Structural organization of a major neuronal G protein regulator, the RGS7-Gβ5-R7BP complex
Figures
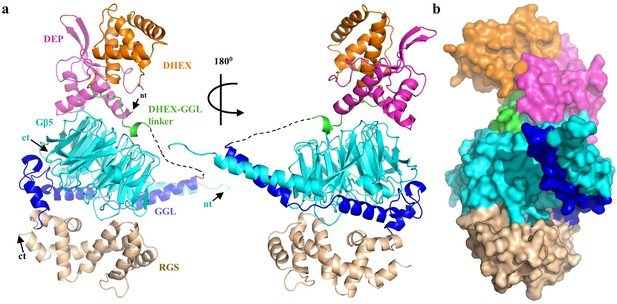
Overall structure of RGS7-Gβ5.
(a) Cartoon representation of the RGS7-Gβ5 complex with the RGS7 domains colored individually (DEP, pink; DHEX, orange; GGL, blue; RGS, sand). Gβ5 is shown in cyan and is sandwiched between the RGS7 domains. The missing part of the DHEX-GGL linker is shown as a dotted line. (b) Overall surface representation of the RGS7-Gβ5 complex colored as in panel A.
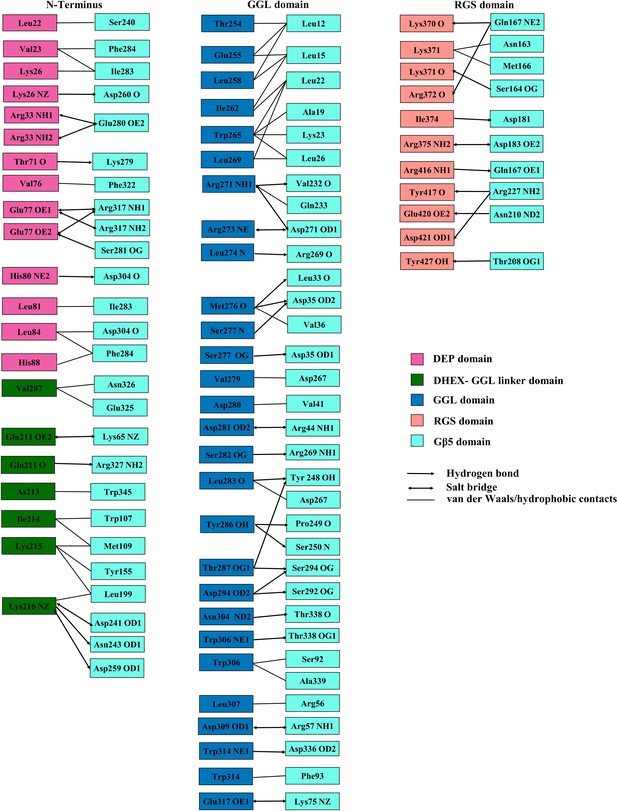
Contacts between residues of the RGS7 domains and Gβ5.
Schematic showing the specific contacts formed between residues of the RGS7 domains and Gβ5. The color coding for the RGS7 domains and Gβ5 are shown on right side along with arrows and lines indicating the type of contact.
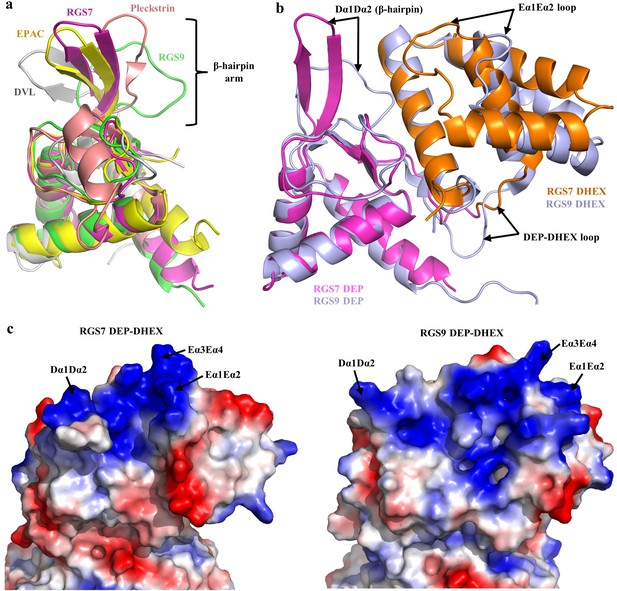
Architecture of the DEP-DHEX module.
(a) Superposition of the DEP domains of RGS7 (pink), RGS9 (green; PDB entry 2pbi), EPAC (yellow; PDB entry 2byv), pleckstrin (brown; PDB entry 1w4m), and Dvl (grey; PDB entry 1fsh) reveals diversity in the conformational organization of the β-hairpin arm. (b) Comparison of the DEP-DHEX domains of RGS7 and RGS9 shows differences in several loops and in the orientation of the DHEX domain. In RGS7, the DHEX domain is lifted upward thus generating a wide space between the domains. (c) Evaluation of the electrostatic surface potentials of the DEP-DHEX domains of RGS7 (left) and RGS9 (right). A strong basic patch (blue) is formed by three loops in the RGS7 structure but differences in the organization of these loops result in a more scattered distribution of basic charges in the RGS9 structure.
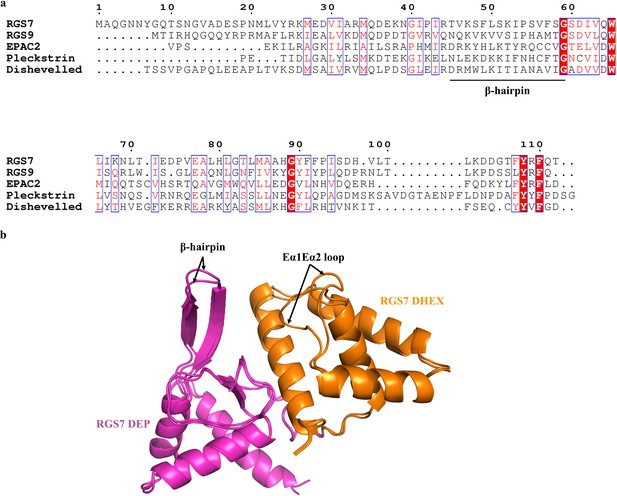
Homology of the DEP domains and loop flexibility in the DEP-DHEX domain of RGS7.
(a) Multiple sequence alignments of the DEP domain containing proteins. The β-hairpin shows no sequence conservation. The DEP domain sequences are taken from the structures of RGS7, RGS9 (PDB entry 2pbi), EPAC (PDB entry 2byv), pleckstrin (PDB entry 1w4m), and Dvl (PDB entry 1fsh). This Figure was generated with ESPript 3.0. (b) Comparison of the loop flexibilities of the DEP-DHEX domains of RGS7. Superposition of the two molecules in the asymmetric unit of the RGS7-Gβ5 dimer shows the loop variability in the β-hairpin and the Eα1Eα2 loop is indicated by black arrows. The DEP and DHEX domains are colored in pink and orange, respectively.
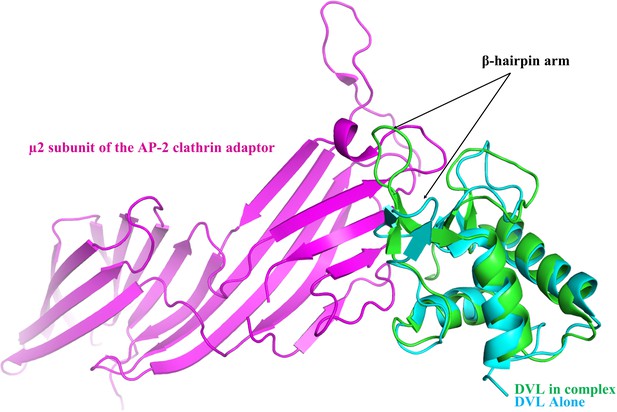
Comparison of the Dvl2 DEP domain with the Dvl2 DEP domain-µ2 complex.
Cartoon representation of the superposed Dvl2 DEP domain (cyan, PDB entry 1fsh) with the Dvl2 DEP domain (green)-µ2 subunit of the AP-2 clathrin adaptor (pink) complex (PDB entry 3ml6) is shown. The involvement in the complex formation and conformational changes of the β-hairpin arm are indicated by a black arrow.
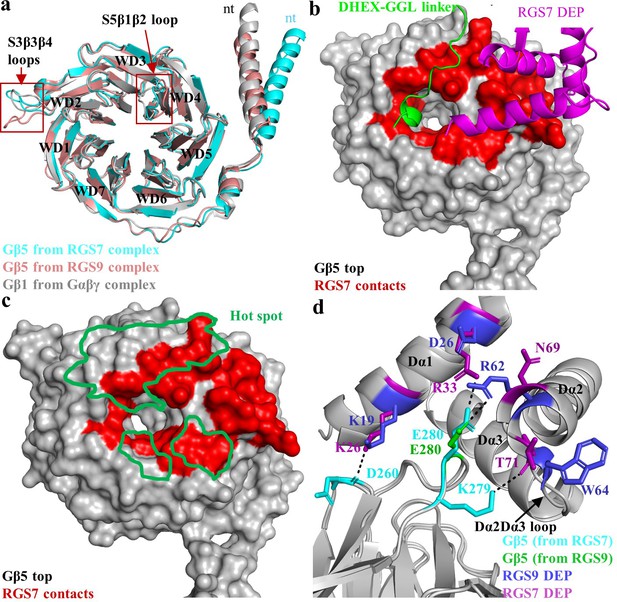
Interaction of RGS7 with Gβ5.
(a) Cartoon representation of the superposed Gβ5 structures from RGS7 with RGS9 and Gβ1. The differences between the loop regions are highlighted in red boxes. The N-terminal α-helix of Gβ5 from RGS7 also shows variation compared to Gβ5 from the RGS9 complex and Gβ1 from the Gαβγ complex. (b) Surface representation of Gβ5 top face (grey) highlighting the RGS7 contact residues (red). Two contact interfaces that are formed by the DHEX-GGL linker and the DEP domain are shown as a carton in green and pink, respectively. (c) The hotspot region (green) mapped onto the Gβ5 surface based on contacts formed by known Gβ1 binding partners. RGS7 (red) overlaps with the hotspot footprint. The DEP domain forms a unique contact surface on the top face of Gβ5. (d) Distinct interacting residues of the DEP domain of RGS7 (pink) that forms direct contacts with Gβ5 (cyan) are shown along with equivalent residues of RGS9. A unique electrostatic interaction is formed by Dα1 residue R33 of the RGS7 DEP domain with E280. Its organizational equivalent in RGS9 is formed by interactions with R62 from α-helix Dα2. The Dα2-Dα3 loop of the DEP domain that is involved in interactions with Gβ5 has a different organization in RGS7 and RGS9 and is indicated by an arrow.
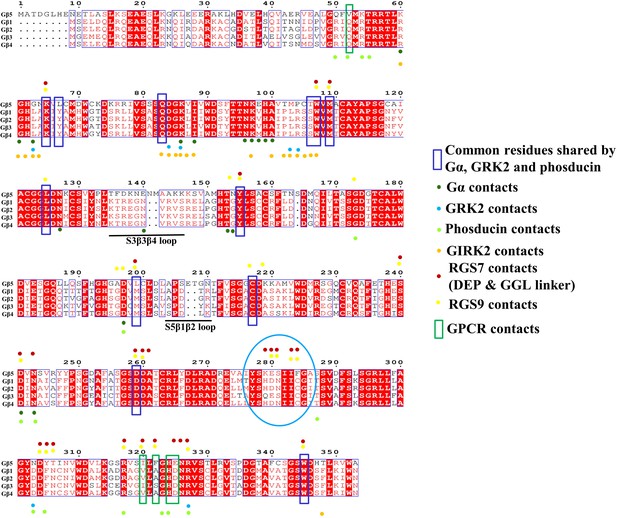
Gβ subunit sequence alignments and contact residues with effector molecules.
Multiple sequence alignments of the Gβ subunits. The residues in contact with the Gβ subunits with the effector molecules are plotted and shown as colored spots. Common residues in contact referred to as hotspot regions are conserved among all Gβ subunits and are shown in a blue box. GPCR contact residues are shown in a green box. The unique contacts formed by the DEP domain of RGS7 and RGS9 are shown in the cyan circle. The unique two loops specific to Gβ5 with two amino acids insertions are underlined.
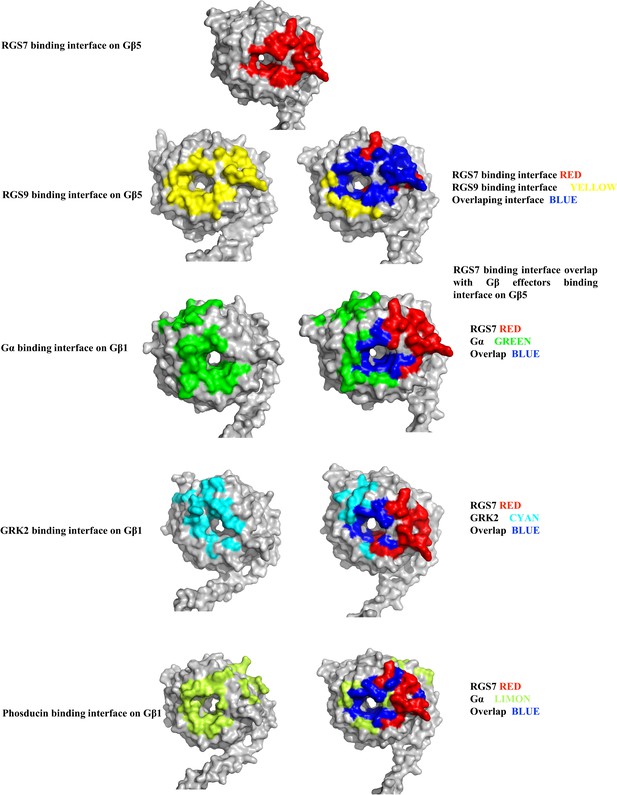
Comparison of binding interfaces of various effector molecules of the Gβ subunits with RGS7-Gβ5 by mapping the overlap onto the Gβ5 surface.
The contacts between the Gβγ effector molecule complexes are compared and mapped onto the Gβ subunits. The effector molecule contacts are mapped onto the Gβ subunits surface and shown in left and are mapped onto the Gβ5 subunits along with the RGS7 contacts in the right panel. The overlap between the interfaces are colored in blue. The β subunit were taken from respective complex structure of RGS9-Gβ5 (PDB entry 2pbi), Gαβγ trimer complex (PDB entry 1gp2), GRK2-Gβγ complex (PDB entry 1omw), and phosducin-Gβγ complex (PDB entry 1b9y).
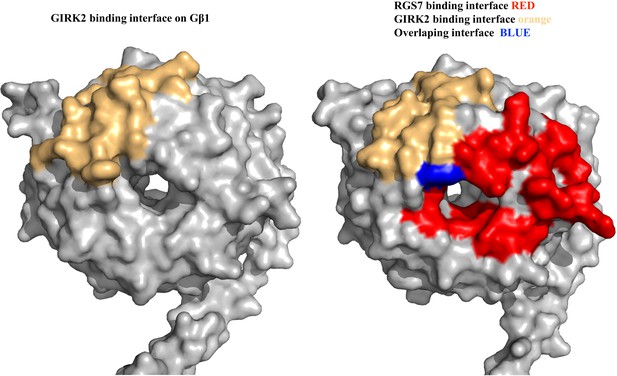
Comparison of the binding interfaces of RGS7 with GIRK2 of the Gβ subunits.
GIRK2 and RGS7 are in contact with the Gβ subunit. Left, GIRK2 is in contact with the Gβ1 subunit from the GIRK2-Gβ1γ2 complex (PDB entry 4kfm) and mapped onto the Gβ1 surface. Right, the GIRK2 and RGS7 contacts are mapped onto the Gβ5 surface and the overlap is shown in blue.
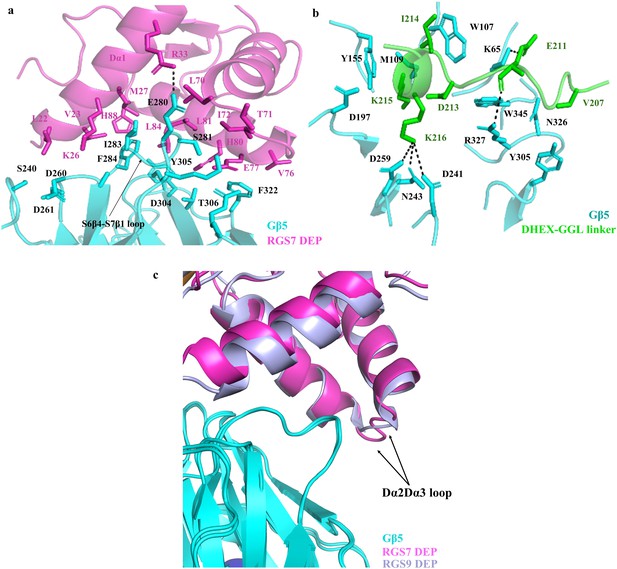
Interaction of RGS7 with the Gβ5 subunit.
Detailed view of the interface formed by Gβ5 with residues of (a) the DEP domain and (b) the DHEX-GGL linker, represented as a cartoon. Hydrogen bonds are shown in black dotted lines. (c) Cartoon representation of the superposed DEP domains of RGS7 (pink) and RGS9 (light blue) interfaced with Gβ5. The different organization of the Dα2-Dα3 loop of the DEP domains is shown by an arrow.
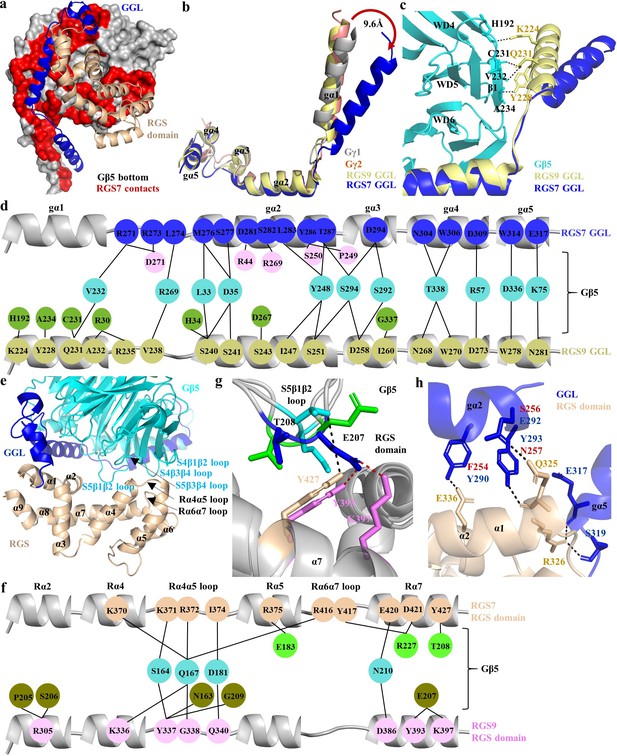
Unique mode of the Gβ5 interaction with the GGL domain of RGS7.
(a) Surface representation of Gβ5 (bottom face; grey) with RGS7 contact residues (red) formed by GGL (glue) and the RGS domain (sand) is shown. (b) Comparison of the GGL domains in RGS7 and RGS9 with Gγ1 and Gγ2. (c) The Gα1 α-helix of RGS7 is located 9.6 Å away from its position in RGS9, Gγ1, and Gγ2 diminishing the contacts of the Gα1 α-helix with Gβ5. (d) Schematic view of detailed comparison of the direct contact residues of GGL of RGS7 and RGS9 with Gβ5 residues. Most of the contact residues of Gβ5 are conserved (cyan); however, the RGS7 Gα1 α-helix does not form any contacts. The Gα1-Gα2 and Gα2 α-helices contribute to forming additional contacts (pink) that consist of two electrostatic interactions which are unique to RGS7. The RGS9-specific residues of Gβ5 are shown in green color. (e) Cartoon representation of the contact interface of RGS domain (orange) with Gβ5 (cyan) and GGL (blue) are shown. The α-helices of the RGS domain are numbered as α1-α9. Loops are indicated by black arrows. (f) Schematic view of the comparison of the direct contact residues of the RGS domains of RGS7 and RGS9 with Gβ5. Common contact residues of Gβ5 are shown in cyan, RGS-specific residues in green, and RGS9 in olive. (g) The S5β1β2 loop of Gβ5 (cyan and green for the two molecules in the asymmetric unit for the RGS7 complex and blue for the RGS9 complex) contacts different residues in the RGS domains of RGS7 (orange) and RGS9 (pink). (h) Additional contacts of the RGS7 GGL domain with the RGS domain contribute to a more extensive interface compared to RGS9. The residue differences in RGS9 which cannot form hydrogen bonds are shown in red.
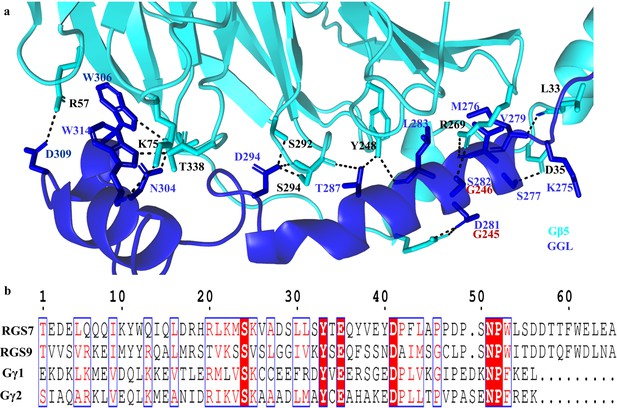
GGL domain interaction with Gβ5 and sequence alignments.
(a) Detailed view of the contact interface between RGS7 GGL and Gβ5 are shown as a cartoon representation. Specific hydrogen bonds are shown as black dotted lines. Additional contacts formed by Gα2 residues of RGS7 GGL are compensating for the lost contacts of the Gα1 α-helix. These extra contacts are absent in RGS9 due to sequence variation (red residues). (b) Multiple sequence alignments of GGL from RGS7 and RGS9, Gγ1, and Gγ2. The sequences are used from the structures of RGS7, RGS9 (PDB entry 2pbi), Gγ1 (PDB entry 1got), and Gγ12 (PDB entry 1gp2). This Figure was generated by using ESPript 3.0.
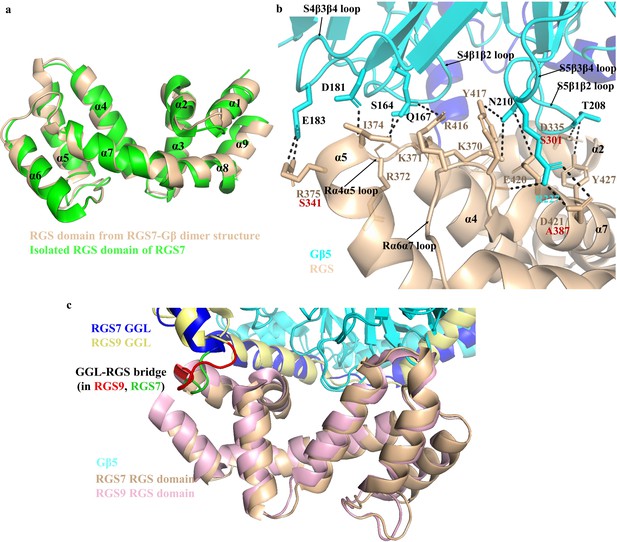
Organization of the RGS domain and its interaction with Gβ5.
(a) Cartoon representation of the superposed RGS domain from the RGS7-Gβ dimer structure with isolated RGS domains of RGS7 (PDB entry 2d9j) is shown with nine α-helices (α1-α9). (b) The interaction interface of Gβ5 (blue) and the RGS domain (sand) is shown as a cartoon. Residues involved in the interface are mostly contributed by the loops of both proteins. Several additional contacts formed by RGS7 are missing in RGS9 and the respective residues at that position are shown in red. Loops are indicated by a black arrow. GGL α-helices surrounding the Gβ5 are shown in blue. (c) The superimposition of the RGS domains of RGS7 (sand) and RGS9 (light pink) is shown as a cartoon. The GGL-RGS interaction shows a different organization in RGS7 (green) versus RGS9 (red) and is longer in RGS9.
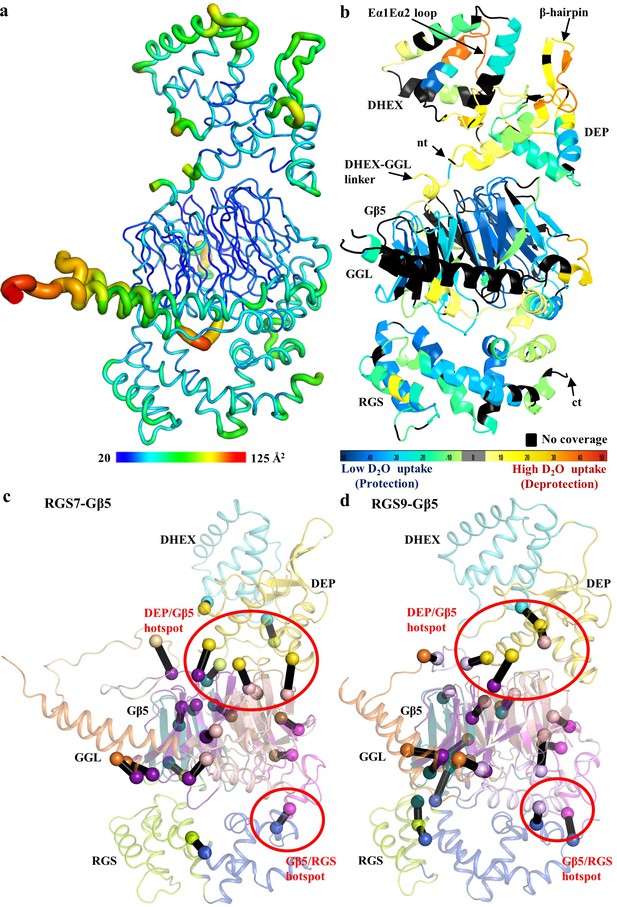
Conformational dynamics of the RGS7-Gβ5 dimer.
(a) Temperature factor (B-factor) variations plotted on the RGS7-Gβ5 structure as putty cartoon view. Colored as blue to red for low to higher B-factors, respectively. (b) Mapping baseline accessibility of peptides revealed by HDX-MS onto the crystal structure of RGS7-Gβ5. The color coding reflects that the HDX rates are higher (red) reflecting greater flexibility to lower (blue) reflecting slower conformational dynamics. (c, d) Dynamical network analysis of MD simulations performed on (c) RGS7-Gβ5 and (d) RGS9-Gβ5 reveals conserved communities or structural ‘mini-domains’ that have shared dynamical features (color coded using the same colors). And critical interaction nodes calculated between the various communities, which form dynamical signaling interfaces between communities (shown as spheres for Cα atoms, which are color coded by community linked by black lines). Red circles highlight critical nodes that form key hotspots for allosteric communication between the RGS DEP domain and Gβ5; and Gβ5 and the RGS GGL-linker and RGS domain.
-
Figure 5—source data 1
RGS7-Gβ5 communities.
- https://cdn.elifesciences.org/articles/42150/elife-42150-fig5-data1-v3.docx
-
Figure 5—source data 2
RGS7-Gβ5 critical nodes.
- https://cdn.elifesciences.org/articles/42150/elife-42150-fig5-data2-v3.docx
-
Figure 5—source data 3
RGS9-Gβ5 communities.
- https://cdn.elifesciences.org/articles/42150/elife-42150-fig5-data3-v3.docx
-
Figure 5—source data 4
RGS9-Gβ5 critical nodes.
- https://cdn.elifesciences.org/articles/42150/elife-42150-fig5-data4-v3.docx
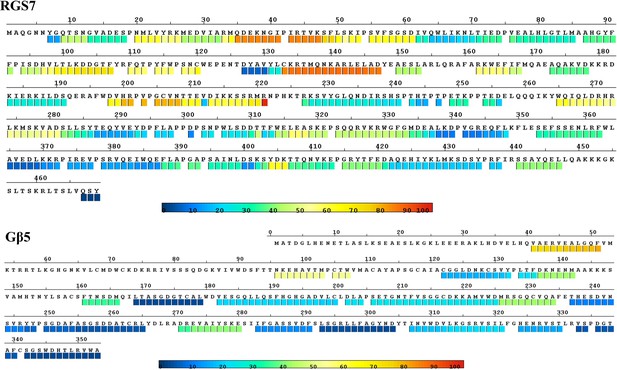
Baseline HDX mapping of RGS7 and Gβ5.
Intrinsic HDX behavior mapped on the sequence of RGS7 and Gβ5. Percentage of deuterium exchange of peptides from dimeric RGS7/Gβ5 complex. The color coding same as in Figure 5b, HDX rates are higher (red) reflecting greater flexibility and lower (blue) reflecting slower conformational dynamics. Raw HDX data are deposited at: https://doi.org/10.6084/m9.figshare.7316462.v2.
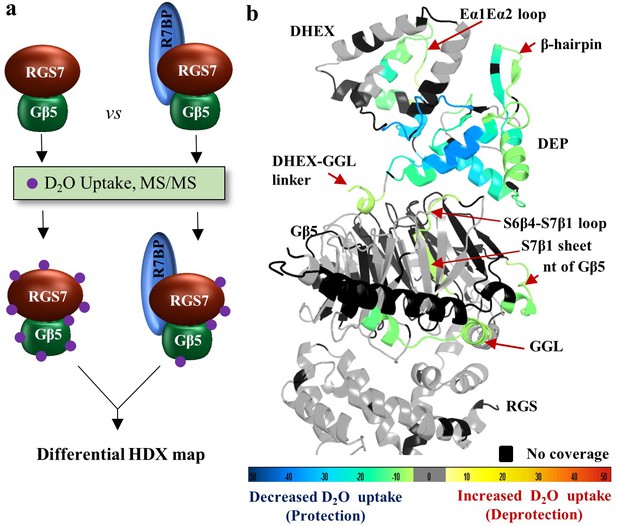
R7BP binding interface revealed by HDX-MS.
(a) Schematics of the HDX-MS experiment. Three protein samples were explored separately for the HDX-MS analyses and differential HDX data were obtained. Protection from deuterium exchange suggests the stabilization of a region upon protein-protein interaction. (b) Differential consolidation of HDX data are mapped onto the RGS7-Gβ5 dimer structure and shows stabilization of the DEP domain upon R7BP binding by protection from deuterium exchange. Altered conformational dynamics by R7BP binding are indicated by a red arrow.
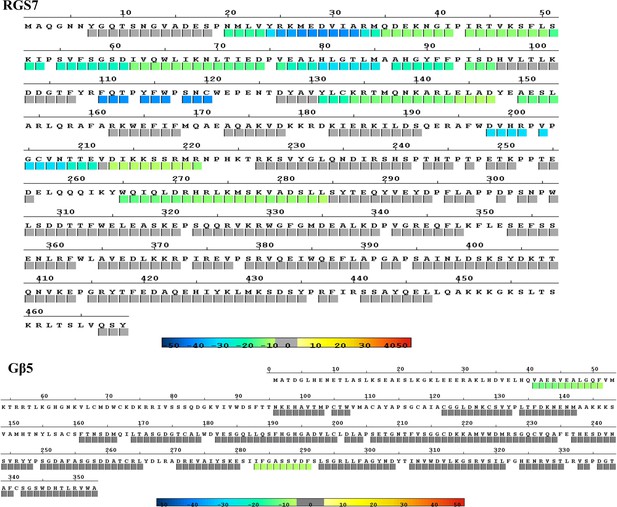
Differential mapping of changes in HDX of RGS7 and Gβ5 induced by R7BP binding.
Peptides whose HDX was significantly changed in the presence of R7BP are colored and mapped onto the RGS7 and Gβ5 sequences. Color coding as in Figure 6b. Raw HDX data are deposited at: https://doi.org/10.6084/m9.figshare.7316462.v2.
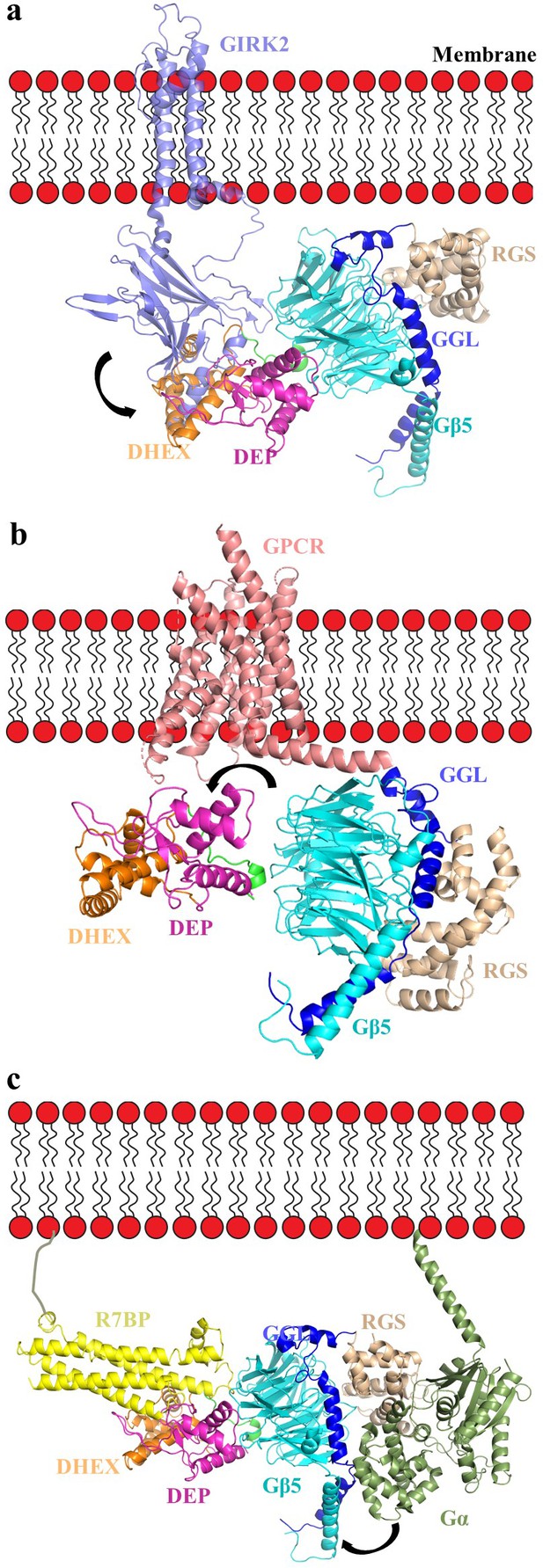
Orientation of the RGS7-Gβ5 dimer complex relative to the membrane in complex with GIRK channel, GPCR, and R7BP.
(a) A cartoon representation showing the assembly of the GIRK-RGS7-Gβ5 complex. The RGS7-Gβ5 dimer is docked onto the GIRK2-Gβγ complex (PDB entry 4kfm). Gβ5 is docked onto the Gβ1 binding interface of the GIRK channel. The respective GIRK binding site on Gβ5 is not capped by the DEP-DHEX domain however, the other part of cytoplasmic channel domain (CTCD) of GIRK clashes with the DEP-DHEX domain (arrow). (b) A model representing the GPCR-RGS7-Gβ5 assembly. The RGS7-Gβ5 dimer is docked onto GPCR similar to Gβγ in a typical GPCR-Gαβγ complex (here we used calcitonin receptor-heterotrimeric Gαs protein complex structure; PDB entry 5uz7). The DEP-DHEX domain (shown as arrow) is repositioned as it capped the probable GPCR binding interface of the Gβ5 subunit. (c) A model representing the tetrameric assembly complex of RGS7-Gβ5-R7BP-GαGTP. The complex is oriented relative to membrane according to the Gα position and its interaction with the RGS domain as well as docked to R7BP near its binding site based on HDX analysis. Structural rearrangement is required to avoid clashes of the helical domain of Gα with RGS7 GGL and Gβ5 (shown in arrow).
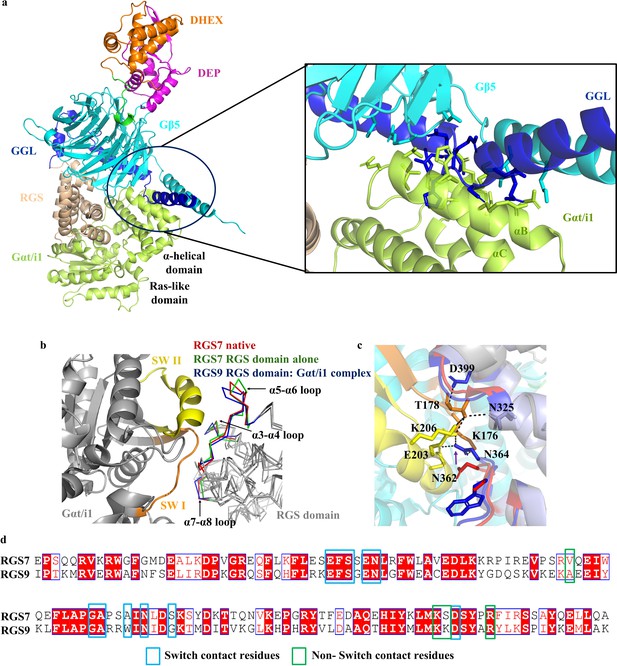
Interaction of the RGS domain with Gβ5 and probable interface of RGS-Gα.
(a) The superposition of the RGS7-Gβ5 complex onto the RGS9 RGS domain-Gαt/i1 complex structure (PDB entry 1fqk) is shown as a cartoon. The color coding for the RGS7-Gβ5 complex is as in Figure 1. Gαt/i1 is shown in green. The clashes of Gα with Gβ5 and the GGL residues are highlighted and boxed. (b) Superposition of the RGS7 domain from the RGS7-Gβ5 complex onto the RGS9 RGS domain-Gαt/i1 complex and the RGS7 RGS domain (PDB entry 2d9j) structures. The loops that are involved in interactions with the Gα subunit switch region residues are indicated by a black arrow. (c) Specific contacts formed by RGS9 RGS domain residues with switch region residues of Gαt/i1 are shown by black dotted lines. The difference of the RGS7 residue (red) and the Gα interacting RGS9 residue (blue) is shown by a black arrow suggesting that structural rearrangement occurred upon complex formation. (d) Multiple sequence alignments of the RGS domains of RGS7 and RGS9. The sequences are from the structures of RGS7 and RGS9 (PDB entry 2pbi). The Gα contacts of RGS9 residues are mapped and shown in blue and green boxes for switch and non-switch contacts, respectively. This Figure was generated with ESPript 3.0.
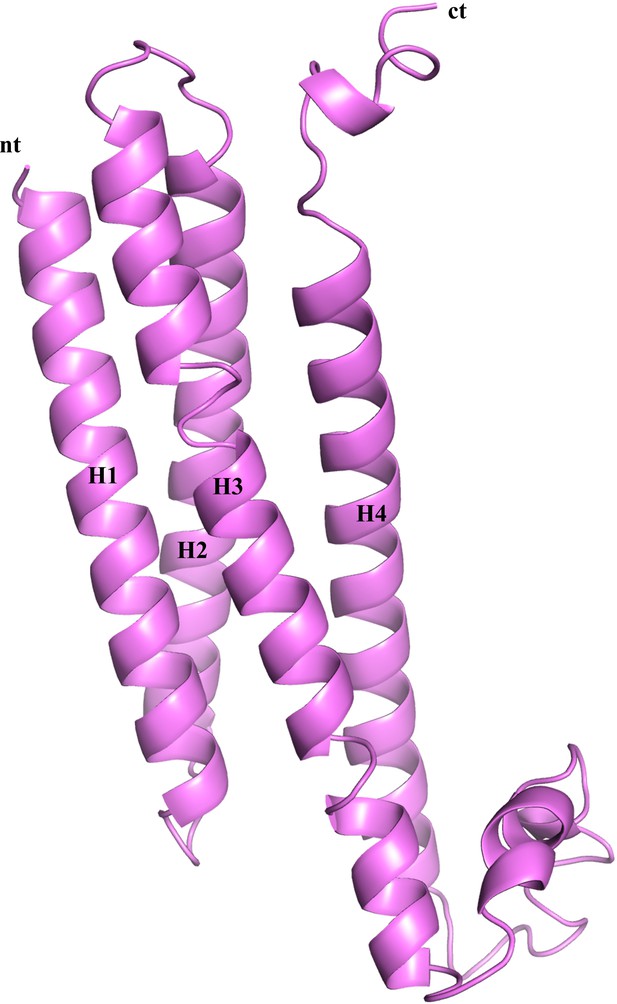
Homology model of R7BP.
Cartoon representation of the model of mouse R7BP (residues 47–231) is shown. The four α-helices are labeled as H1-H4 along with N- and C-terminal regions. The model was generated using the I-TASSER program based on the homology of R7BP with SNARE proteins (PDB entries 1fio, 2xhe, 1hs7).
Tables
X-ray data reduction statistics for the RGS7-Gβ5 dimer.
Isotropic scaling | |
---|---|
Space group | P 21 |
Unit cell dimensions | |
a, b, c, β | 68.32 Å, 162.76 Å, 95.29 Å, 98.36° |
Resolution (last shell) | 94.28 Å - 2.81 Å (2.86 Å - 2.81 Å) |
Total measurements | 191,664 |
No. of unique reflections (last shell) | 50,669 (2,501) |
Wavelength | 1.0 Å |
Rp.i.m.* (last shell) | 0.036 (0.431) |
I/σ(I) (last shell) | 15.1 (2.3) |
Completeness (last shell) | 0.997 (1.0) |
Multiplicity (last shell) | 3.8 (3.9) |
CC1/2 † (last shell) | 0.999 (0.868) |
Anisotropic scaling | |
Resolution (last shell) | 4.28 Å - 2.13 Å (2.48 Å - 2.13 Å) |
Total measurements | 20,4124 |
No. of unique reflections (last shell) | 54,345 (2,718) |
Wavelength | 1.0 Å |
Rp.i.m.* (last shell) | 0.038 (0.5) |
I/σ(I) (last shell) | 14.6 (2.1) |
Completeness (spherical) (last shell) | 0.47 (0.064) |
Completeness (ellipsoidal) (last shell) | 0.92 (0.63) |
Multiplicity (last shell) | 3.8 (3.7) |
CC1/2 † (last shell) | 0.999 (0.546) |
-
*Precision-indicating merging R factor (Weiss and Hilgenfeld, 1997; Weiss, 2001).
†Pearson correlation coefficient calculated between the average intensities of each random half of measurements of unique reflections (Karplus and Diederichs, 2012).
Crystallographic refinement statistics for the RGS7-Gβ5 dimer.
Resolution Å (last shell) | 37.54 Å - 2.13 Å (2.16 Å - 2.13 Å) |
---|---|
No. of reflections (working set) | 54,330 |
No. of reflections (test set) | 2610 |
R-factor (last shell) | 0.174 (0.296) |
R-free (last shell) | 0.216 (0.451) |
No. of polypeptide chains | 4 |
No. of non-hydrogen atoms: | |
Protein | 12,051 |
solvent | 480 |
B-factor (Å Gutierrez, 2018) | |
Protein | 55.7 |
Solvent | 48.21 |
Root mean square deviations (r.m.s.d.) | |
Bond lengths (Å) Bond angles (0) | 0.002 0.488 |
Ramachandran plot | |
favored (%) | 96.25 |
allowed (%) | 3.75 |
outliers (%) | 0.0 |
Reagent type (species) or resource | Designation | Source or reference | Identifiers | Additional information |
---|---|---|---|---|
Genetic reagent (baculovirus) | His-RGS7 | This Paper | N/A | |
Genetic reagent (baculovirus) | Gβ5 | This Paper | N/A | |
Genetic reagent (baculovirus) | GST-PP-R7BP | This Paper | N/A | |
Cell line (insect cells) | Sf9 | ThermoFisher Scientific | 11496015 | |
Chemical compound, drug | Sypro Orange dye | Sigma-Aldrich | S5692 | |
Chemical compound, drug | Crystallization plates | TTP Labtech | 4150–0560 | |
Chemical compound, drug | Crystallization screens | Hampton Research and Qiagen | N/A | |
Chemical compound, drug | Polyethylene glycol 3350 | Sigma | 88276–250G | |
Chemical compound, drug | TCEP-HCl | Hampton Research | HR2-801 | |
Software, algorithm | MASCOT | Matrix Science | N/A | |
Software, algorithm | HDX Workbench | Pascal et al., 2012 | PMID: 22692830 | |
Software, algorithm | autoPROC | Vonrhein et al., 2011 | https://www.globalphasing.com/autoproc/ | |
Software, algorithm | PHASER | McCoy et al., 2007 | http://www.phaser.cimr.cam.ac.uk/index.php/ | |
Software, algorithm | PHENIX | Adams et al., 2010 | https://www.phenix-online.org | |
Software, algorithm | BUSTER | Bricogne, 2011 | https://www.globalphasing.com/buster/ | |
Software, algorithm | Coot | Emsley and Cowtan, 2004 | http://www2.mrc-lmb.cam.ac.uk/personal/pemsley/coot/ | |
Software, algorithm | MolProbity | Chen et al., 2010 | http://molprobity.biochem.duke.edu | |
Software, algorithm | PyMOL | Schršdinger, LLC | https://pymol.org/2/ | |
Software, algorithm | I-TASSER | Yang and Zhang, 2015 | https://zhanglab.ccmb.med.umich.edu/I-TASSER/ | |
Software, algorithm | PISA | N/A | http://www.ebi.ac.uk/msd-srv/prot_int/cgi-bin/piserver | |
Software, algorithm | Modeller | Eswar et al., 2006 | PMID: 18428767 | |
Software, algorithm | AMBER 16 | AMBER software, University of California, San Francisco | http://ambermd.org/ | |
Software, algorithm | H ++ server | Gordon et al., 2005 | http://biophysics.cs.vt.edu/H++ | |
Software, algorithm | UCSF Chimera | Pettersen et al., 2004 | http://www.rbvi.ucsf.edu/chimera/ | |
Software, algorithm | cpptraj | Roe and Cheatham, 2013 | PMID: 26583988 | |
Software, algorithm | VMD | Humphrey et al., 1996 | https://www.ks.uiuc.edu/Research/vmd/ | |
Software, algorithm | Carma | Glykos, 2006 | https://utopia.duth.gr/glykos/Carma.html | |
Software, algorithm | CatDCD | Written by Justin Gullingsrud | http://www.ks.uiuc.edu/Development/MDTools/catdcd | |
Software, algorithm | gncommunities | By Luthey-Schulten group | http://faculty.scs.illinois.edu/schulten/software/networkTools/index.html |