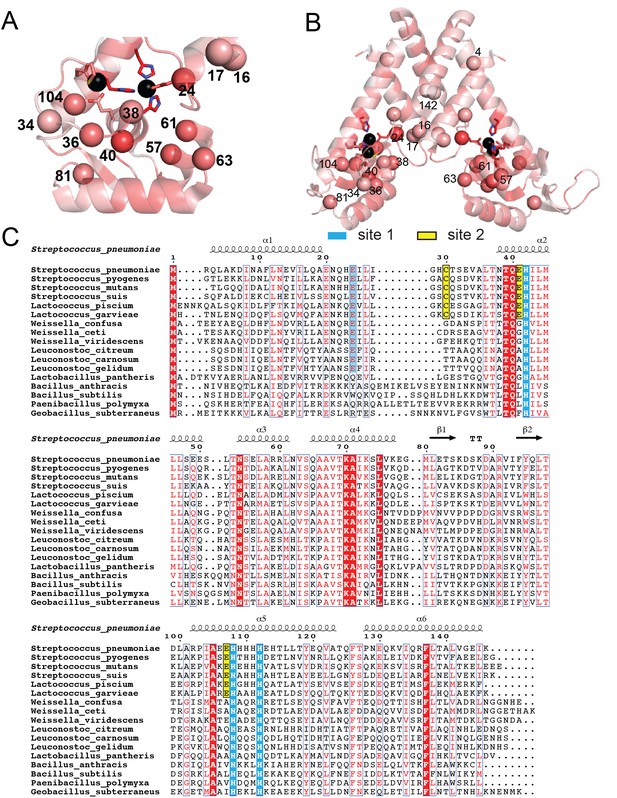
Multiple sequence analysis of AdcRs and closely related MarR family repressors.
Amino acid sequence conservation of S. pneumoniae AdcR and candidate closely related MarR family repressors. Sequence conservation highlighting those residues targeted for mutagenesis in this work with a Cα sphere on the ZnII2 AdcR structure (Guerra et al., 2011) in the DNA binding domain (A) and the entire molecule (B). The ribbon structure shows the degree of conservation by ramping the color from white to bright red, with those residues of high conservation shaded bright red, using Protskin (Ritter et al., 2004). For reference, ZnII ligands are invariant (100% conserved). (B) Multiple sequence analysis of the 17 AdcR-like repressors used to create the sequence conservation map.
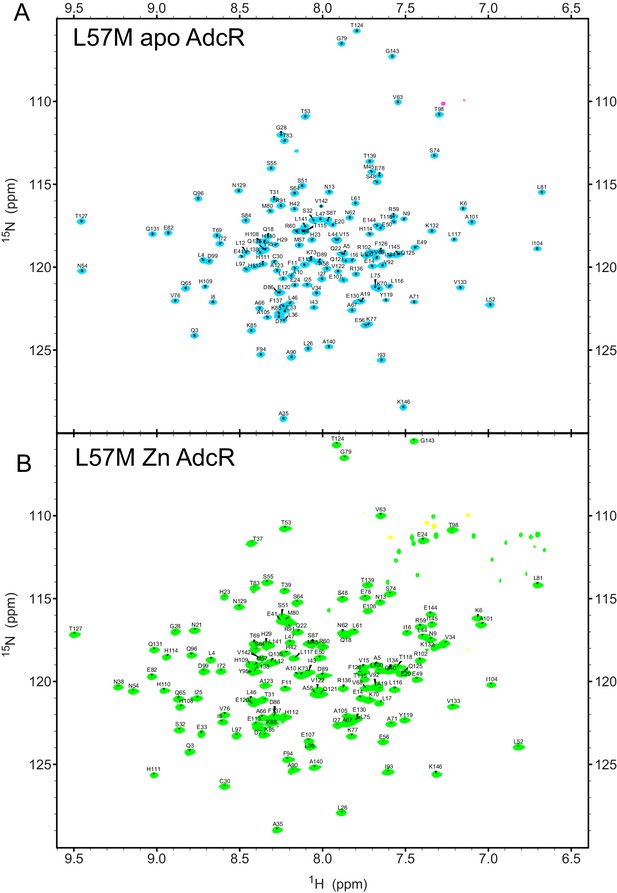
Backbone 1H-15N TROSY spectra showing the resonance assignments of (A) apo L57M AdcR and (B), ZnII L57M AdcR.
https://doi.org/10.7554/eLife.37268.025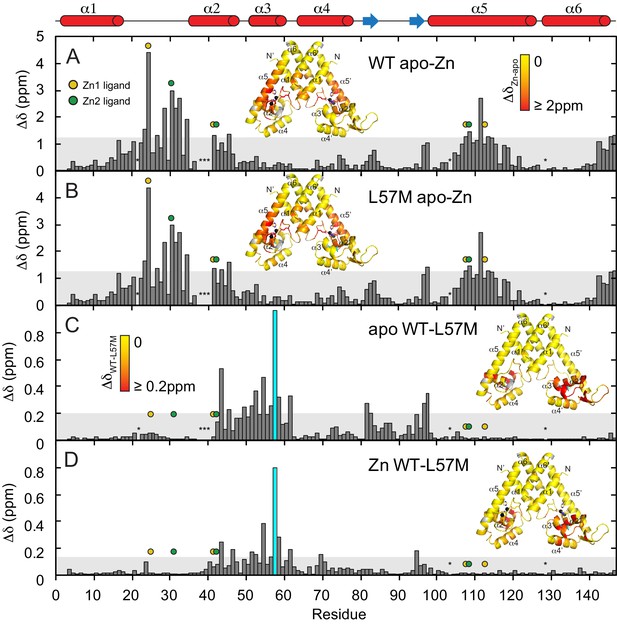
Backbone chemical shift perturbation (CSP) maps for L57M AdcR. Apo-L57M AdcR and for ZnII2 L57M AdcR at pH 5.5, 50 mM NaCl, 35°C relative to WT AdcR as indicated.
ZnII binding to WT AdcR is repeated for reference in panel (A) ZnII binding to L57M AdcR is shown in panel (B) CSPs of the mutation are shown for apo in panel (C) and ZnII2-bound AdcR in panel (D) CSPs are painted on the ribbon representation of the structure of ZnII2 AdcR. The shaded bar in each case represents one standard deviation from the mean perturbation. Site 1 and site 2 ligands in the primary structure are denoted by the yellow and green circles, respectively; the asterisks at residue positions 21 and 38 – 40 indicate no assignment in the apo-state, while asterisks at positions 103 and 128 denote prolines. For panels (C) and (D), the CSP of the mutated residue L57M is indicated with a cyan bar, and L57 is shown as cyan sticks on the painted cartoon.
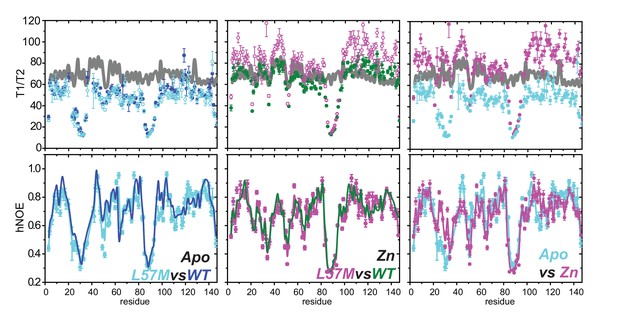
15N NMR spin relaxation analysis of L57M vs. wild-type AdcR.
Experimental values of relaxation times and associated uncertainties for L57M AdcR in apo-state (left, blue), ZnII2-bound state (middle, green) and overlay of both allosteric states (right) at 800 MHz with respect to the protein sequence T1/T2 (top) and hNOE (bottom). The position of the secondary structure motifs is shown above the top panel. The grey line in the T1/T2 panel represent the predicted values obtained from hydroNMR for ZnII2-AdcR crystal structure (3tgn) at 35°C, radius of atomic element of 3.2 Å and the corresponding viscosity for 10% D2O (0.7192). Apo and ZnII2-WT AdcR results are represented in blue and green lines for comparison.
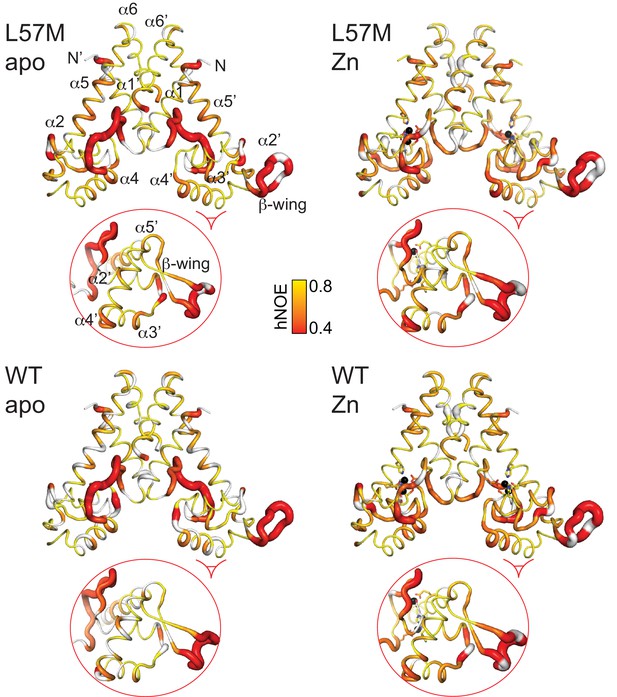
Heteronuclear NOE analysis of L57M vs. wild-type AdcR. Apo- and ZnII2 L57M AdcR values of the 15N-{1H}-NOE (hNOE) are painted onto the 3tgn structure, relative to the WT parameters reproduced here to facilitate comparison.
The ovals highlight a region of the DNA binding domain where the L57M and WT dynamics differ from one another.
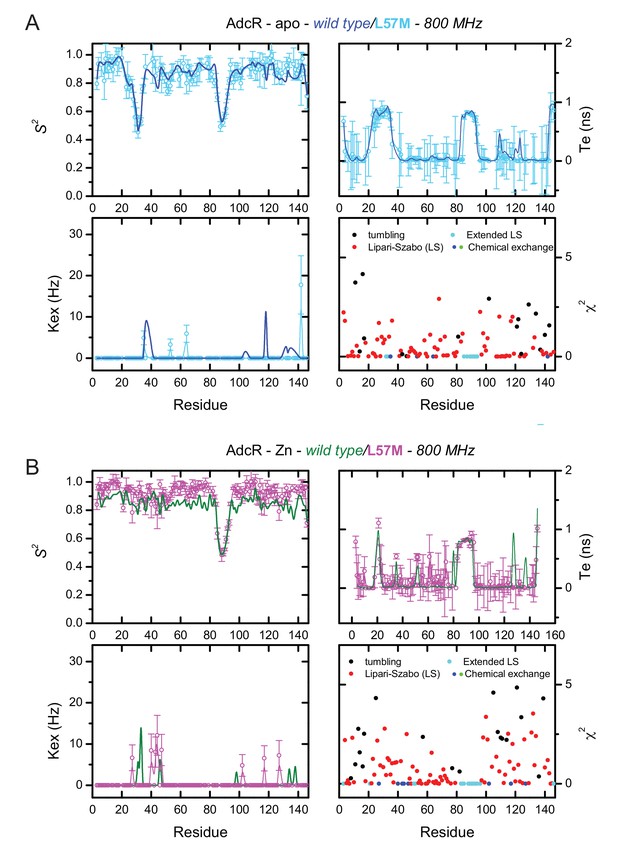
Backbone dynamics parameters obtained for L57M vs. wild-type AdcRs.
Dynamics parameters odtained with tensor2 for (A) apo- and (B) ZnII2-L57M AdcR assuming an anisotropic rotational diffusion tensor: (top, left) amide order parameter, S2, (top, right) internal correlation time, (bottom, left) phenomenological chemical exchange contribution, (bottom, right) error function for the corresponding model determined for each residue. Apo and ZnII2-WT AdcR results are represented in blue and green continuous lines for comparison.
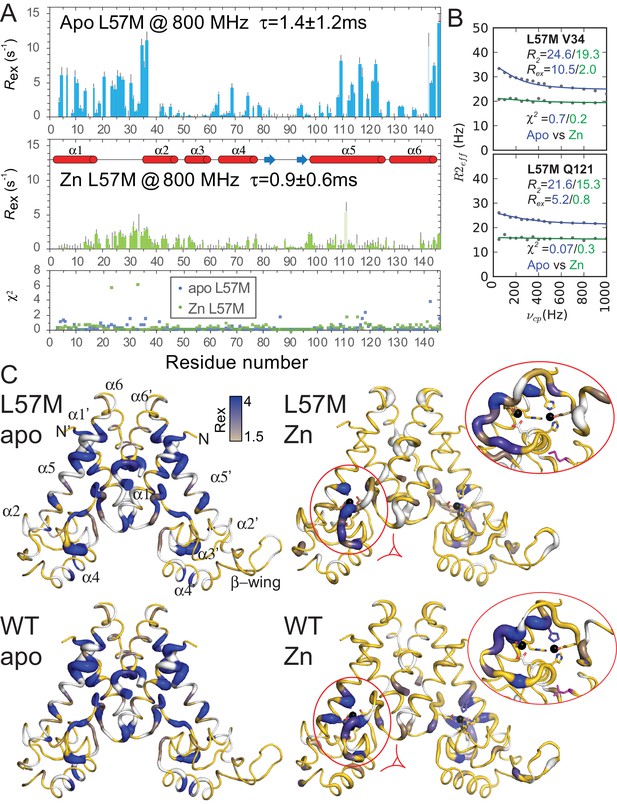
Backbone relaxation dispersion analysis of the L57M vs. wild-type AdcRs.
Values of Rex determined from 15N-1H TROSY CPMG relaxation dispersion experiments at 800 MHz for the apo- and ZnII2 L57M AdcR are shown in panel A. Residues 5, 7, 15, 19, 22, 44, 56, 70, 75, 77, 86, 88, 95, 106, 115, 120, 125, 130, 135, and 145 are omitted due to resonance overlap for the apoprotein, while residues 12, 19, 20, 29, 41, 45, 58, 75, 80, 86, 87, 95, 130, 135, 137, 138, and 141 are omitted due to overlap for the Zn-bound state, as are the proline residues 103 and 128 from both charts. Residue 142 is omitted due to poor fit for the apo state, while residues 23, 33, 111, and 115 are omitted due to poor fit for the Zn-bound state, as indicated by the χ2 values. Representative relaxation dispersion curves are shown in panel B, including the reduced χ2 values indicating the quality of the fits. Note that Apo L57M is prone to significant aggregation over multiple days at 35°C and pH 5.5, thus leading to decreased signal intensities relative to reference spectra, and thus the unexpectedly high apparent R2eff values observed in these relaxation dispersion curves. Panel C shows the values of Rex painted onto the 3tgn structure, relative to the WT parameters reproduced below to facilitate comparison. The ovals expand and highlight a region of the DNA binding domain where the L57M and WT dynamics differ from one another. The L57 side chain in shown in magenta, in stick representation.
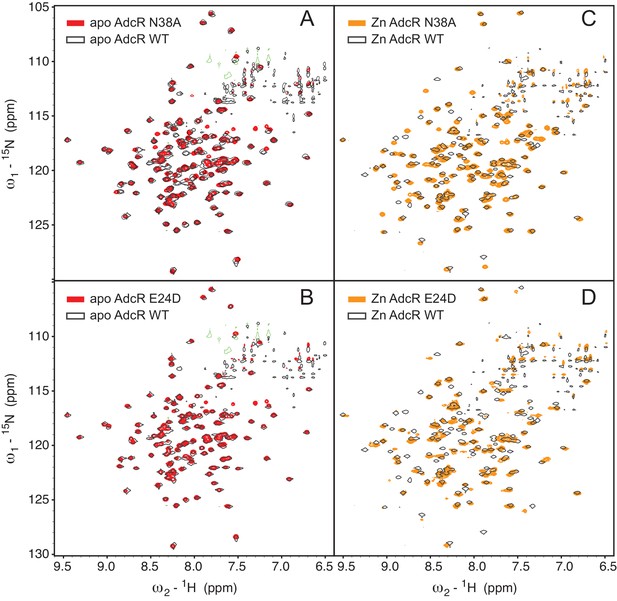
TROSY NMR spectra of selected AdcR mutants.
2D 1H,15N TROSY spectra of apo- (left) and ZnII2 (right) states of N38A and E24D AdcRs, compared to the wild-type AdcR (black contour; single contour line shown only) acquired under the same solution conditions (50 mM NaCl, pH 6.0, 35°C).
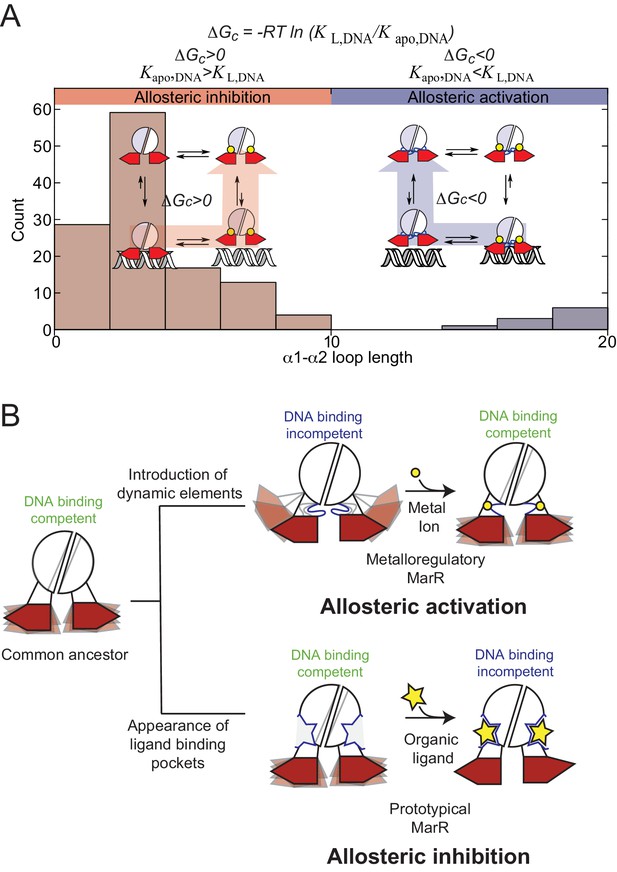
(A) Histogram representation of the distribution of α1-α2 loops lengths in the reported structures in MarR family of proteins, with the bars are colored to account for the measured or proposed coupling free energies in each case.
Proteins that are DNA binding-competent in the apo- state and DNA binding-incompetent in the ligand-bound state are colored in red, while proteins that are DNA binding-incompetent in the apo-state and DNA binding-competent in the liganded state are colored in blue (see Figure 1—source data 1 for a full accounting of these structures). A schematic representation of allosteric inhibition and activation are shown (inset), with shorter α1-α2 loops associated with allosteric inhibition of DNA binding upon ligand binding, while longer loops are associated with allosteric activation (like that for AdcR/ZitR) upon ligand binding. (B) Dynamically driven model for how conformational dynamics can be harnessed to evolve allosteric activation (upper right) vs. allosteric inhibition (lower right) in the same molecular scaffold. This model suggests that dynamic properties of the DNA binding competent states have been conserved to give rise to a more favorable conformational entropy. In the metalloregulatory MarRs (AdcR, ZitR), the inactive state shows perturbed dynamics over a range of timescales; apo-AdcR therefore exhibits low affinity for DNA. Metal ion (yellow circle) coordination quenches both local and global modes in the dimerization domain and linkers, while inducing conformational disorder in the DNA-binding domain that enhances DNA binding affinity, thus stabilizing a conformation that has high affinity for DNA and giving rise to a favorable conformational entropy. For prototypical MarRs, where the ligand (yellow star) is an allosteric inhibitor, ligand binding narrows the conformational ensemble to a DNA-binding incompetent conformation decreasing the enthalpic contribution to DNA binding.
Tables
Interprotomer distances between the Cα of the N-terminal residue in the α4 and α4’ helices for representatives MarR proteins
https://doi.org/10.7554/eLife.37268.005DNA-bound state | DNA binding incompetent statea,* | DNA binding competent stateb* | ||||
---|---|---|---|---|---|---|
MarR | Distance (Å) | Pdb id | Distance (Å) | Pdb id | Distance (Å) | Pdb id |
ZitR (AdcR) | 32.3/31.7 | 5yi2/5yi3 | 59.6/57.3 | 5yh0/5yh1 | 35.5/54.0/50.2 (22.2/34/33.8) | 5yhx/5yhy/ 5yhz (3tgn/5jls/5 jlu) |
Ec MarR | 29 | 5hr3 | 12.9/12 | 1jgs/4jba | 8.3/8.4 | 3vod/3voe |
OhrR | 27.6 | 1z9c | (32.2) | (2pfb) | 23.9 (28.9) | 1z91 (2pex) |
SlyA | 27.8 | 3q5f | 29.4 | 3deu | 15.5 (23.8, 20) | 3qpt (1lj9, 4mnu) |
AbsC | 26.3 | 3zpl | 30.8 | 3zmd | - | - |
RovA | 21.8/21.9 | 4aij/ 4aik | - | - | 20.9 | 4aih |
MosR | 25.1 | 4f×4 | 15.1 | 4f×0 | - | - |
MepR | 26.4/26.9 | 4lll/ 4lln | 18.9/16.9/ 30.8/57.9 | 3eco/4l9n/ 4l9t/4l9v | 27.9/46.8 | 4l9j/4ld5 |
AbfR | 29.9/30 | 5hlh/5hlg | 40.7 | 5hli | 37 | 4hbl |
Rv2887 | 22.5 | 5hso | 7.9/15.1 | 5hsn/5hsl | 8.3 | 5hsm |
HcaR | 28.6 | 5bmz | 19.1/19.8/19.5/19.2 | 4rgx/4 rgu/4rgs/ 4rgr | 18.7 | 3k0l |
ST1710 | 10.1c | 3gji | 23 | 3gf2 | 22.8 | 2eb7 |
TcaR | 19.1d | 4kdp | 22.3/24.7 | 4eju/3kp7 | 26.4/22.5/21.1/22/27.6/ 18.3/21.1/18.2 | 3kp2/3kp3/3kp4/3kp5 /3kp7/4ejt/4ejv/4ejw |
-
aAny protein allosteric state that has been shown to bind to DNA in-vitro with an affinity higher than 107 M-1 or is capable of repressing the expression of downstream gene.
bAny protein allosteric state that fails to repress these genes and/or exhibits a significantly lower DNA binding affinity from the DNA binding-competent conformation (at least 10-fold) or an affinity lower than 106 M-1 *In addition to these two categories, two other categories were classified as DNA binding-competent or DNA binding-incompetent states in Figure 1C. They refer to any protein allosteric state for which the DNA binding properties have not been determined, but the conformational state in the crystal structure is known (i.e., reduced, ligand bound).
-
cNot inserted in the major groove of the DNA.
dThis structure was co-crystallized with ssDNA. Any entry in parentheses corresponds to a structure of a homologue from a different organism (see Figure 1—source data 1).
DNA binding parameters for wild-type AdcR and substitution mutants*
https://doi.org/10.7554/eLife.37268.032ZnII | Dynamic changes (ZnII) at 600 MHz | |||||
---|---|---|---|---|---|---|
AdcR | Kapo,DNA (x106 M−1) | KZn, DNA (x106 M−1) | ΔGc (kcal mol−1) | ΔS2axis | ΔRex | Fractional ASA† |
wild-type | 0.5 ± 0.2 | 450 ± 220 | –4.0 ± 0.6 | |||
I104A | 0.20 ± 0.01 | 280 ± 30 | –4.3 ± 0.4 | −0.08 ± 0.01 | −0.3 ± 0.6 | 0.04 |
L36A | 0.07 ± 0.01 | 80 ± 30 | –4.1 ± 0.4 | 0.13 ± 0.10 | −2.0 ± 0.5 | 0.05 |
V34A | 0.37 ± 0.17 | 13 ± 1 | –2.0 ± 0.3 | 0.13 ± 0.02 | −2.0 ± 0.5 | 0.46 |
L81V | 0.16 ± 0.12 | 12 ± 8 | –2.4 ± 0.6 | 0.13 ± 0.05 | 0.0 ± 0.5 | 0.00 |
L61V** | - | - | - | −0.23 ± 0.01 | −1.0 ± 0.5 | 0.01 |
L57M | 0.035‡ ± 0.030 | 1 ± 0.2 | –2.0 ± 0.7 | −0.18 ± 0.02 | 1.0 ± 0.5 | 0.00 |
L57V** | <0.05§ | <0.05§ | N/A | −0.18 ± 0.02 | 1.0 ± 0.5 | 0.00 |
I16A | 1.8 ± 0.9 | 17 ± 14 | –1.8 ± 0.4 | −0.08 ± 0.02 | −4.0 ± 1.0 | 0.11 |
L4A | 0.5 ± 0.2 | 11 ± 8 | –1.8 ± 0.3 | 0.004 ± 0.045 | −4.0 ± 1.0 | 0.01 |
V142A | 0.41 ± 0.05 | 4.1 ± 2.3 | –1.4 ± 0.2 | −0.09 ± 0.02 | −3.0 ± 1.0 | 0.31 |
I27A | 0.09 ± 0.01 | 80 ± 3 | –4.0 ± 0.2 | 0.03 ± 0.01 | 1.2 ± 0.5 | 0.07 |
L17A | 0.22 ± 0.1 | 219 ± 36 | –4.0 ± 0.2 | −0.10 ± 0.02 | 0.0 ± 0.5 | 0.50 |
V63A** | - | - | - | 0.01 ± 0.04 | 1.0 ± 0.5 | 0.24 |
N38A | 0.05 ± 0.01 | 19 ± 10 | –3.5 ± 0.7 | –# | – | – |
N38A/Q40A | 0.10 ± 0.04 | 2.2 ± 0.4 | –1.9 ± 0.2 | – | – | – |
E24D | 0.17 ± 0.04 | 2.2 ± 1.7 | –1.6 ± 0.3 | – | – | – |
-
*Conditions: 10 mM Hepes, pH 7.0, 0.23 M NaCl, 1 mM TCEP (chelexed), 10 nM DNA, 25.0°C with 2.0 mM EDTA (for apo-AdcR) or 20 μM ZnCl2 (for ZnII2 AdcR) added to these reactions. See Figure 6C, for a graphical representation of these data. All ∆Gc values lower than −3.5 kcal mol-1, with the exception of N38A AdcR are statistically significantly different (p≤0.1) from the wild-type ∆Gc value.
†Accessible surface area (ASA) was calculated from the ZnII2-bound AdcR (Guerra et al., 2011) using the web server for quantitative evaluation of protein structure VADAR 1.8 (vadar.wishartlab.com/)
-
‡Upper limit on measureable Kapo,DNA under these solution conditions.
§ Weaker than upper limit.
-
#Not measurable using the NMR experiments employed here.
**Significantly lower thermal stability as estimated by differential scanning fluorimetry (Supplementary file 1-Table S2) prevented a quantitative analysis of their DNA and metal binding affinities.
Additional files
-
Supplementary file 1
Supplementary table for wild-type AdcR and selected AdcR mutants zinc binding affinities and melting temperatures from differential scanning fluorimetry.
- https://doi.org/10.7554/eLife.37268.034
-
Source code 1
Python script for determining S2axis values.
Representative python script used to analyze sets of peaklists from Sparky (Lee et al., 2015) to compute ratios of peak heights and to fit the peak height ratios to η values in order to determine S2axis values for sidechain methyls of AdcR. Spectral noise must be input for propagation to errors in the peak height ratios, from which Monte Carlo simulation is used to obtain an error estimate for S2axis.
- https://doi.org/10.7554/eLife.37268.035
-
Source code 2
Python script for determining relaxation parameters for two state model.
Representative python script used to read in a table of peak heights as output from the sparky ‘rh’ or ‘Relaxation Peak Heights’ command, where the number of cycles has been entered as the time parameter. This script calculates R2eff for each peak at each number of cycles, then uses these values to fit R2 and Rex, given a fixed τe, specified in s. Output consists of Excel spreadsheets giving fitted parameters and errors in fitting, as well as PDF charts of each fit and Rex values for all probes.
- https://doi.org/10.7554/eLife.37268.036
-
Source code 3
Python script for finding τ for obtaining relaxation parameters.
Representative python script used to read in a table of peak heights as output from the sparky ‘rh’ or ‘Relaxation Peak Heights’ command, where the number of cycles has been entered as the time parameter. This script calculates R2eff for each peak at each number of cycles, then uses these values to fit τe, R2 and Rex for each probe. Output consists of Excel spreadsheets giving fitted parameters and errors in fitting, as well as PDF charts of each fit and Rex values for all probes.
- https://doi.org/10.7554/eLife.37268.037
-
Source code 4
Dynafit script DNA binding isotherms.
Representative Dynafit script file used for nonlinear least squares fitting of the fluorescence anisotropy-derived DNA binding isotherms to a dimer linkage model, in which AdcR monomers-dimer equilibrium is displaced to dimers (P2) (defined by Kdimer >> protein concentration), which are competent to bind a single AdcO with an affinity Ka. Units of [concentrations] and [constants] in this script are nM and nM−1, respectively.
- https://doi.org/10.7554/eLife.37268.038
-
Source code 5
Dynafit script ZnII-binding isotherms.
Representative Dynafit script file used for nonlinear least squares fitting of the ZnII-binding isotherms obtained from a titration of apo (metal-free) wild-type AdcR or a mutant AdcR and mag-fura-2 (mf2) with ZnSO4. Units of [concentrations] and [constants] in this script are µM and µM-1, respectively.
- https://doi.org/10.7554/eLife.37268.039
-
Transparent reporting form
- https://doi.org/10.7554/eLife.37268.040