Neurocalcin regulates nighttime sleep and arousal in Drosophila
Figures
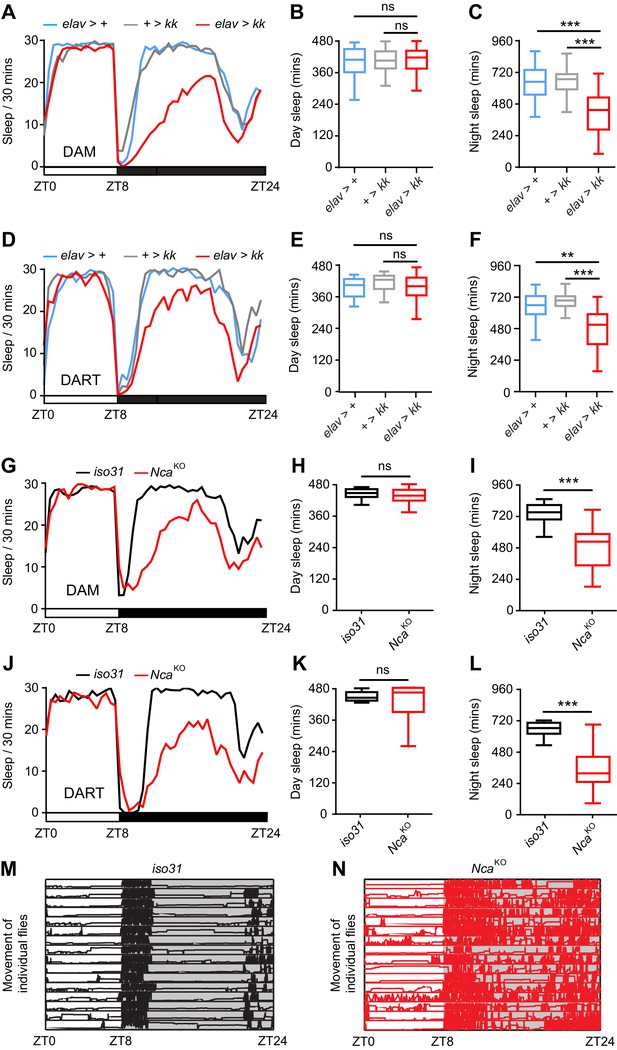
Neurocalcin promotes night sleep.
(A) Mean sleep levels measured using the DAM system under 8L: 16D conditions for adult male pan-neuronal Nca knockdown flies (elav > kk) and associated controls (elav-Gal4 driver or kk RNAi transgene heterozygotes). (B–C) Median day (B) and night (C) sleep levels in the above genotypes. n = 54–55. Data are presented as Tukey box plots. The 25th, Median, and 75th percentiles are shown. Whiskers represent 1.5 x the interquartile range. Identical representations are used in all subsequent box plots. (D) Mean sleep levels measured using the DART system in 8L: 16D conditions for male adult pan-neuronal Nca knockdown flies (elav > kk) and associated controls. (E–F) Median day (E) and night (F) sleep levels in the above genotypes. n = 20 per genotype. (G) Mean sleep levels in 8L: 16D conditions for NcaKO adult males and iso31 controls measured using the DAM. (H–I) Median day (H) and night (I) sleep levels in the above genotypes. n = 32 per genotype. (J) Mean sleep levels in 8L: 16D conditions for NcaKO adult males and iso31 controls measured by DART. (K–L) Median day (K) and night (L) sleep levels in the above genotypes. n = 16 per genotype. (M–N) The longitudinal movement for individual iso31 (M) and NcaKO (N) flies are shown as rows of traces plotting vertical position (Y-axis) over 24 hr (X-axis) under 8L: 16D condition. ns (not significant) - p>0.05, **p<0.01, ***p<0.001, Kruskal-Wallis test with Dunn’s post-hoc test (B–C, E–F) or Mann-Whitney U-test (H–I, K–L).
-
Figure 1—source data 1
Sleep, velocity, rhythmicity data and gene expression data from Nca knockdown and knockout flies relating to Figure 1 and associated figure supplements.
Blank cells represent data from dead flies removed prior to analysis.
- https://doi.org/10.7554/eLife.38114.011

Human Hippocalcin and Drosophila Neurocalcin are highly homologous neuronal calcium sensors.
Amino-acid alignment of human Hippocalcin (Hs HPCA) and Drosophila Neurocalcin (Dm NCA) is shown. Blue boxes: location of the calcium-binding EF-hand domains of Hippocalcin and Neurocalcin. Black boxes represent full amino-acid conservation, grey boxes represent functional conservation.
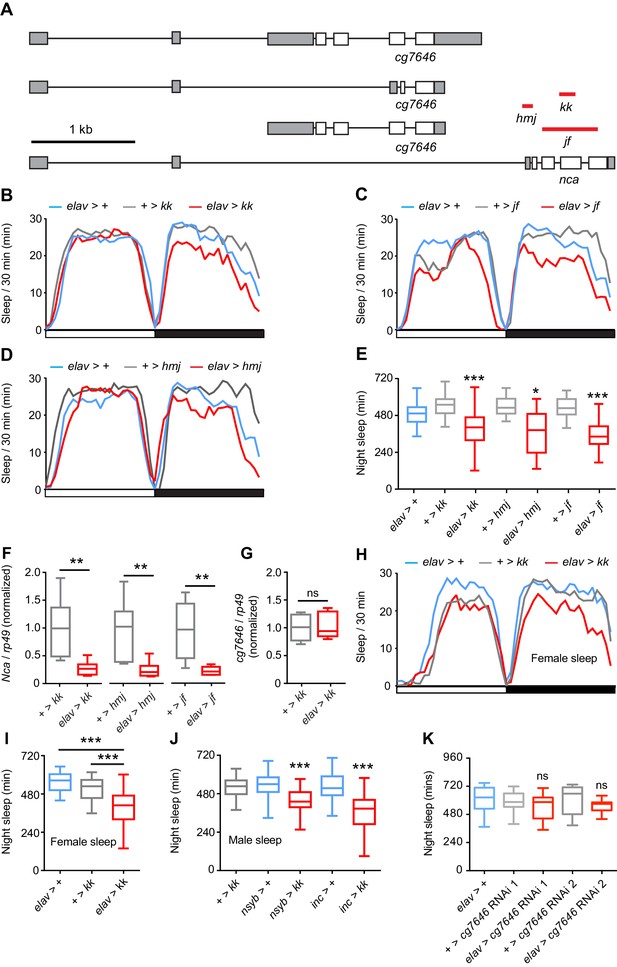
Pan-neuronal knockdown of Nca using independent RNAi lines causes night sleep loss.
(A) Schematic showing transcripts derived from the Nca locus alongside transcripts derived from the cg7646 locus, which shares common 5’ untranslated regions with Nca. Regions of Nca mRNA targeted by the kk108825, hmj21533 and jf03398 RNAi lines (termed kk, hmj and jf respectively) are shown as red bars. (B–D) Mean sleep levels measured using the DAM system under 12L: 12D conditions for male adult pan-neuronal Nca knockdown flies (B: elav > kk, C: elav > jf; D: elav > hmj) and associated controls (elav-Gal4 driver or RNAi transgene heterozygotes). n = 17–48. (E) Median sleep levels in the above genotypes. Night sleep is significantly reduced in all knockdown backgrounds compared to both transgene and driver alone controls. (F) qPCR verification of Nca knockdown by the kk, hmj and jf RNAi constructs. Transgene insertions lacking the elav-Gal4 driver were used as controls. (G) Knockdown of Nca had no effect on expression of cg7646. Expression levels of Nca or cg7646 were normalised to the ribosomal protein 49 (rp49) control transcript and are displayed as the ratio to the mean level of the respective RNAi alone controls (+ > kk, + > hmj or + > jf). n = 6–9 for all qPCRs (2–3 independent biological repetitions of RNA extraction with triplicated qPCR reactions for each genotype). (H–I) Pan-neuronal Nca knockdown in adult Drosophila females reduces night sleep. Mean sleep patterns of NcaKD females and associated controls in 12L: 12D conditions are shown in (H). Median night sleep levels are shown in (I). Day sleep levels are unaffected relative to heterozygous kk RNAi insertion controls (H). n = 31–32. (J) Pan-neuronal or broad Nca knockdown in adult males using either nsyb- or insomniac (inc)-Gal4 also reduced total night sleep levels in 12L: 12D conditions compared to both transgene and driver alone controls. n = 38–53. (K) Pan-neuronal expression of RNAi targeting cg7646 mRNA did not alter night sleep in Drosophila males compared to both transgene and driver alone controls. Two different chromosomal insertions of the same RNAi hairpin were used (RNAi one and RNAi 2). n = 12–15. ns - p>0.05, *p<0.05, **p<0.01, ***p<0.001, Kruskal-Wallis test with Dunn’s post-hoc test (E, I, J, K) or Mann-Whitney U-test (F, G).

Reduced consolidated sleep in NcaKD flies.
(A–C) Individual sleep bout durations were measured using a custom-made R program and visualised by plotting sleep bout onset against offset for sleep bouts in control and NcaKD adult males. In control flies (elav > + and + > kk), longer sleep bouts initiated early during the night are highlighted in red (A, B), which are largely absent in NcaKD adult males (C). n = 48 for each genotype. (D) Distribution of sleep bout lengths in NcaKD and control adult males. Note the significant shift towards shorter sleep bout lengths in NcaKD flies (NcaKD vs. driver alone control, χ2, df: 142.0, 4, p<0.0001; vs. RNAi alone control, χ2, df: 2112.0, 4, p<0.0001).

Pan-neuronal expression of independent Nca RNAi lines results in night sleep loss in 8L: 16D conditions.
(A–B) Mean sleep profiles under 8L: 16D conditions for elav-Gal4 driven hmj (A) or jf (B) Nca RNAi. (C) Median night sleep amounts for genotypes shown in (A–B). elav > +: n = 32; + > hmj: n = 26; elav > hmj: n = 17; + > jf: n = 32; elav > jf: n = 32. (D–F) Nca knockdown in muscle cells does not affect sleep in Drosophila. (D) Mean sleep patterns of adult male flies with muscle-specific Nca knockdown via mef2-Gal4 (mef2 > kk) and associated controls under 8L: 16D. (E–F) Median day (E) and night (F) sleep levels are unaffected relative to controls. n = 16 per genotype. ns – p>0.05, Kruskal-Wallis test with Dunn’s post-hoc test. **p<0.01, ***p<0.001, ns – p>0.05, Kruskal-Wallis test with Dunn’s post-hoc test.

Nca knockdown does not alter circadian rhythmicity.
(A) Actograms showing representative individual patterns of locomotor activity in one day of 12L: 12D conditions followed by 11 days of free-running activity in constant dark (DD) conditions. (B) Mean locomotor rhythm strength in NcaKD adult males and controls. Robust circadian patterns of locomotor activity were still observed following in adult males expressing Nca RNAi (kk) under elav-Gal4 relative to controls. Error bars represent standard error of the mean. n = 14–15.

Generation of Nca null alleles using ends-out homologous recombination.
(A) Schematic illustration of the procedure used to generate Nca knockout alleles. Homologous arms upstream (Arm 1) and downstream (Arm 2) of the Nca locus are indicated. Upstream 5’ promoter regions shared by the cg7646 and Nca loci (see Figure 1—figure supplement 2A) are external to the homologous arm sequences and are not shown. Following homologous recombination, the endogenous Nca locus is replaced by a cassette containing the mini-white selection marker (red bar), and attP (blue bar) and loxP sites (yellow bars). The mini-white cassette was subsequently removed via Cre-loxP recombination. (B–C) PCR validation of homologous recombination events. Correct recombination was verified using primers designed to the attP site and upstream of the cg7646 coding regions (B), which will only generate a ~ 3 kb product following homologous recombination between the targeting vector and the Nca locus (C). Three independent targeting events (ko1-3) were validated by genomic PCR. WT: wild-type genome lacking an attP site 3’of cg7646. (D–E) No Nca mRNA was detected in NcaKO1 using either standard RT-PCR (D) or quantitative RT-PCR (E; n = 3 qPCR reactions for iso31 control and NcaKO1 flies).
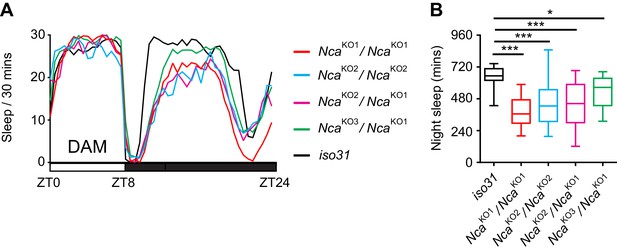
Independent combinations of Nca knockout alleles exhibit night sleep loss.
(A–B) Trans-heterozygotic combinations of the NcaKO1-3 alleles, as well as homozygotes for the NcaKO2 allele, all result in significant night sleep loss compared to iso31 controls. Night sleep levels in NcaKO1 homozygotes are also shown. (A) Mean sleep levels in the above genotypes in 8L: 16D conditions. (B) Median night sleep. n = 15–26. *p<0.05, **p<0.01, ***p<0.001 compared to iso31 controls, Kruskal-Wallis test with Dunn’s post-hoc test.
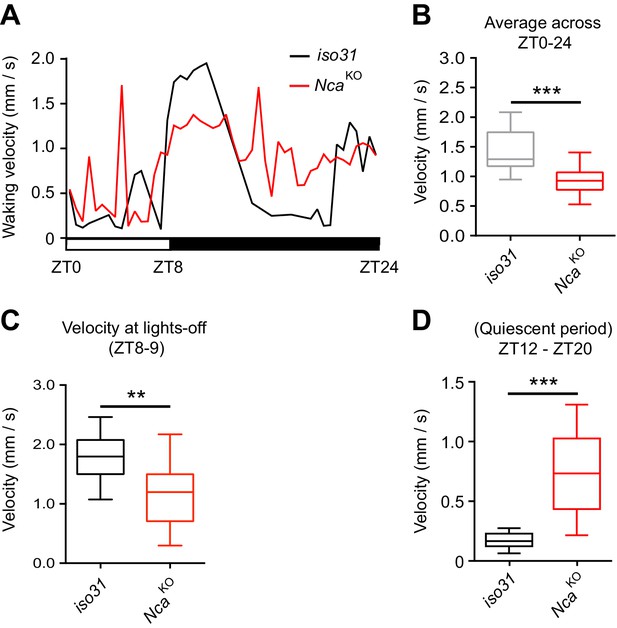
Locomotor velocities in Nca knockout flies.
(A) Mean locomotor velocities across a 24 hr period in 8L: 16D conditions in NcaKO1 adult males and iso31 controls, measured via the DART system, reveals increased locomotor velocity during the night and reduced velocity during the evening activity peak NcaKO1 flies. (B) Median locomotor velocities across 24 hr in 8L: 16D conditions in NcaKO1 adult males and iso31 controls. (C) Median locomotor velocity is significantly reduced in NcaKO1 flies during the evening activity peak (ZT8-ZT9). (D) NcaKO1 flies exhibit a significant increase in locomotor velocity between ZT12-ZT20 compared to controls – a normally quiescent period. n = 16 per genotype. **p<0.01, ***p<0.001, Mann-Whitney U-test.
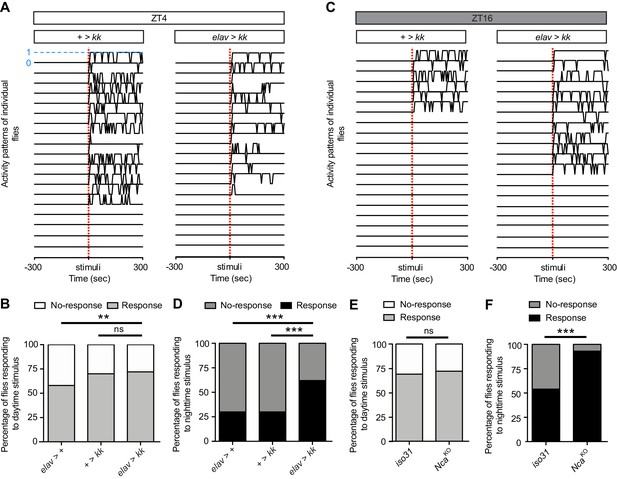
NCA reduces responsiveness to stimuli at night under 8L: 16D conditions.
(A, C) Locomotor activity in twenty representative control (+ > kk) and NcaKD (elav > kk) adult male flies at either ZT4 (A) or ZT16 (C), as measured using the DART system. X-axis denotes 300 s before and after a vibration stimulus (red dotted line). Y-axis represents movement of individual flies in a binary manner (1 = movement, marked by blue dotted line for one fly; 0 = immobility). Only flies that were immobile for five mins preceding the stimulus were selected for analysis. (B, D) Percentage of NcaKD and control flies responding or not responding to vibration stimulus at either ZT4 (B) or ZT16 (D). ZT4: elav > +: n = 24, + > kk: n = 33, elav > kk: n = 33. ZT16: elav > +: n = 23, + > kk: n = 30, elav > kk: n = 29. (E, F) Percentage of NcaKO and iso31 control flies responding or not responding to vibration stimulus at either ZT4 (E) or ZT16 (F). ZT4: iso31: n = 48, NcaKO: n = 53. ZT16: iso31: n = 48, NcaKO: n = 44. ns – p>0.05, **p<0.01, ***p<0.001, Binomial test with Bonferonni correction for multiple comparisons.
-
Figure 2—source data 1
Proportion of Nca knockdown and knockout flies responding to mechanical stimuli.
- https://doi.org/10.7554/eLife.38114.013
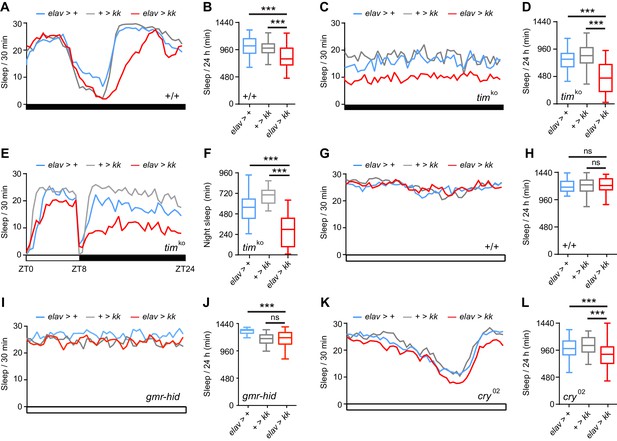
Circadian clock and light-sensing pathways define when NCA promotes sleep.
(A–B) Mean sleep levels in NcaKD and control adult males across 24 hr in constant-dark (DD) conditions (A), and total median sleep levels in the above genotypes (B). n = 44–47. Note the reduced sleep in the subjective night in NcaKD relative to control adult males, but not the day. (C–D) Mean sleep levels in NcaKD and control adult males across 24 hr in DD conditions in a timeless knockout (timKO) background (C), and total median sleep levels (D). n = 32–39. (E–F) Mean sleep levels in NcaKD and control adult males across 24 hr in 8L: 16D conditions in a timKO background (E), median night sleep levels (F). n = 22–26. (G–H) Mean sleep levels in NcaKD and control adult males across 24 hr in constant-light (LL) conditions (G), and total median sleep levels (H). n = 44–47. (I–J) Mean sleep levels in NcaKD and control adult males across 24 hr in LL conditions in a gmr-hid background (I), and total median sleep levels (J). elav > kk, gmr-hid/+: n = 51; + > kk, gmr-hid/+: n = 48; elav > +, gmr-hid/+: n = 24. (K–L) Mean sleep levels in NcaKD and control adult males across 24 hr in LL conditions in a cryptochrome null (cry02) background (K), and total median sleep levels in the above genotypes (L). n = 61–72. Note the small but consistent reduction in sleep in NcaKD, cry02 males (K), leading to a significant decrease in total median sleep levels relative to controls (L). ns - p>0.05, ***p<0.001, as compared to driver and RNAi alone controls via Kruskal-Wallis test with Dunn’s post-hoc test.
-
Figure 3—source data 1
Sleep levels in Nca knockdown flies under varying environmental and genetic conditions.
Blank cells represent data from dead flies removed prior to analysis.
- https://doi.org/10.7554/eLife.38114.015
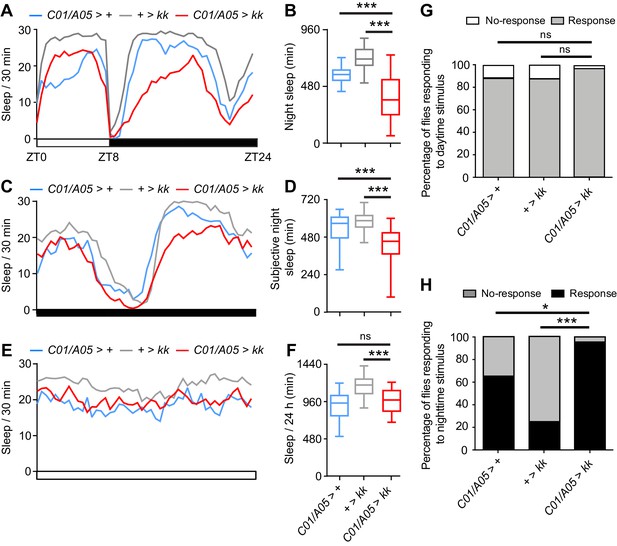
NCA acts in a two distinct neural subpopulations to regulate night sleep.
(A–F) Sleep patterns in adult male flies with Nca knockdown (using the kk Nca RNAi) in two neural domains defined by the A05- and C01-Gal4 drivers in varying light/dark regimes, compared to controls. (A–B) A: mean sleep patterns in 8L: 16D conditions. B: median night sleep in Nca knockdown flies compared to heterozygote drivers and transgene alone controls. + > kk: n = 80; C01/A05 > +: n = 42; C01/A05 > kk: n = 71. (C–D) Mean sleep patterns (C) and median subjective night sleep (D) in constant dark (DD) conditions. + > kk: n = 64; C01/A05 > +: n = 47; C01/A05 > kk: n = 51. (E–F) Mean sleep patterns (E) and median total sleep (F) in constant light (LL) conditions. + > kk: n = 76; C01/A05 > +: n = 26; C01/A05 > kk: n = 28. (G–H) Percentage of C01/A05 > kk and control flies responding or not responding to vibration stimuli at either ZT4 (G; C01/A05 > kk, n = 38, + > kk, n = 61 and C01/A05 > +, n = 26) or ZT16 (H; C01/A05 > kk, n = 24, + > kk, n = 54 and C01/A05 > +, n = 28) under 8L: 16D conditions. ns – p>0.05, *p<0.05, **p<0.01, ***p<0.001, compared to driver and RNAi alone controls, Kruskal-Wallis test with Dunn’s post-hoc test (B, D, F) or Binomial test with Bonferonni correction for multiple comparisons (G–H).
-
Figure 4—source data 1
Sleep levels and proportion of flies responding to mechanical stimuli following Nca knockdown in C01- and A05-neurons or other specific neuronal subtypes, relating to Figure 4 and associated figure supplements.
Blank cells represent data from dead flies removed prior to analysis.
- https://doi.org/10.7554/eLife.38114.019

Transgenic RNAi-based mini-screen to identify key NCA-expressing neurons.
(A) Nca knockdown with broadly expressed drivers resulted in reduced night sleep in adult males under 8L: 16D conditions. In contrast, Nca knockdown in previously defined sleep-regulatory centers, clock neurons, the visual system or subsets of Dop1R1-expressing neurons did not impact night sleep. FSB: fan-shaped body. MB: mushroom body. Grey and blue box plots: control lines. Magenta box plots: experimental lines showing reduced night sleep relative to controls. Green box plots: experimental lines failing to show reduced night sleep relative to one or both controls. Grey box plot: kk Nca RNAi alone (+ > kk) controls. Blue box plots: Gal4 driver heterozygotes. (B) Nca knockdown using combinations of driver lines labelling C01- and A05-neurons in addition to neurons in the dopaminergic pathway (dopamine-release and Dop1R1-expressing neurons), the anterior visual pathway (tubercular-bulbar (TuBu) neurons), and cryptochrome (cry)-expressing neurons. See Figure 4 Source Data for n-values and additional statistical comparisons. *p<0.05, **p<0.01, ***p<0.001, as compared to driver and RNAi alone controls via Kruskal-Wallis test with Dunn’s post-hoc test.
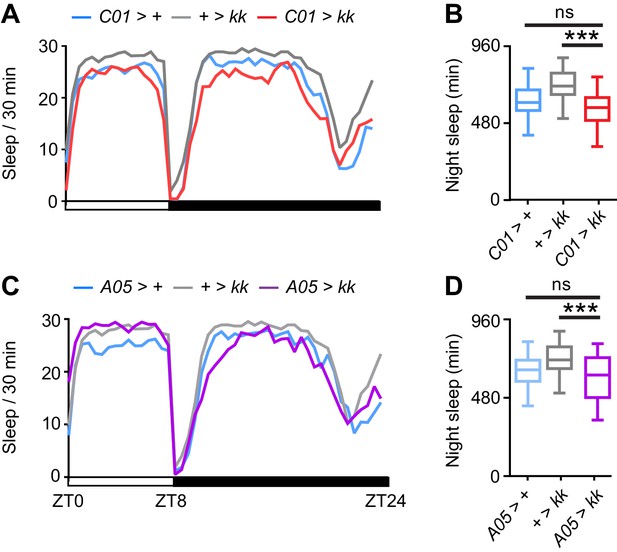
Nca knockdown in C01- or A05-neurons alone does not significantly alter sleep.
(A–B) Mean sleep patterns (A) and median night sleep (B) of adult males with expressing Nca RNAi (kk) in C01-neurons compared to heterozygote driver and transgene alone controls in 8L: 16D conditions. + > kk: n = 80, C01 > +: n = 64, C01 > kk: n = 80. (C–D) Mean sleep patterns (A) and median night sleep (B) of adult males with expressing Nca RNAi (kk) in A05-neurons compared to controls in 8L: 16D conditions. + > kk: n = 80, A05 > +: n = 31, A05 > kk: n = 31. Note that the same population of + > kk control males was used in Figure 4A–B, as the combined C01- and A05-Gal4 experiments were performed in parallel. ns – p>0.05, ***p<0.001 compared to driver and RNAi alone controls, Kruskal-Wallis test with Dunn’s post-hoc test.
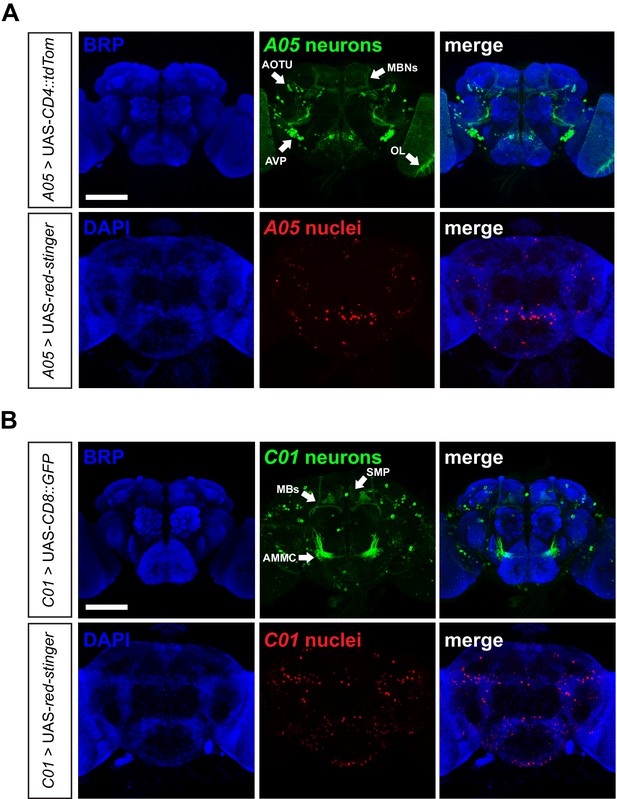
Distribution of A05- and C01-neurons in the adult Drosophila brain.
(A–B) Confocal z-stacks of adult male brains expressing genetically-encoded fluorophores labelling either neuronal processes (CD4::TdTom or CD8::GFP) or nuclei (Red-stinger) under the A05- (A) or C01-Gal4 (B) drivers. Neuropil regions are labelled with anti-Bruchpilot (BRP). Nuclei are co-labelled with DAPI. Scale bars, 100 µm. Arrows point to neuropil centers. AOTU: anterior optic tubercle. MBNs: mushroom body neurons. OL: optic lobe. AMMC: antennal mechanosensory and motor center. AVP: anterior ventrolateral protocerebrum. SMP: superior medial protocerebrum.
-
Figure 5—source data 1
Sleep levels following Nca knockdown in C01-, A05- or ok107-neurons (or combinations of), relating to Figure 5—figure supplements 1 and 2.
Blank cells represent data from dead flies removed prior to analysis.
- https://doi.org/10.7554/eLife.38114.023
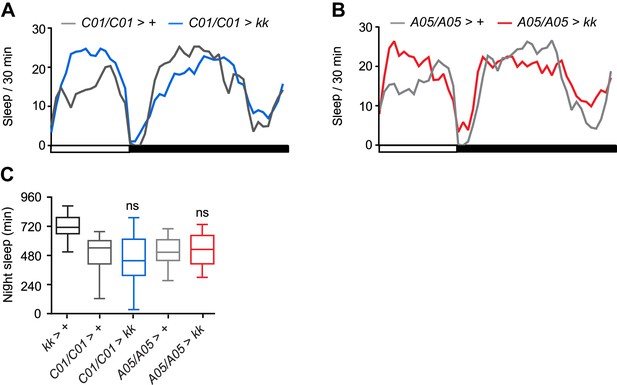
Nca knockdown using homozygous C01- or A05-Gal4 drivers does not affect night sleep.
(A) Mean sleep patterns of adult males homozygous for the C01-Gal4 driver with and without the kk Nca RNAi insertion. (B) Mean sleep patterns of adult males homozygous for the A05-Gal4 driver with and without the kk Nca RNAi insertion. (C) Median night sleep levels for heterozygous RNAi transgene and homozygous driver controls, and males expressing Nca RNAi with two Gal4 driver copies. No night sleep loss was observed using two copies of either driver relative to controls. + > kk: n = 80 (the same population was used in Figure 4A–B, as the experiments were performed in parallel), C01/C01 > +: n = 24, C01/C01 > kk: n = 39; A05/A05 > +: n = 22, A05/A05 > kk: n = 23. ns – p>0.05, Kruskal-Wallis test with Dunn’s post-hoc test.

The mushroom bodies are a sleep-relevant subdomain within C01-neurons.
(A) Standardised confocal stacks labelling R14A05 (A05 > GFP, green) and R72C01 (C01 > GFP, magenta) positive neurons. Images from Jenett et al. (2012), deposited at the Virtual Fly Brain, www.virtualflybrain.org), were downloaded and digitally superimposed (right panel) onto the universal fly brain (the image source data is distributed under a CC BY-NC-SA 4.0 license). BRP, Bruchpilot. (B–C) Mean sleep pattern (B) and median night sleep levels (C) in adult male flies expressing Nca RNAi (kk) in MB-KCs using ok107-gal4 in 8L: 16D. ok107 > +, n = 55, + > kk, n = 47, ok107 > kk, n = 65. (D–E) Nca knockdown in both A05 and MB-KC neurons results in reduced night sleep. Mean sleep patterns in 8L: 16D are shown in (D), median night sleep levels are shown in (E). A05/ok107 > +: n = 33, + > kk: n = 31, A05/ok107 > kk: n = 42. (F–G) Knocking down Nca in MB-KC and the C01-neurons does not result in significant night sleep loss in 8L: 16D. Mean sleep patterns are shown in (F), median night sleep levels are shown in (G). C01/ok107 > +: n = 11, + > kk: n = 15, C01/ok107 > kk: n = 21. ns – p>0.05, *p<0.05, **p<0.01, ***p<0.001, Kruskal-Wallis test with Dunn’s post-hoc test.
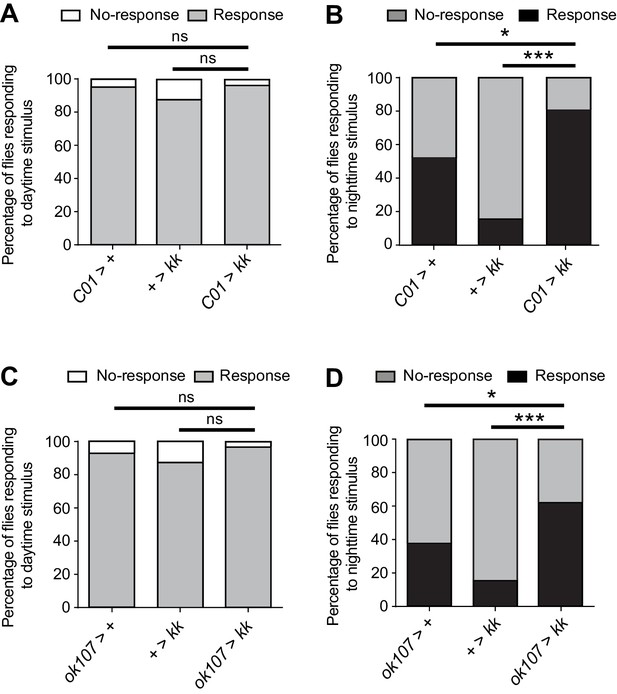
NCA acts in the mushroom bodies to regulate nocturnal arousal.
(A–B) Percentage of adult male flies expressing Nca RNAi (kk) in C01-neurons (C01 > kk) and control flies responding or not responding to vibration stimulus at either ZT4 (day; A) or ZT16 (night; B). ZT4: C01 > +, n = 22, + > kk, n = 61, C01 > kk, n = 27. ZT16: C01 > +, n = 19, + > kk, n = 54, C01 > kk, n = 21. (C–D) Percentage of adult male flies expressing Nca RNAi (kk) in MB-KCs (ok107 > kk) and control flies responding or not responding to vibration stimulus at either ZT4 (day; C) or ZT16 (night; D). ZT4: ok107 > +, n = 26, + > kk, n = 47, ok107 > kk, n = 28. ZT16: ok107 > +, n = 26, + > kk, n = 44, ok107 > kk, n = 27. ns – p>0.05, *p<0.05, ***p<0.001, Binomial test with Bonferonni correction for multiple comparisons.
-
Figure 6—source data 1
Proportion of flies responding to mechanical stimuli following Nca knockdown in C01- or ok107-neurons.
- https://doi.org/10.7554/eLife.38114.025
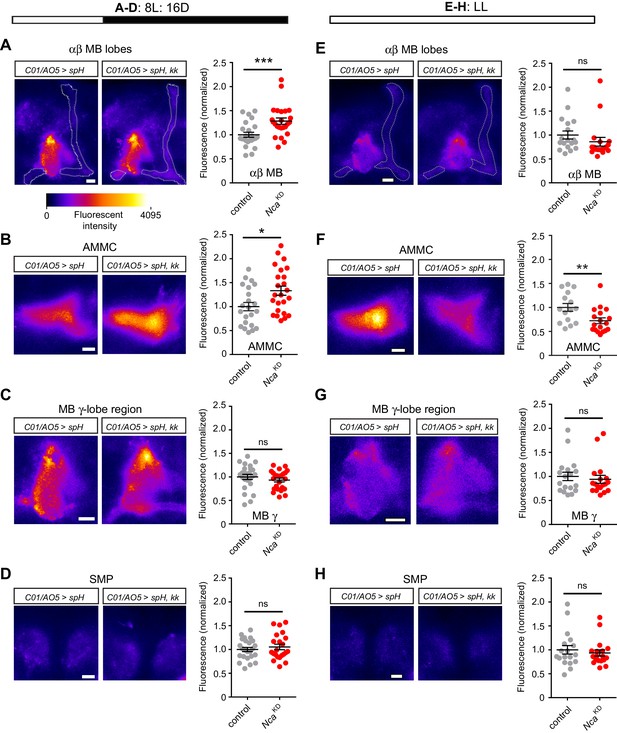
NCA suppresses synaptic release in subsets of C01/A05-neurons during darkness.
(A–D) Fluorescence of an optical reporter of synaptic release (synapto-pHluorin, spH) in neuropil regions labelled by the C01- and A05-drivers, in control adult males (C01/A05 > spH) or following Nca knockdown in C01- and A05-neurons (C01/A05 > spH, kk). Flies were housed under 8L: 16D conditions, in which Nca knockdown in C01- and A05-neurons causes robust nighttime sleep loss. (E–G) spH fluorescence in control adult males or following Nca knockdown in C01- and A05-neurons (C01/A05 > spH, kk). Flies were housed in LL conditions, in which Nca knockdown in C01- and A05-neurons has no effect on sleep levels. In each panel, representative confocal images of spH fluorescence (left) and mean fluorescent intensity (right, normalized to the mean of C01/A05 > spH controls) are shown. Dots within dot plots represent individual brain hemisphere measurements. A-D: n = 22–24. E-H: n = 15–18. Neuropil regions are noted. MB: mushroom body. AMMC: antennal mechanosensory motor center. SMP: superior medial protocerebrum. ns – p>0.05, *p<0.05, **p<0.01, ***p<0.001, Mann-Whitney U-test.
-
Figure 7—source data 1
Normalized synaptopHluorin fluorescence in specified neuropil regions (see Figure 7) in a wild-type background or following Nca knockdown in C01- and A05-neurons, in either 8L: 16D or in constant light (LL).
- https://doi.org/10.7554/eLife.38114.027
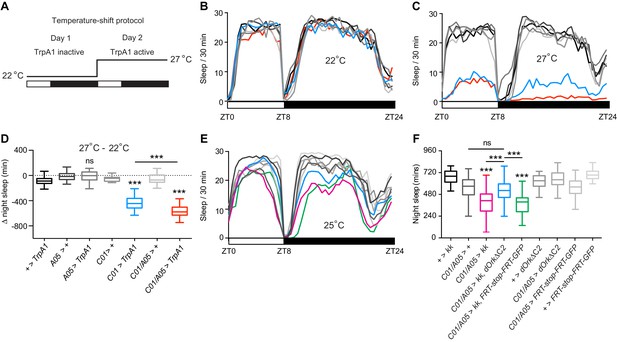
Sleep loss in Nca knockdown flies is caused by enhanced excitability of C01/A05-neurons.
(A) Experimental paradigm for acute activation of A05 or C01-neurons. 22°C: non-activating temperature for TrpA1. 27°C: activating temperature. Sleep levels were recorded over two days in 8L: 16D conditions. (B–C) Mean sleep levels across 8L: 16D following expression of TrpA1 in A05-, C01- or A05- and C01-neurons (and associated controls) at 22°C (B) or 27°C (C). (D) Median change in night sleep levels (Δ night sleep) following the shift from 22°C on day 1°C to 27°C on day 2. + > TrpA1: n = 53, A05 > +: n = 23, A05 > TrpA1: n = 68, C01 > +: n = 24, C01 > TrpA1: n = 40, C01/A05 > +: n = 33, C01/A05 > TrpA1: n = 40. ns – p>0.05, ***p<0.001, as compared to TrpA1 or driver alone controls by Kruskal-Wallis test with Dunn’s post-hoc test (for C01 > TrpA1, A05 > TrpA1, or C01/A05 > TrpA1 compared to controls) or Mann-Whitney U-test (for C01/A05 > TrpA1 compared to C01 > TrpA1). (E–F) Inhibition of C01/A05-neurons by expressing dORKΔC2 rescues sleep loss due to Nca knockdown, while expression of dORKΔC2 does not change baseline sleep. Mean sleep patterns in 8L: 16D conditions are shown in (E). Median night sleep levels are shown in (F).+ > kk: n = 72, C01/A05 > +: n = 85, C01/A05 > kk: n = 95, C01/A05 > dORKΔC2, kk: n = 77, C01/A05 > kk, FRT-stop-FRT-GFP: n = 39, + > dORKΔC2: n = 57, C01/A05 > dORKΔC2: n = 73, C01/A05 > FRT-stop-FRT-GFP: n = 49, + > FRT-stop-FRT-GFP: n = 36. ns – p>0.05, ***p<0.001, Kruskal-Wallis test with Dunn’s post-hoc test.
-
Figure 8—source data 1
Sleep levels following excitation or inhibition of C01- and A05-neurons (simultaneously or in isolation), either in a wild type background or in parallel to Nca knockdown.
Blank cells represent data from dead flies removed prior to analysis.
- https://doi.org/10.7554/eLife.38114.029
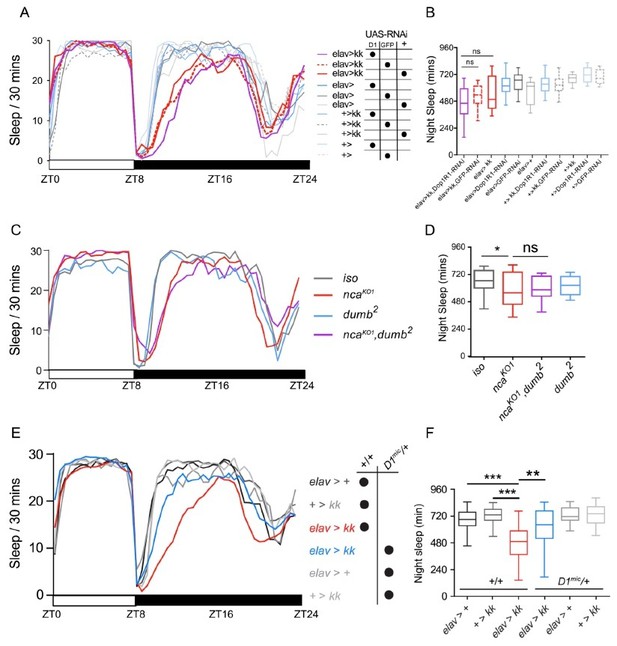
Sleep loss in NcaKO or NcaKD flies is not modified by altered expression of Dop1R1.
(A-B) Night sleep levels in NcaKD (elav > kk) adult males co-expressing control UASGFP RNAi (GFP, dashed red lines) are significantly reduced compared to controls (grey lines).However, this profile does not differ from flies co-expressing UAS-Dop1R1 and kk (Nca) RNAi (D1, purple lines). Mean sleep levels are shown in (A) across 24 h in L8: D16 conditions, while median night sleep levels are shown in (B). n = 16-24. (C-D) Night sleep levels in NcaKO (red lines) flies are reduced as compared to iso31 controls (grey line). However, this sleep loss is not suppressed by homozygosity for the hypomorphic dumb2 allele of Dop1R1 (purple line). n = 19-24. (E-F) heterozygosity of the non-isogenized hypomorphic Dop1R1 allele Dop1R1MI03085-GFST.2(D1mic/+) suppressed sleep loss in NcaKD adult males. Mean sleep patterns were shown in the original Figure 2G. Median total night sleep levels were shown in the original Figure 2H. n = 15-48. *p < 0.05, ** p <0.01, *** p <0.001, ns– p > 0.05, Kruskal-Wallis test with Dunn’s post-hoc test. Control and experimental genotypes are indicated by color scheme and black dots.
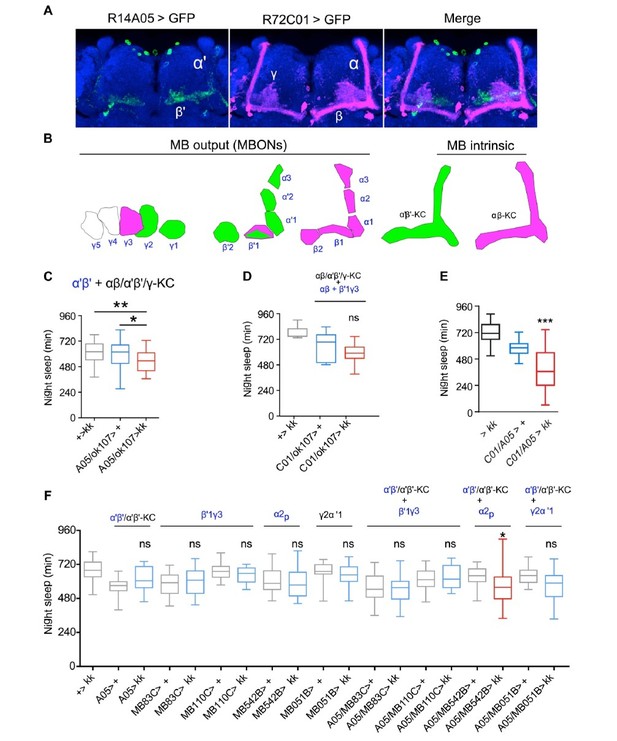
Modestcontribution of MB output neurons (MBONs) to NCA-mediated sleep.
(A) Confocal stacks labelling R14A05- (A05, green) and R72C01- (C01, magenta) positive neurons. Images are from (Jenett et al., 2012) and deposited at Virtual Fly Brain (www.virtualflybrain.org). Images were downloaded and digitally superimposed (Merged) onto a standardised fly brain (active zones are labelled in blue using the nc82 antibody; the image source data is distributed under a CC BY-NC-SA 4.0 license). (B) Tiling scheme of MBONs adapted from (Aso et al., 2014). The A05- (green) and C01-Gal4 (magenta) drivers potentially label complementary parts of MBONs (blue letters), though there is potential limited overlap within the β’1 region. C01-Gal4 clearly labels the αβ lobe of MB intrinsic Kenyon cells αβ-KC, black letters), while A05-Gal4 labels α’β’-KC. (C) Simultaneous Nca knockdown in A05-neurons (potentially including α’β’-KC and α’β’-MBONs) and all KC neurons (using ok107Gal4) results in limited night sleep loss. (D) Simultaneous Nca knockdown in C01-neurons (including αβ-KC and γ3-MBONs) and all KC neurons (using ok107-Gal4) did not result in significant night sleep loss. (E) Nca knockdown in both A05- and C01-positive neurons results in robust night sleep loss. For (C-E), please see Figure 5—figure supplement 2G for nvalues. (F) Knockdown of Nca in A05-neurons (which potentially include α’β’-KCs and α’β’MBONs) and in 3 distinct MBON regions potentially labelled by A05-Gal4 (γ2α’1) or C01-Gal4 (β’1γ3 and α2p) does not cause sleep loss. Simultaneous Nca knockdown in both A05-neurons and in α2p MBONs results in modest sleep loss (A05/MB542B > kk, red). In contrast, no sleep reduction was observed following Nca knockdown in A05 (β’1γ3 MBON or A05/γ2α’1 MBONs. n = 16-28. *p < 0.05, **p < 0.01, ***p < 0.001, ns – p > 0.05, compared to driver and RNAi alone controls by Kruskal-Wallis test with Dunn’s post-hoc test.

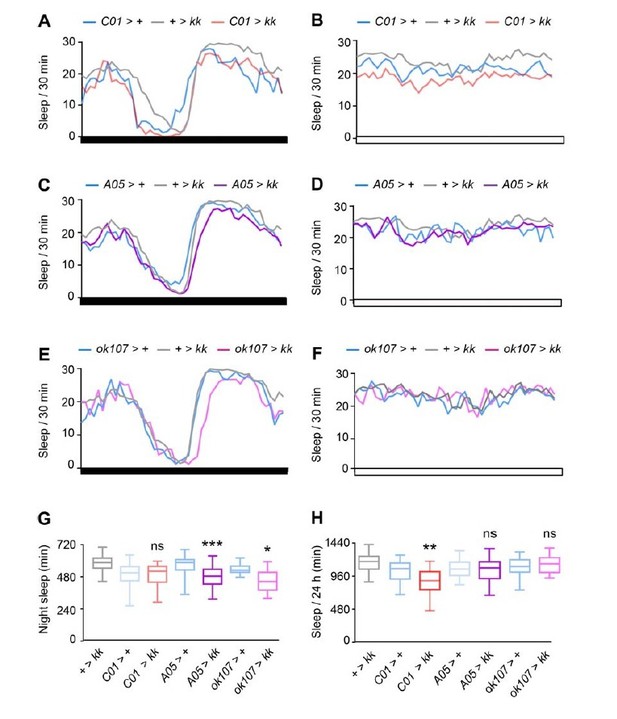
Effect of Nca knockdown in C01-, A05- and ok107-neurons on sleep in LL and DD.
(A-B) Mean sleep patterns following Nca knockdown in C01-neurons in constant dark (DD) (A) and constant-light (B) conditions. A: + > kk, n = 64, C01 > +, n = 24, C01 > kk, n = 24. B: + > kk, n = 76, C01 > +, n = 51, C01 > kk, n = 52.(C-D) Mean sleep patterns following Nca knockdown in A05-neurons in constant dark (DD) (C) and constant-light (D) conditions. C: + > kk, n = 64, A05 > +, n = 51, A05 > kk, n = 54. D: + > kk, n = 76, A05 > +, n = 28, A05 > kk, n = 32.(E-F) Mean sleep patterns following Nca knockdown in ok107-neurons in constant dark (DD) (E) and constant-light (F) conditions. E: + > kk, n = 64, ok107 > +, n = 24, ok107 > kk, n = 19. F: + > kk, n = 76, ok107 > +, n = 24, ok107 > kk, n = 21.(G-H) Median subjective night sleep (G) or total sleep (H) for the above genotypes in either constant-dark (G) or constant light (H). ns – p > 0.05, *p < 0.05, **p < 0.01, ***p < 0.001, compared to driver and RNAi alone controls, Kruskal-Wallis test with Dunn’s post-hoc test.
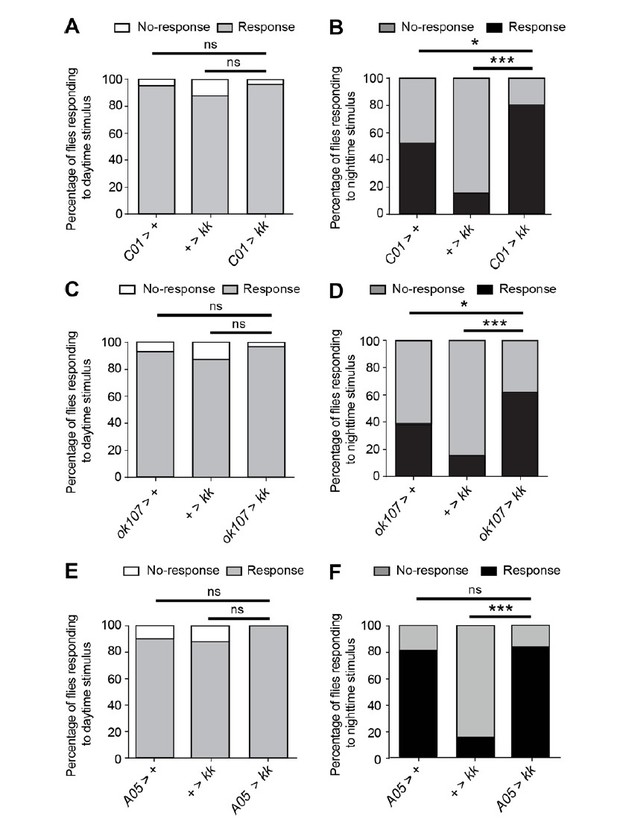
NCA acts in the mushroom bodies to regulate nocturnal arousal.
(A-B) Percentage of adult male flies expressing Nca RNAi (kk) in C01-neurons (C01 > kk) and control flies responding or not responding to vibration stimulus at either ZT4 (day; A) or ZT16 (night; B). ZT4: C01 > +, n = 22, + > kk, n = 61, C01 > kk, n = 27. ZT16: C01 > +, n = 19, + > kk, n = 54, C01 > kk, n = 21. (C-D) Percentage of adult male flies expressing Nca RNAi (kk) in MB-KCs (ok107 > kk) and control flies responding or not responding to vibration stimulus at either ZT4 (day; C) or ZT16 (night; D). ZT4: ok107 > +, n = 26, + > kk, n = 47, ok107 > kk, n = 28. ZT16: ok107 > +, n = 26, + > kk, n = 44, ok107 > kk, n = 27. (D-E) Percentage of adult male flies expressing Nca RNAi (kk) in A05-neurons (A05 > kk) and control flies responding or not responding to vibration stimulus at either ZT4 (day; A) or ZT16 (night; B). ZT4: A05 > +, n = 19, + > kk, n = 61, A05 > kk, n = 29. ZT16: A05 > +, n = 20, + > kk, n = 54, A05 > kk, n = 31.
ns – p > 0.05, *p < 0.05, ***p < 0.001, Binomial test with Bonferonni correction for multiple comparisons.
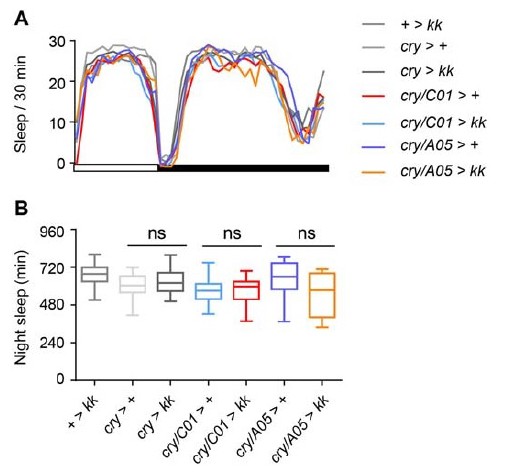
NCA expression in cry-neurons is not required for NcaKD-mediated sleep loss.
(A-B) Mean sleep pattern in 8L: 16D conditions (A) and median night sleep (B) for the indicated genotypes (see text). n= 20-51, ns – p > 0.05, Kruskal-Wallis test with Dunn’s post-hoc test.

Constant light increases total sleep in adult male Drosophila.
Median total sleep across 24 h in either constant-dark (DD) or constant-light (LL) are shown for adult males from four different genetic backgrounds. n = 15-64. *p < 0.05, ***p < 0.001, Kruskal-Wallis test with Dunn’s post-hoc test, with LL compared to both LD and DD.
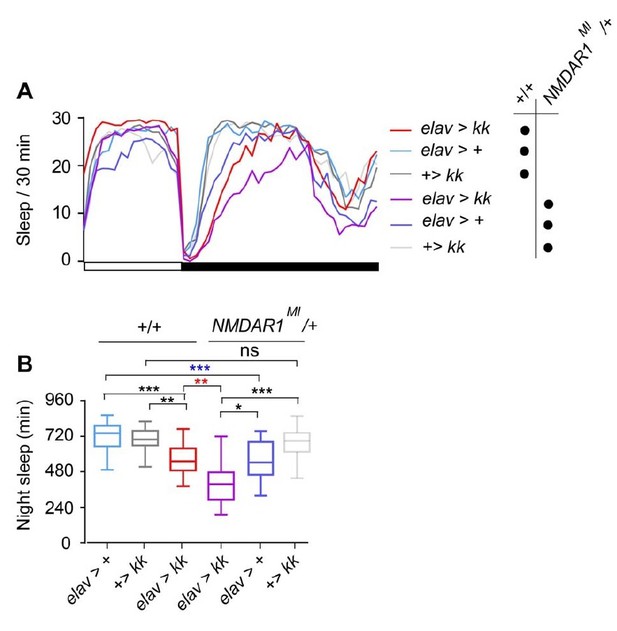
Nca and NMDAR1 mutations additively affect sleep.
(A) Mean sleep patterns of NcaKD males (elav > kk) and heterozygous controls with and without one copy of the NMDAR1MI11796 (NMDAR1MI/+) allele in 8L: 16D conditions. (B) Median night sleep levels in the above genotypes. Heterozygosity for NMDAR1MI11796 resulted in sleep loss in the background of elav-Gal4/+ controls, and reduced sleep further in an additive manner in elav > kk males. elav > kk; NMDAR1MI/+: n = 32; elav > +; NMDAR1MI/+: n = 26; + > kk; NMDAR1MI/+n = 38; elav > kk: n = 32; elav > +: n = 30; + > kk: n = 34. ns – p > 0.05, *p < 0.05, **p < 0.01, ***p <0.001, Kruskal-Wallis test with Dunn’s post-hoc test.
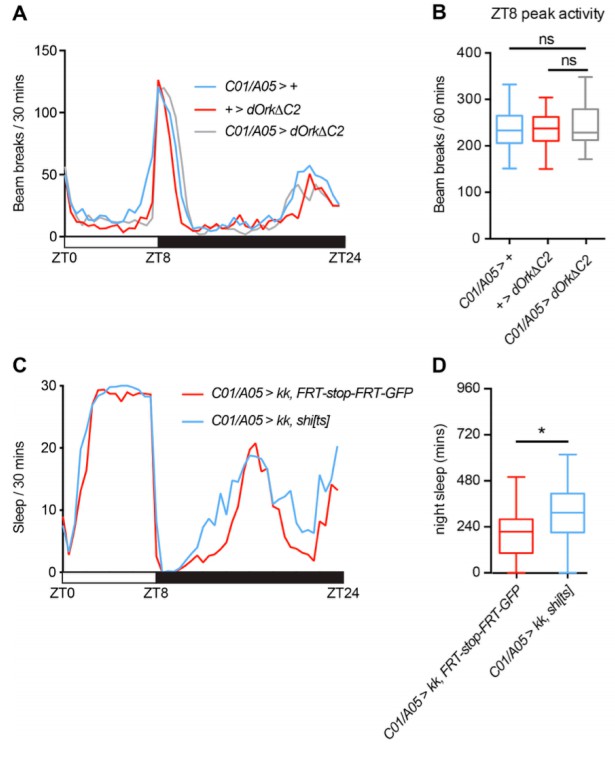
Acute silencing of C01- and A05-neurons suppresses sleep loss induced by Nca knockdown.
(A) Activity in adult male flies expressing UAS-dORK-∆C2 in C01/A05-neurons, as quantified by infrared beam breaks using the DAM system. Driver and transgene alone controls are also shown. (B) Box plots comparing median peak activity at ZT8 (the evening activity peak). No significant difference in peak activity was found following constitutive silencing of C01/A05-neurons. C01/A05 > +, n = 38; + > dORK-∆C2, n = 26, C01/A05 > dORK-∆C2, n = 34. ns: p>0.05, Kruskal-Wallis test with Dunn’s post-hoc test. (C-D) Average sleep patterns (C) and total night sleep levels (D) in 8L: 16D at 31ºC of adult males expressing kk Nca RNAi in C01/A05-neurons alongside the acute inhibitor of synaptic vesicle endocytosis (shi[ts]) or a control transgene (FRT-stop-FRT-GFP). Note the significant increase in night sleep upon inhibiting synaptic release from C01/A05-neurons with reduced NCA levels (D). C01/A05 > kk, FRT-stop-FRT-GFP, n = 25; C01/A05 > kk, shi[ts] n = 22. *p

Median night sleep levels following pan-neuronal expression of two independent RNAi lines targeting cg7646 mRNA compared to driver (elavGal4/+) and RNAi transgene alone controls.
No significant difference in night sleep following cg7646 knockdown using either RNAi construct. n = 12-15. Ns: p>0.05, Kruskal-Wallis test with Dunn’s post-hoc test.
Tables
Reagent type (species) or resource | Designation | Source or reference | Identifiers | Additional information |
---|---|---|---|---|
Genetic reagent (Drosophila melanogaster) | kk108825 | Vienna Drosophila Resource Center | RRID:FlyBase_FBst0481000 | |
Genetic reagent (Drosophila melanogaster) | y[1]v[1]; P{y[+t7.7] v[+t1.8]=TRiP.HMJ21533}attP40 | Bloomington Stock Center | RRID:BDSC_54814 | |
Genetic reagent (Drosophila melanogaster) | y[1]v[1]; P{y[+t7.7] v[+t1.8]=TRiP.JF03398}attP2 | Bloomington Stock Center | RRID:BDSC_29461 | |
Genetic reagent (Drosophila melanogaster) | w[*]; P{w[+mC]=ple-GAL4.F}3 | Bloomington Stock Center | RRID:BDSC_8848 | |
Genetic reagent (Drosophila melanogaster) | w[1118];P{w[+mC]=ChAT-GAL4.7.4}19B/CyO, P{ry[+t7.2]=sevRas1 .V12}FK1 | Bloomington Stock Center | RRID:BDSC_6798 | |
Genetic reagent (Drosophila melanogaster) | w[1118]; P{w[+mW.hs]=GawB}VGlut[OK371] | Bloomington Stock Center | RRID:BDSC_26160 | |
Genetic reagent (Drosophila melanogaster) | P{w[+mC]=Gad1 GAL4.3.098}2/CyO | Bloomington Stock Center | RRID:BDSC_51630 | |
Genetic reagent (Drosophila melanogaster) | w[1118]; P{w[+mC]=Ddc-GAL4.L}4.3D | Bloomington Stock Center | RRID:BDSC_7010 | |
Genetic reagent (Drosophila melanogaster) | w[*]; P{w[+mC]=GAL4 ninaE.GMR}12 | Bloomington Stock Center | RRID:BDSC_1104 | |
Genetic reagent (Drosophila melanogaster) | w[1118]; P{w[+mC]=Trh-GAL4.long}2 | Bloomington Stock Center | RRID:BDSC_38388 | |
Genetic reagent (Drosophila melanogaster) | w[*]; P{w[+mC]=Tdc2 GAL4.C}2 | Bloomington Stock Center | RRID:BDSC_9313 | |
Genetic reagent (Drosophila melanogaster) | w[*]; P{w[+mW.hs]=GawB}cv-c[C5] | Bloomington Stock Center | RRID:BDSC_30839 | |
Genetic reagent (Drosophila melanogaster) | w[*]; P{w[+mW.hs]=GawB}OK107 ey[OK107]/In(4)ci[D], ci[D] pan[ciD] sv[spa-pol] | Bloomington Stock Center | RRID:BDSC_854 | |
Genetic reagent (Drosophila melanogaster) | y[1] w[1118]; PBac{w[+mC]=5HPw[+]}Nca[A502] | Bloomington Stock Center | RRID:BDSC_16130 | |
Genetic reagent (Drosophila melanogaster) | w[1118]; P{y[+t7.7] w[+mC]=GMR23E10-GAL4}attP2 | Bloomington Stock Center | RRID:BDSC_49032 | |
Genetic reagent (Drosophila melanogaster) | w[1118]; P{y[+t7.7] w[+mC]=GMR55B01-GAL4}attP2 | Bloomington Stock Center | RRID:BDSC_39100 | |
Genetic reagent (Drosophila melanogaster) | w[1118]; P{y[+t7.7] w[+mC]=GMR52 H12-GAL4}attP2 | Bloomington Stock Center | RRID:BDSC_38856 | |
Genetic reagent (Drosophila melanogaster) | w[1118]; P{y[+t7.7] w[+mC]=GMR17 F12-GAL4}attP2 | Bloomington Stock Center | RRID:BDSC_48779 | |
Genetic reagent (Drosophila melanogaster) | w[1118]; P{y[+t7.7] w[+mC]=GMR72B05-GAL4}attP2 | Bloomington Stock Center | RRID:BDSC_39611 | |
Genetic reagent (Drosophila melanogaster) | w[1118]; P{y[+t7.7] w[+mC]=GMR72B07-GAL4}attP2 | Bloomington Stock Center | RRID:BDSC_39764 | |
Genetic reagent (Drosophila melanogaster) | w[1118]; P{y[+t7.7] w[+mC]=GMR72B08-GAL4}attP2 | Bloomington Stock Center | RRID:BDSC_46669 | |
Genetic reagent (Drosophila melanogaster) | w[1118]; P{y[+t7.7] w[+mC]=GMR72 C01-GAL4}attP2 | Bloomington Stock Center | RRID:BDSC_41358 | |
Genetic reagent (Drosophila melanogaster) | w[1118]; P{y[+t7.7] w[+mC]=GMR72 C01-GAL4}attP2 | Bloomington Stock Center | RRID:BDSC_47729 | |
Genetic reagent (Drosophila melanogaster) | w[1118]; P{y[+t7.7] w[+mC]=GMR72 C02-GAL4}attP2/TM3, Sb[1] | Bloomington Stock Center | RRID:BDSC_46672 | |
Genetic reagent (Drosophila melanogaster) | w[1118]; P{y[+t7.7] w[+mC]=GMR78B07-GAL4}attP2 | Bloomington Stock Center | RRID:BDSC_39989 | |
Genetic reagent (Drosophila melanogaster) | w[1118]; P{y[+t7.7] w[+mC]=GMR88A06-GAL4}attP2 | Bloomington Stock Center | RRID:BDSC_46847 | |
Genetic reagent (Drosophila melanogaster) | w[1118]; P{y[+t7.7] w[+mC]=GMR91A07-GAL4}attP2/TM3, Sb[1] | Bloomington Stock Center | RRID:BDSC_47147 | |
Genetic reagent (Drosophila melanogaster) | cg7674 RNAi 1 (chromosome III) | NIG-FLY stock center | Accession number: NM_140910.2 | |
Genetic reagent (Drosophila melanogaster) | cg7674 RNAi 2 (chromosome II) | NIG-FLY stock center | Accession number: NM_140910.2 | |
Genetic reagent (Drosophila melanogaster) | nompC-Gal4 | Kamikouchi et al., 2009 | ||
Genetic reagent (Drosophila melanogaster) | inc-Gal4:2 | Stavropoulos and Young, 2011 | ||
Genetic reagent (Drosophila melanogaster) | ppk-Gal4 | Zhong et al., 2012 | ||
Genetic reagent (Drosophila melanogaster) | TrpA1-CD-Gal4 | Zhong et al., 2012 | ||
Genetic reagent (Drosophila melanogaster) | timKO | Lamaze et al., 2018 | ||
Genetic reagent (Drosophila melanogaster) | GMR14A05-Gal4 | Janelia Research Campus FlyLight Project | 26432 | |
Genetic reagent (Drosophila melanogaster) | w[1118];+; Nca[ko1]/TM2 | This paper | Null allele of Nca | |
Genetic reagent (Drosophila melanogaster) | w[1118];+; Nca[ko2]/TM2 | This paper | Nca null allele (second allele) | |
Genetic reagent (Drosophila melanogaster) | w[1118];+; Nca[ko3]/TM2 | This paper | Nca null allele (third allele) | |
Strain, strain background (Drosophila melanogaster) | Canton-S | Bloomington Stock Center | RRID:BDSC_64349 | |
Antibody | Rabbit anti-DsRed | Clontech | RRID:AB_10013483 | (1:2000) |
Antibody | Mouse anti-Bruchpilot | Developmental Studies Hybridoma Bank | RRID:AB_2314866 | (1:200) |
Antibody | Rabbit anti-GFP | Invitrogen | RRID:AB_221569 | (1:1000) |
Antibody | Goat anti-Mouse Alexa Fluor-647 | ThermoFisher | RRID:AB_141725 | (1:500) |
Antibody | Alexa Fluor 488 goat anti-rabbit IgG | ThermoFisher | RRID:AB_2576217 | (1:2000) |
Antibody | Alexa Fluor 555 goat anti-rabbit IgG | ThermoFisher | RRID:AB_2633281 | (1:2000) |
Antibody | DAPI | Sigma-Aldrich | D9542-10MG | |
Commercial assay or kit | Wizard SV Gel and PCR Clean-Up System | Promega | Cat. #: A9281 | |
Commercial assay or kit | Zero Blunt TOPO PCR Cloning Kit | ThermoFisher Scientific | Cat. #: 450245 | |
Commercial assay or kit | TRIzol | ThermoFisher Scientific | Cat. #: 15596026 | |
Commercial assay or kit | MMLV RT | Promega | Cat. #: M170A | |
Commercial assay or kit | Power SYBR Green Master Mix | ThermoFisher Scientific | Cat. #: 4367659 |
Additional files
-
Transparent reporting form
- https://doi.org/10.7554/eLife.38114.030