Lipoate-binding proteins and specific lipoate-protein ligases in microbial sulfur oxidation reveal an atpyical role for an old cofactor
Figures
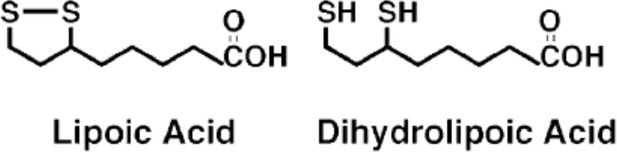
Structures of lipoic acid and its reduced derivative dihydrolipoic acid.
https://doi.org/10.7554/eLife.37439.002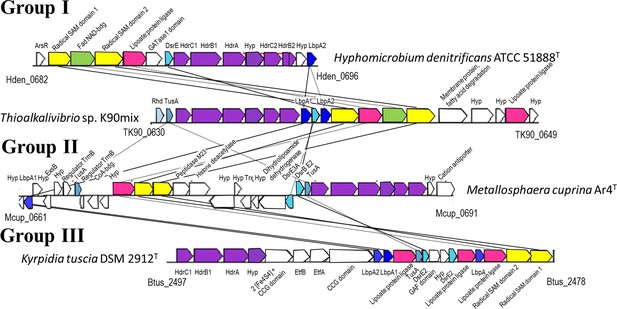
Representative operon structures/gene clusters from organisms containing hdr-like and lbpA genes.
Homologous genes are colored the same between organisms. Genes in white are not conserved between the groups. The Kegg/NCBI locus tag identifiers for the first and last genes are shown below each cluster. GATase1, glutamine amidotransferase class I; DsrE, -E2, -E3A, DsrE-like sulfurtransferases (Dahl, 2015); Rhd, rhodanese-like sulfurtransferase (Bordo and Bork, 2002); Hyp, hypothetical protein; TusA, TusA-like sulfur carrier protein (Dahl, 2015; Dahl et al., 2013); CCG domain, domain with CCG signature motif of non-cubane [4Fe-4S] cluster-binding heterodisulfide reductase active sites (Wagner et al., 2017); Etf, electron-transferring flavoprotein (Garcia Costas et al., 2017).
-
Figure 2—source data 1
Occurrence of hdr-like, lbpA and lbpA maturation genes in genome-sequenced prokaryotes.
- https://doi.org/10.7554/eLife.37439.005
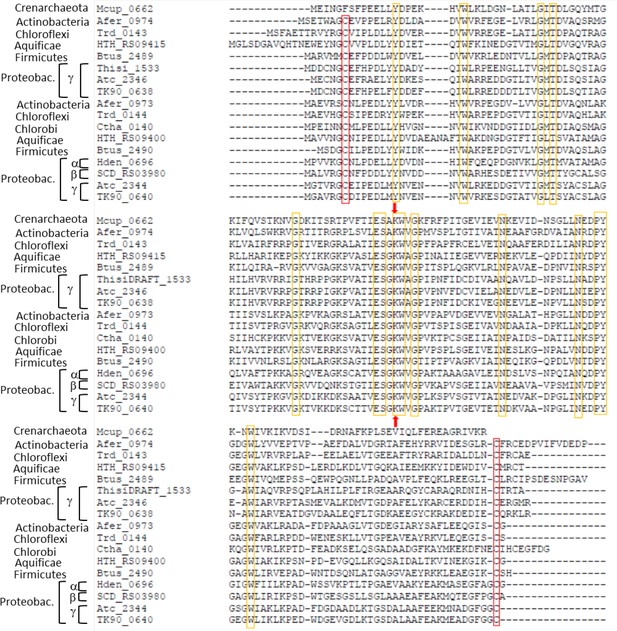
Sequence alignment of Hdr-associated lipoate-binding proteins from seven archaeal and bacterial phyla.
The strictly conserved lipoate-binding lysine residue shared with the H proteins of the glycine-cleavage system is indicated by red arrows. Strictly conserved residues are marked with yellow boxes. Strictly conserved cysteine residues are highlighted by red boxes. Species: Metallosphaera cuprina Ar4T (Mcup_0662 (AEB947679)) Acidimicrobium ferrooxidans DSM 10331T (Afer_0973 (Acu53912), Afer_0974 (ACU53913)), Thermomicrobium roseum DSM 5159T (Trd_0143 (ACM05628), Trd_0144 (ACM04497)), Hydrogenobacter thermophiles (HTH_RS09400 (HTH_1874, BAI70318), HTH_RS09415 (HTH_1877, BAI70321), Kyrpidia tusciae DSM 2912T(Btus_2489 (ADG07149), Btus_2490 (ADG07150)), Thiorohdospira sibirica ATCC 700588T (ThisiDRAFT_1533 (EGZ46119)), Acidithiobacillus caldus SM-1 (Atc_2344 (AEK58992), Atc_2346 (AEK58994)), Thioalkalivibrio sp. K90mix (TK90_0638 (ADC71153), TK_0640 (ADC71155)), Chloroherpeton thalassium ATCC 35110T (Ctha_0140 (ACF12611)), Hyphomicrobium denitrificans ATCC 51888T (Hden_0696 (ADJ22516)), Sulfuricella denitrificans DSM 22764T (SCD_RS03985 (SCD_01710, BAN34642)). Proteobac., Proteobacteria.
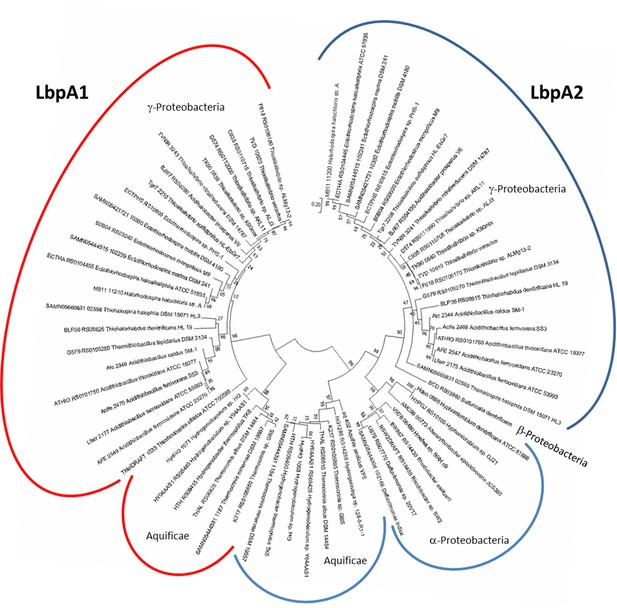
Molecular phylogenetic analysis of LbpA proteins by maximum likelihood method.
First, the best amino acid substitution model was calculated in MEGA7 (Kumar et al., 2016). The Le_Gascuel_2008 model (Le and Gascuel, 2008) had the lowest BIC (Bayesian information Criterion) score and was considered to describe the substitution pattern the best. The evolutionary history was then inferred by using the Maximum Likelihood method based on this model. The tree with the highest log likelihood (−5373.74) is shown. Bootstrap values given at branching points are based on 1000 replicates. Initial tree(s) for the heuristic search were obtained automatically by applying Neighbor-Join and BioNJ algorithms to a matrix of pairwise distances estimated using a JTT model, and then selecting the topology with superior log likelihood value. A discrete Gamma distribution was used to model evolutionary rate differences among sites (5 categories (+G, parameter = 0.8540)). The tree is drawn to scale, with branch lengths measured in the number of substitutions per site. The analysis involved 68 amino acid sequences. All positions containing gaps and missing data were eliminated. There were a total of 123 positions in the final alingment. Evolutionary analyses were conducted in MEGA7 (Kumar et al., 2016).
-
Figure 3—source data 1
List of source organisms, locus tags and accessions for the LbpA and GvcH proteins considered in phylogenetic trees shown in Figure 3 and Figure 3—figure supplement 1.
- https://doi.org/10.7554/eLife.37439.008
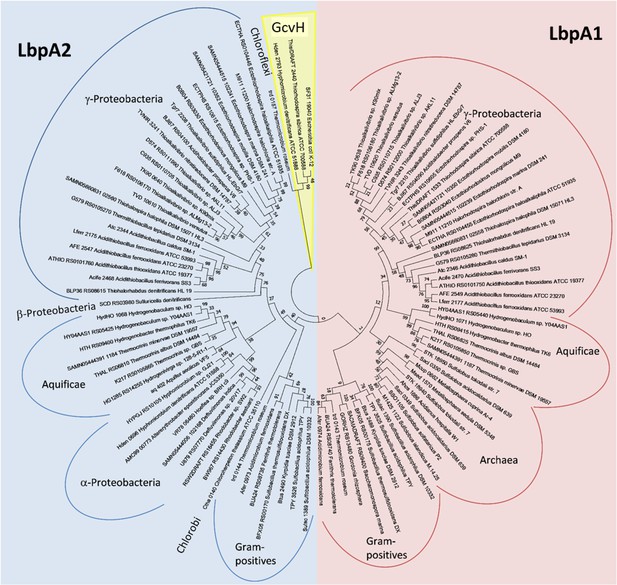
Molecular phylogenetic analysis of LbpA and GvcH proteins by maximum likelihood method.
Three bona fide GvcH proteins were included in the analysis (GcvH from Escherichia coli K12 substr. W3110 (BAE76969), ThisiDRAFT_2440 from Thiorhodospira sibirica, and Hden_2793 from Hyphomicrobium denitrificans). First, the best amino acid substitution model was calculated in MEGA7(Kumar et al., 2016). The Le_Gascuel_2008 model (Le and Gascuel, 2008) had the lowest BIC (Bayesian information Criterion) score and was considered to describe the substitution pattern the best. The evolutionary history was then inferred by using the Maximum Likelihood method based on this model. The tree with the highest log likelihood (−5373.74) is shown. Bootstrap values given at branching points are based on 1000 replicates. Initial tree(s) for the heuristic search were obtained automatically by applying Neighbor-Join and BioNJ algorithms to a matrix of pairwise distances estimated using a JTT model, and then selecting the topology with superior log likelihood value. A discrete Gamma distribution was used to model evolutionary rate differences among sites (5 categories (+G, parameter = 0.8540)). The tree is drawn to scale, with branch lengths measured in the number of substitutions per site. The analysis involved 98 amino acid sequences. All positions containing gaps and missing data were eliminated. There were a total of 123 positions in the final alingment. Evolutionary analyses were conducted in MEGA7 (Kumar et al., 2016).
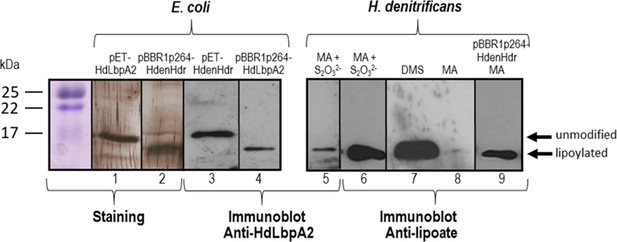
SDS-PAGE and Western blot of HdLbpA2.
Lanes 1, 2, 3 and 4 were loaded with 20 ng of pure recombinant HdLbpA2 stemming from pET- or pBBR1p264-based expression as indicated. Lanes 5 to 8 were loaded with extracts (15–20 μg protein) H. denitrificans wildtype grown on methylamine plus thiosulfate (MA + S2O32-), dimethylsulfide (DMS) and methylamine (MA). Lane 9 was loaded with extract (17.5 μg protein) of H. denitrificans expressing plasmid-encoded genes dsrEhdrC1B1AhyphdrC2B2hyplbpA from a constitutive promoter. Lanes 1 and 2 show silver stained gels. Western blots were developed with antiserum against HdLbpA2 or with anti-lipoic acid antibody as indicated.
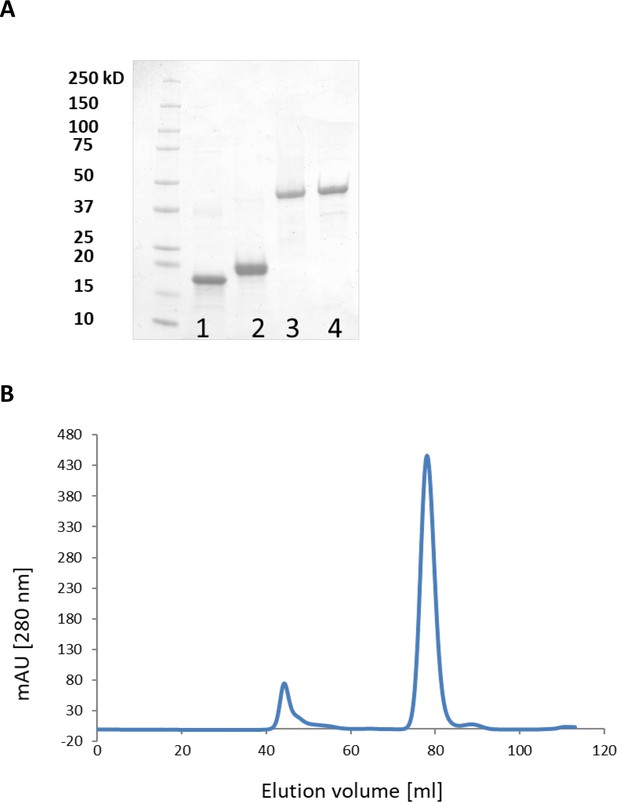
Purification of LbpA and LplA-like proteins and analysis of T. sibirica LbpA1 by gel permeation chromatography on Superdex 75.
(A) Purification of LbpA and LplA-like proteins. Lane 1, LbpA1 from T. sibirica; lane 2, LbpA2 from H. denitrificans; lane 3, LplA from Thioalkalivibrio sp. K90mix; lane 4, LplA from H. denitrificans. (B) Analysis of T. sibirica LbpA1 by gel permeation chromatography on Superdex 75. Buffer: 20 mM sodium phosphate, pH 7.4 containing 150 mM NaCl. Protein elution peaked at 79 ml corresponding to a mass of 16–18 kDa. The protein thus behaved as a monomer.
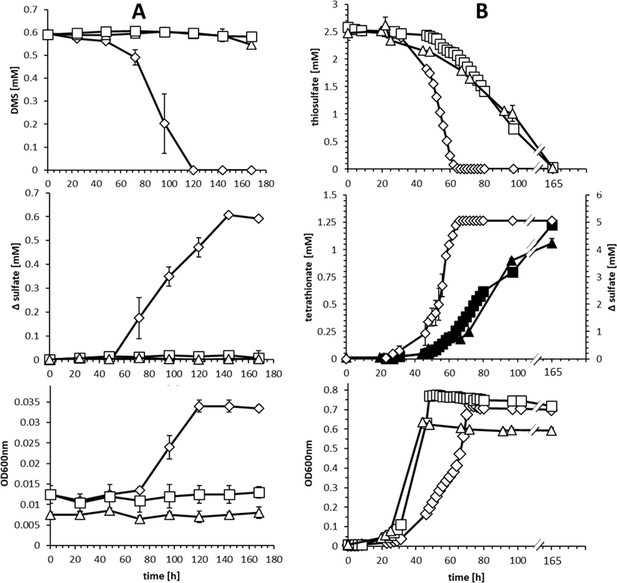
Comparison of substrate consumption, product formation and growth of wildtype and knockout strains of H. denitrificans during growth on different sulfur compounds.
(A) Consumption of DMS (upper panel) and growth on DMS (lower panel) by H.denitrificans wt (◊) and the ∆hdr (□) and ∆lbpA (∆) strains. DMS concentrations above 1.5 mM are toxic in batch culture and appreciable cell yields necessitate repeated feeding of cultures (Koch and Dahl, 2018). Stoichiometric production of sulfate from DMS for the wt is shown in the middle panel. (B) Consumption of thiosulfate (upper panel) on methanol-containing medium by H. denitrificans wt (◊), the ∆hdr (□) and the ∆lbpA (∆) strain. Sulfate formation by the wt (open diamonds, ◊) and tetrathionate formation by the ∆hdr (filled boxes, ■) and ∆lbpA (filled triangles ▲) strains is given in the middle panel. The lower panel shows growth on methanol plus thiosulfate. Representative experiments averaged from two independent biological replicates are shown. Error bars indicate SD.
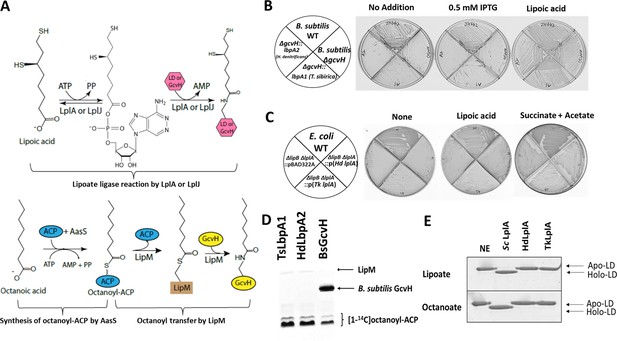
In vivo and in vitro activities of lbpA and LbpA.
(A) Biochemical reactions for lipoate/octanoate assembly. Upper panel, lipoate ligase reaction catalyzed by LplA or LplJ. When lipoic acid is provided in the environment, LplA/LplJ catalyzes the ligase reaction in two steps. First, lipoic acid is activated to lipoyl-AMP with concomitant release of pyrophosphate. In the presence of a LD or GcvH acceptor protein, the lipoyl moiety is transferred to the LD or GcvH. LD, lipoyl domain. Lower panel, synthesis of octanoyl-ACP and amidotransfer of octanoyl moiety from ACP to GcvH. The synthesis of octanoyl-ACP requires AasS (the acyl-ACP synthetase from Vibrio harveyi) holo-ACP, ATP and free octanoic acid. The octanoyl transfer step requires LipM, which is the octanoyl transferase in B. subtilis. (B) Complementation of B. subtilis ∆gcvH NM20 strain with lbpA genes from sulfur oxidizers and LipM modification assay. The lbpA genes were integrated into the chromosomal amyE site of the B. subtilis ΔgcvH strain under control of Pspac promoter and were inducible by IPTG. Colony formation was scored on minimal agar plates with glucose as the sole carbon source and supplements indicated after 36 hr at 37°C. The wild type (JH642) and B. subtilis ∆gcvH strain (NM20) served as positive and negative controls, respectively. No discrete colonies were formed by the strains that expressed LbpA1 and LbpA2 (the imperfections are scratches from the streaking), whereas strains all grew well with lipoic acid supplementation. (C) Complementation of lipoate auxotrophic E. coli ∆lipB ∆lplA strain with LplA proteins from sulfur oxidizers. The control strains were the wild-type (WT) strain and E. coli ∆lipB ∆lplA strain containing the empty vector (pBAD22A). Strains were grown on M9 minimum glycerol media plates with the indicated supplements at 37°C for 36 hr. Complementation proceeded both in the presence and absence of arabinose induction. Plates above contained 0.2% arabinose. (D) Assay of [1-14C]octanoyl transfer from [1-14C]octanoyl-ACP to lipoate-binding proteins (TsLbpA1 from T. sibirica and HdLbpA2 from H. denitrificans) and to B. subtilis GcvH (BsGcvH) as indicated in the figure. The [1-14C]octanoyl-ACP was synthesized in situ by Vibrio harveyi AasS acyl-ACP synthetase (ATP was added for the AasS reaction). Reaction was analyzed on SDS-PAGE by autoradiography. (E) Mobility shift assay for analysis of in vitro lipoylation and octanoylation catalyzed by LplA using the E2AceF E. coli lipoyl domain (LD) as acceptor. Loss of the positive charge of the modified lysineε−amino group of the LD results in faster migration of the modified form on Urea-PAGE gels. NE, no enzyme; Sc LplA, LplA from S. coelicolor; HdLplA, LplA from H. denitrificans; TkLplA, LplA from Thioalkalivibrio sp. K90mix.
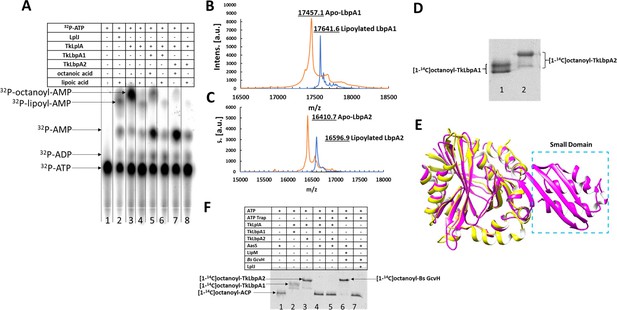
Enzymatic role of LplA from sulfur oxidizing bacteria in lipoylation/octanoylation of Lbp proteins.
(A) TLC analysis of products formed from [α-32P]ATP. Both synthesis of lipoyl/octanoyl-5’-AMP and transfer of the lipoyl/octanoyl moiety to the acceptor proteins LbpA1 and LbpA2 are shown respectively. Addition of an acceptor protein results in consumption of the acyl adenylate intermediates and production of AMP. TkLplA, TkLbpA1 and TkLbpA2 denote LplA, LbpA1 and LbpA2 proteins from Thioalkalivibrio sp. K90mix, respectively. (B and C) MALDI mass spectrometric analysis of lipoylation of TkLbpA1 and TkLbpA2 respectively catalyzed by LplA from Thioalkalivibrio sp. K90mix. The mass of the lipoylated LbpA1 (17641.6 Da) and lipoylated LbpA2 form (16596.9 Da) agree well the calculated values. The change in mass upon modification (calculated for lipoyl modification, 188 Da; observed, 184.5 Da for LbpA1 and 186.2 Da for LbpA2 respectively) is within the accuracy of the instrument utilized. Intens., intensity; a.u., arbitrary units. (D) Activity of TkLplA (KEGG: TK90_0642) on the two lipoate-binding proteins. [1-14C]octanoic acid was used as the substrate. Reactions were incubated at 37°C for 1 hr and loaded on a 15% SDS-PAGE gel. The [1-14C]octanoyl-Lbp proteins were detected by autoradiography. Lane 1, LbpA1 from Thioalkalivibrio sp. K90mix (TK90_0638); lane 2, LbpA2 from Thioalkalivibrio sp. K90mix (TK90_0640). (E) Superimposition model of from Thioalkalivibrio sp. K90mix LplA (yellow) obtained by threading on the structure of E. coli LplA (magenta, PDB 3A7R). The small domain of E. coli LplA was denoted by blue dotted box. (F) Detection of [1-14C]octanoyl transfer from [1-14C]octanoyl-ACP to lipoate-binding proteins (Lbps). The [1-14C]octanoyl-ACP was synthesized in situ from [1-14C]octanoate, V. harveyi AasS acyl ACP synthetase, ATP and E. coli holo-ACP, whereas formation, if any, of [1-14C]octanoyl-Lbp should require apo-Lbp, octanoyl transferase and [1-14C]octanoyl-ACP. Note that the residual ATP remaining from the AasS reaction was depleted by use of an ATP trap (2 units of hexokinase plus 10 mM D-glucose to convert ATP to glucose-6-phosphate and ADP). Each reaction contained ATP, [1-14C]octanoate, and E. coli holo-ACP. Lane 1, [1-14C]octanoyl-ACP; Lane 2, positive control LbpA1 (TK90_0638) in the presence of ATP and LplA (TK90_0642); Lane 3, positive control LbpA2 (TK90_0640) in the presence of ATP and LplA (TK90_0642); Lane 4, LbpA1with AasS coupled reaction in the presence of LplA and ATP trap; Lane 5, LbpA2 with AasS coupled reaction in the presence of LplA and ATP trap; Lane 6, B. subtilis GcvH protein with AasS coupled reaction in the presence of a real octanoyltransferase LipM from B. subtilis and ATP trap.
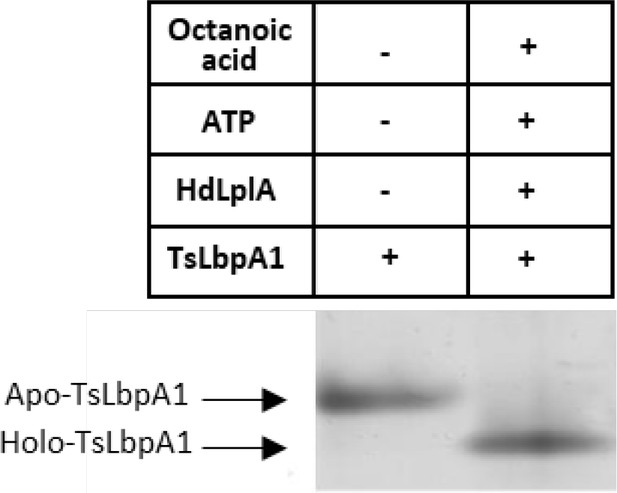
Mobility shift assay for analysis of in vitro octanoylation catalyzed by HdLplA from Hyphomicrobium denitrificans (Hden_0686) using LbpA1 from Thiorhodospira sibirica (TsLbpA1, ThisiDRAFT_1533) as acceptor.
Loss of the positive charge of the modified lysine ε−amino group of TsLbpA1 results in faster migration of the modified form on Urea-PAGE gels.
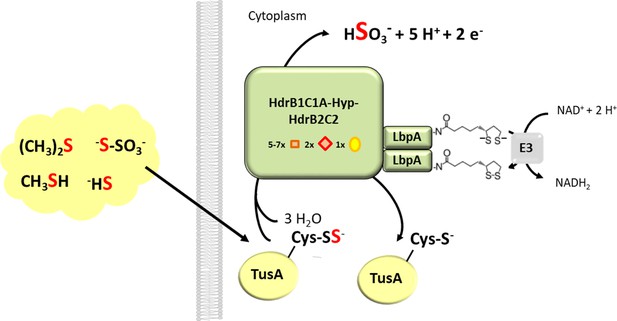
Proposed function of LbpA proteins in the Hdr-like pathway of sulfur oxidation.
The TusA protein is proposed as a central sulfur carrier in the cytoplasm and collects sulfur stemming from the oxidation of organic and reduced inorganic sulfur compounds like DMS, methanethiol, sulfide or thiosulfate (Dahl, 2015; Koch and Dahl, 2018). A complex of Hdr-like proteins containing non-cubane [4Fe-4S] clusters (red diamonds) that serve as the (hetero)disulfide-reducing active site as well as regular [4Fe-4S] clusters (orange boxes) and FAD oxidizes the protein-bound sulfur to sulfite. In the course of the reaction two of the four electrons released per reaction cycle may serve as reductants for dihydrolipoyl formation on LpbA. Dihydrolipoyl dehydrogenase (indicated here as component E3 of the pyruvate dehydrogenase complex) then catalyzes reoxidation of the cofactor to lipoamide and concomitant reduction of NAD+.
Tables
LbpA proteins studied in this work and their identity/similarity with E. coli GcvH.
https://doi.org/10.7554/eLife.37439.009Protein and locus tag | Source organism | E. coli GcvH (identity) | E. coli GcvH (similarity) |
---|---|---|---|
LbpA1 ThisiDRAFT_1533 | Thiorhodospira sibirica ATCC 700588T | 25.6% | 41.7% |
LbpA1 TK90_0638 | Thioalkalivibrio sp. K90mix | 29.5% | 45.6% |
LbpA2 TK90_0640 | Thioalkalivibrio sp. K90mix | 34.5% | 52.4% |
LbpA2 Hden_0696 | Hyphomicrobium denitrificans ATCC 51888T | 28.8% | 45.2% |
Protein identity/similarity between LplA-like proteins studied in this work and their identity/similarity with E. coli LplA, LipB and B. subtilis LipM respectively.
https://doi.org/10.7554/eLife.37439.014Locus tag | Source organism | E. coli LplA (Identity/Similarity) | E. coli LipB (Identity/Similarity) | B. subtilis LipM (Identity/Similarity) |
---|---|---|---|---|
Hden_0686 | Hyphomicrobium denitrificans ATCC 51888T | 18.8%/33.1% | 10.8%/18.5% | 16.9%/31.8% |
TK90_0642 | Thioalkalivibrio sp. K90mix | 23.9%/36% | 10.4%/19.2% | 18.9%/33.6% |
Additional files
-
Supplementary file 1
Table S1 – strain list.
Table listing the strains and plasmids used in this study.
- https://doi.org/10.7554/eLife.37439.018
-
Supplementary file 2
Table S2 primer and plasmid list.
Table listing the primer and plasmid names and properties used in this study.
- https://doi.org/10.7554/eLife.37439.019
-
Transparent reporting form
- https://doi.org/10.7554/eLife.37439.020