Upper bound on the biological effects of 50/60 Hz magnetic fields mediated by radical pairs
Figures
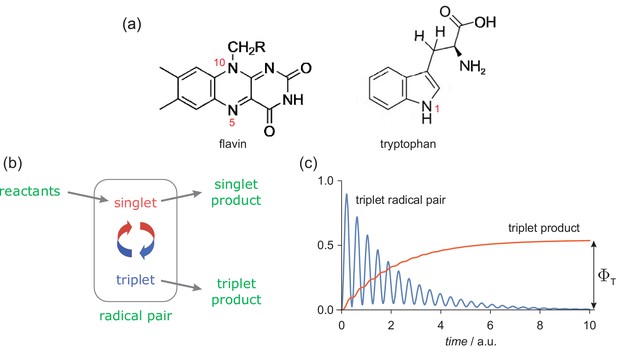
The radical pair mechanism.
(a) Structures and atom numbers schemes for the FAD and TrpH molecules from which magnetically sensitive radical pairs are formed in purified cryptochromes. (b) Simple reaction scheme for a singlet-born radical pair able to react spin-selectively to form singlet and triplet reaction products. The red/blue arrows represent the coherent interconversion of the two forms of the radical pair. The reaction scheme is a simplified version of the cryptochrome photocycle (Hore and Mouritsen, 2016; Maeda et al., 2012). (c) Schematic dependence of the amounts of triplet radical pair and triplet product present as a function of time (in arbitrary units) after the formation of the radical pair in a singlet state. The triplet yield, , is the amount of triplet product formed once all radical pairs have reacted.
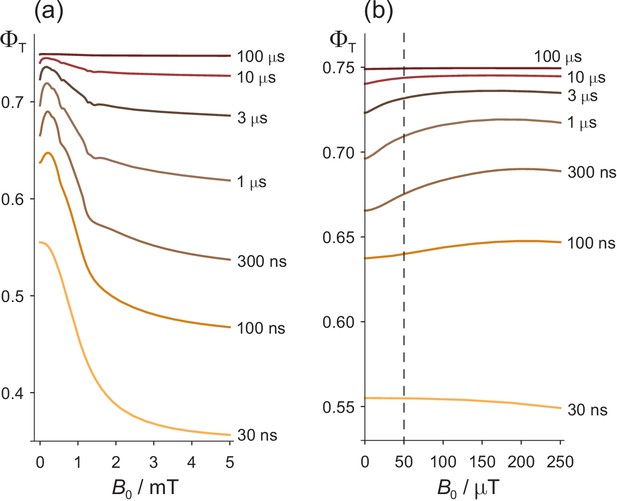
Effects of a static magnetic field on a model of the [FAD•− TrpH•+] radical pair in cryptochrome.
(a) Dependence of the reaction yield on the static magnetic field strength, , for different values of the radical pair lifetime, . The spin relaxation time is 1 μs. (b) An expanded view of the low field region of (a) with = 50 μT indicated by the dashed line. The difference between at zero field and at 50 μT is largest when ≈ 1 μs. The corresponding calculations for a spin relaxation time of 0.1 μs are shown in Figure 2—figure supplement 1.
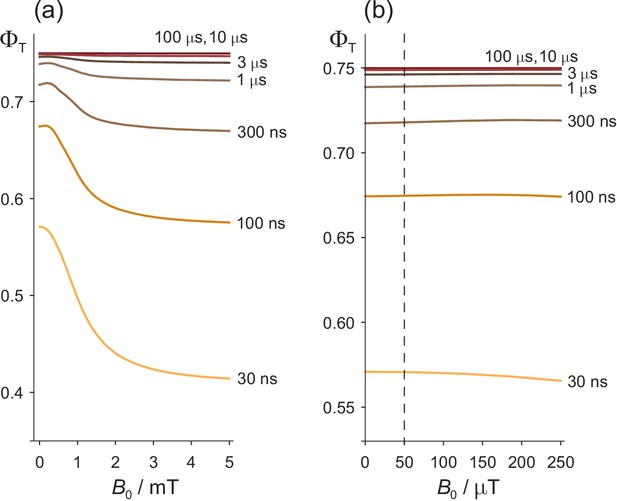
for = 107 s−1.
https://doi.org/10.7554/eLife.44179.004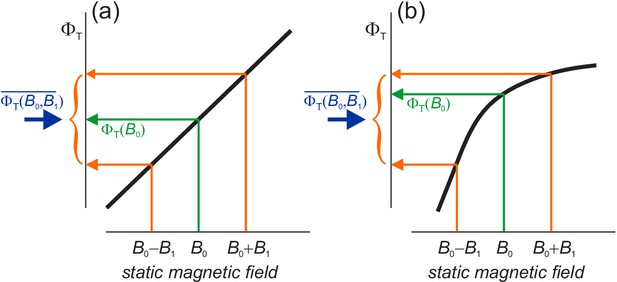
Schematic representations (thick black lines) of the dependence of the reaction yield, , on the strength of a static magnetic field.
The orange arrows indicate the yields for the maximum and minimum values of in Equation (5). The green arrows show the yields when . The blue arrows mark the free radical yields averaged over the phase of the ELF field, Equation (6) . (a) When is linear in , the effect of the GMF and the ELF field together equals that of the GMF alone. (b) When is non-linear, the effects of GMF plus ELF and GMF-alone differ. in (b) has been drawn with a negative curvature (concave downward), with the result that and (Equation (7) and (10)). A positive curvature (concave upward) would give the opposite signs.
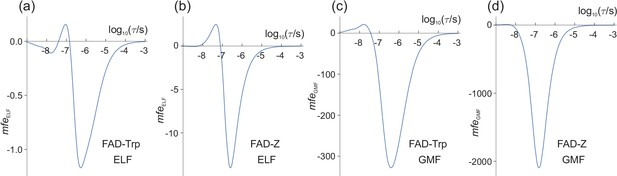
Magnetic field effects (in ppm) on model radical pairs as a function of the lifetime of the radical pair, τ, in the range 1 ns−1 ms.
The spin relaxation time is 1 μs. (a) and (c) A model of the [FAD•− TrpH•+] radical pair in cryptochrome, containing two 14N nuclei in the FAD•− radical and one in the TrpH•+ radical. (b) and (d) A model of the [FAD•− Z•] radical pair in cryptochrome in which the Z• radical has no hyperfine interactions. (a) and (b) (Equation (10)) for = 50 μT, = 1.0 μT. (c) and (d) (Equation (12)) for = 50 μT, = −1.0 μT. Note the different vertical scales of the four panels. The validity of the Taylor series expansion (Equation (9)) was confirmed by numerical integration over α, the phase of the ELF field (Figure 4—figure supplement 1). Corresponding calculations for a spin relaxation time of 0.1 μs are shown in Figure 4—figure supplement 2. Corresponding versions of (a) and (c) when 25 μT ≤ ≤ 65 μT are shown in Figure 4—figure supplement 3.
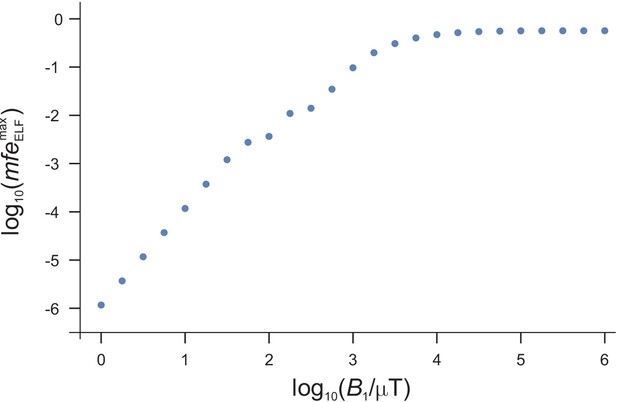
evaluated by numerical integration.
https://doi.org/10.7554/eLife.44179.007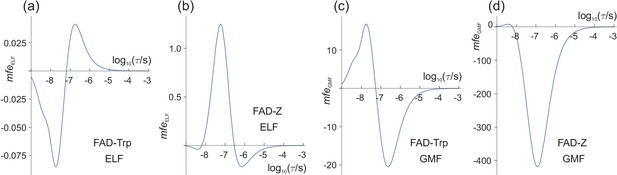
and for = 107s−1.
https://doi.org/10.7554/eLife.44179.008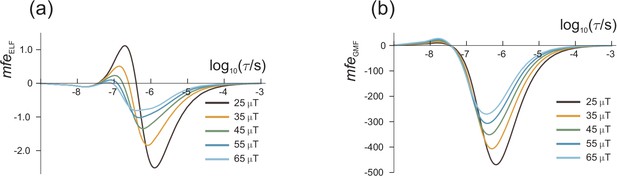
and for 25 μT ≤ ≤ 65 μT.
https://doi.org/10.7554/eLife.44179.009Tables
Changes in reaction product yields (in ppm) arising from small changes in magnetic field strengths and temperature
https://doi.org/10.7554/eLife.44179.010[FAD•− TrpH•+] | [FAD•−Z•] | ||
---|---|---|---|
* | =1.0 μT | −1.2 | −14 |
* | = −1.0 μT | −330 | −2100 |
† | 280 | 150 | |
‡ | −36 | −54 | |
§ | +21 | +27 | |
¶ | −15 | −27 |
-
* = 106 s−1, = 50 μT
†
-
‡ = = 106 s−1, = 103 s−1, = 0, = 50 μT
§ = = 106 s−1, = 0, = 103 s−1, = 50 μT
-
¶ = = 106 s−1, = = 103 s−1, = 50 μT
[FAD•− TrpH•+] | [FAD•− Z•] | [FAD•− TrpH•+] | [FAD•− Z•] | |
r / s−1 | ||||
1 μs | −1.2 | −14 | −330 | −2100 |
10 μs | −3.2 | −18 | −550 | −2500 |
Additional files
-
Source code 1
- https://doi.org/10.7554/eLife.44179.011
-
Transparent reporting form
- https://doi.org/10.7554/eLife.44179.012