Mechanism of completion of peptidyltransferase centre assembly in eukaryotes
Figures
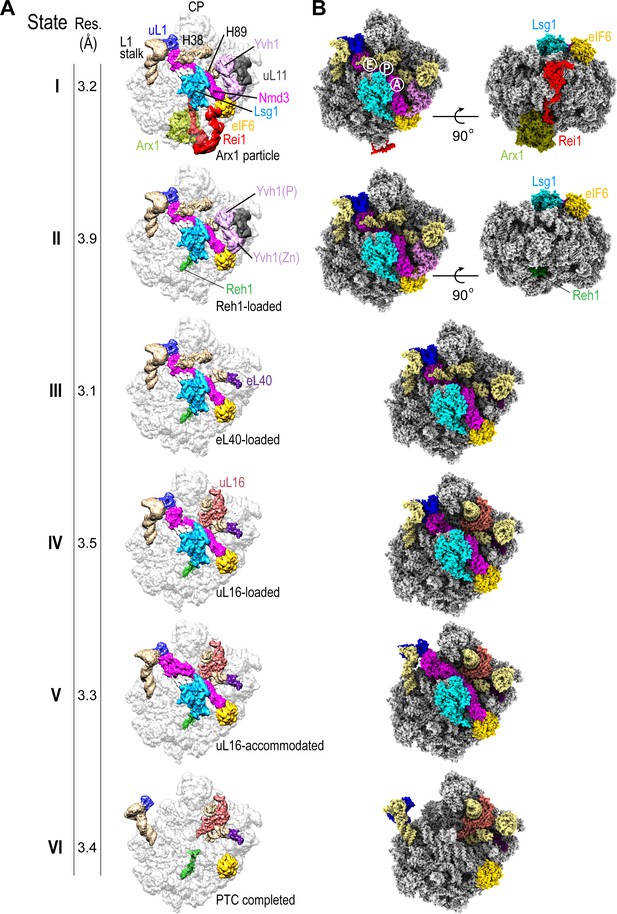
Sequential steps in late cytoplasmic 60S subunit maturation.
(A) Cryo-EM reconstructions of six cytoplasmic maturation states (I–VI). States, overall resolution, changes in protein composition and rRNA conformation are indicated. The phosphatase (P) and zinc finger (Zn) domains of Yvh1 are indicated in state II. (B) Atomic models of pre-60S states I-VI with rRNA and biogenesis factors highlighted.
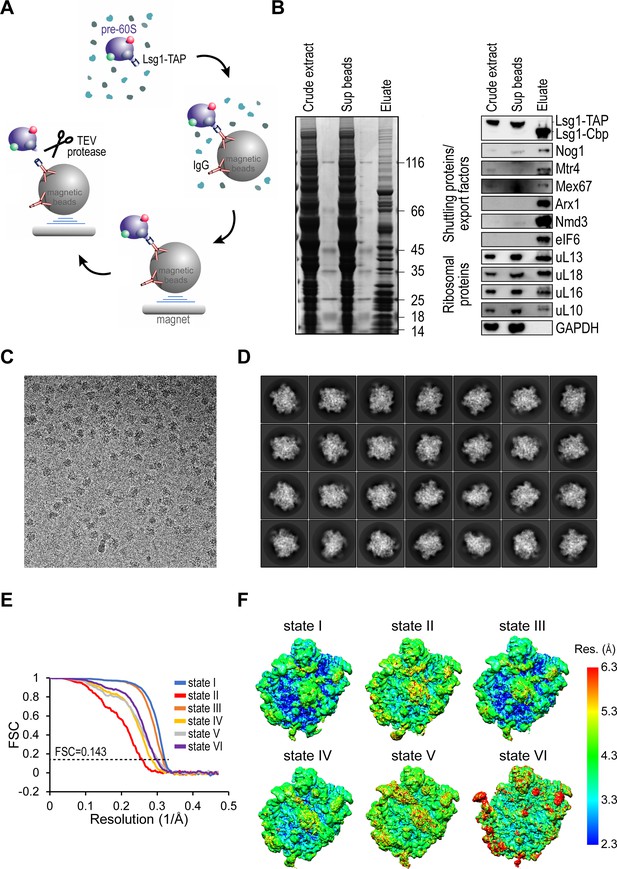
Sample purification, cryo-EM image analysis and local resolution of pre-60S particles.
(A) Schematic of pre-60S Lsg1-TAP affinity purification protocol. (B) Analysis of crude extract and supernatant by Coomassie-stained SDS-PAGE (left) and immunoblotting (right) with the indicated antibodies. (C) Representative micrograph from the Lsg1-TAP dataset. (D) Representative reference-free class averages of the Lsg1-TAP particles used for 3D classification. (E) Gold-standard Fourier shell correlation (FSC) curves after 3D refinement in RELION (Bai et al., 2013; Scheres, 2012b). (F) Surface views of unfiltered states I-VI coloured according to local resolution calculated in ResMap (Kucukelbir et al., 2014).
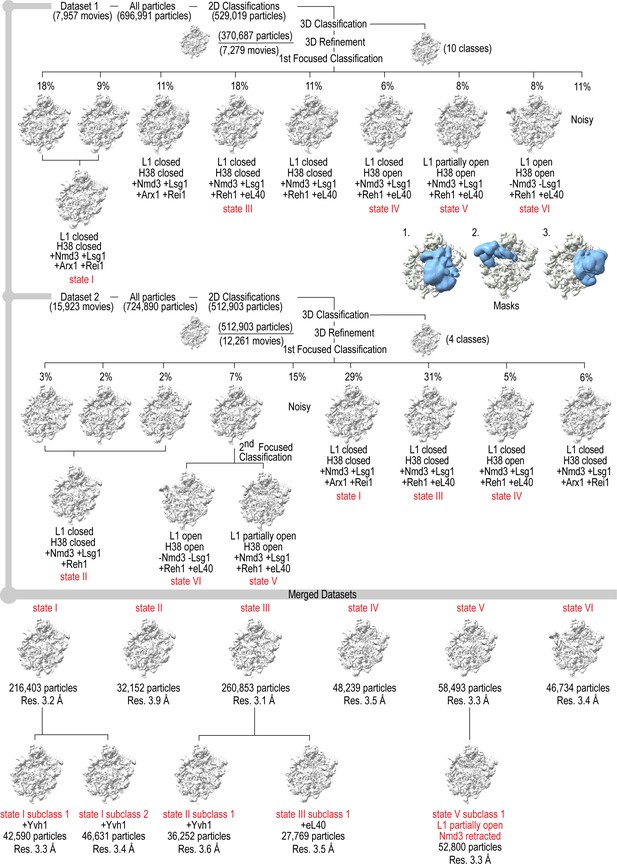
Cryo-EM data processing scheme.
Maximum likelihood classification scheme and masks used to obtain native pre-60S maturation states I-VI. The two datasets were processed independently and identical classes from each dataset merged to yield six different states with five subclasses, highlighted in red at the bottom. Insets show the positions of the three masks (blue) on the 60S subunit (grey).
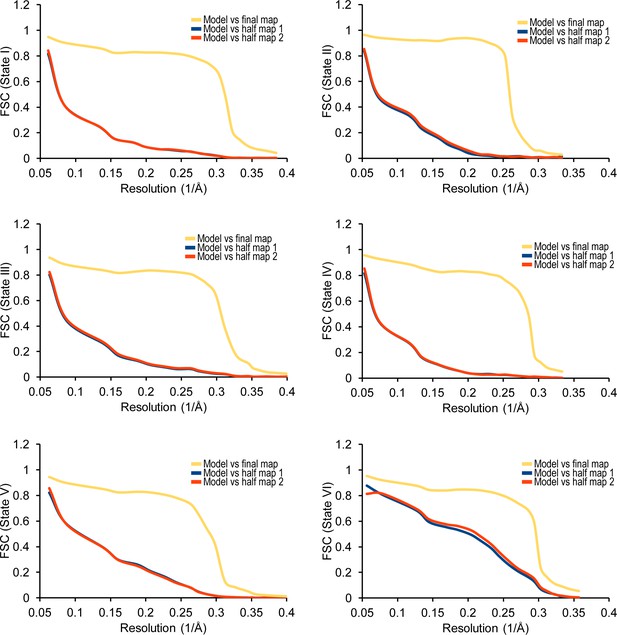
Cross-validation against overfitting.
FSC curves are shown between the final refined atomic model of the states I-IV complexes and the reconstructions from all particles (orange); between the model refined in the reconstruction from only half of the particles and the reconstruction from that same half (red); and between that same model and the reconstruction from the other half of the particles (blue) calculated by REFMAC v5.8 (Amunts et al., 2014).
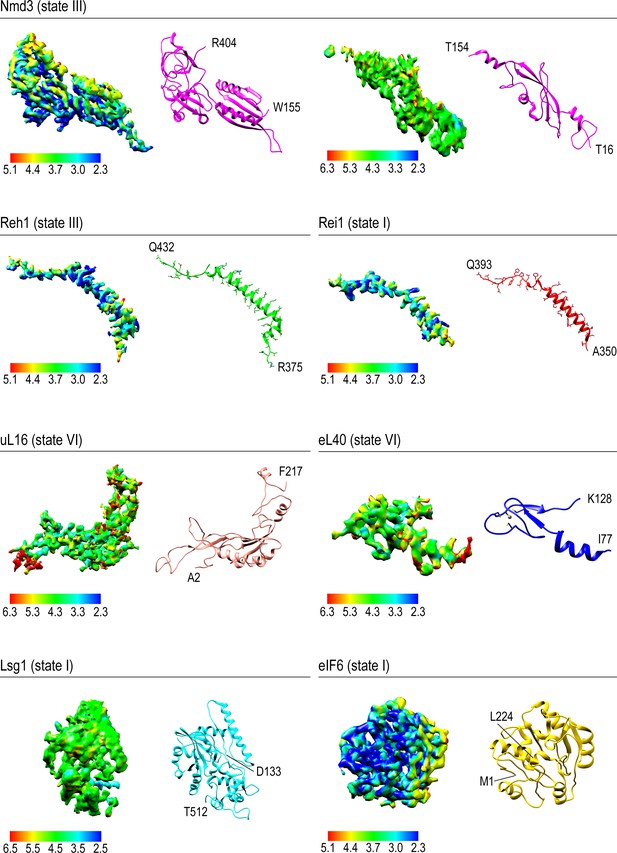
Local resolution for ribosome assembly factors, eL40 and uL16.
Surface views of factors extracted from the indicated states are coloured by local resolution calculated in ResMap (Kucukelbir et al., 2014) and accompanied by atomic models. The Nmd3 N-terminus and Lsg1 are low-pass filtered, other factors unfiltered.
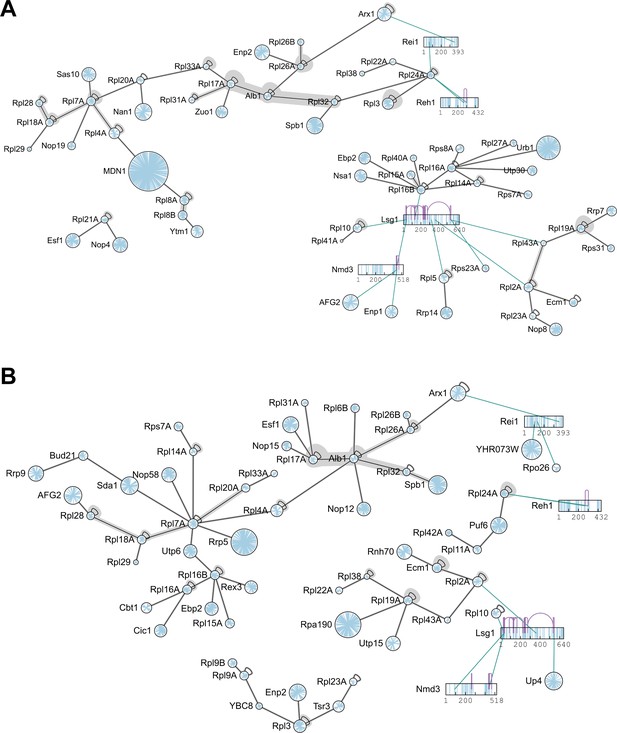
Protein-protein interaction network of pre-ribosomal particles purified by Lsg1-TAP.
Biological replicates I (A) and II (B) of cross-linked pre-ribosomal particles immunopurified using Lsg1-TAP are shown. Proteins are represented as circles. Lysine residues are blue, crosslinks are black or grey (if multiple linkage sites were identified). Crosslinks are depicted at the residue level for Lsg1, Nmd3, Reh1 and Rei1. Inter-protein crosslinks are green; intra-protein crosslinks are purple.
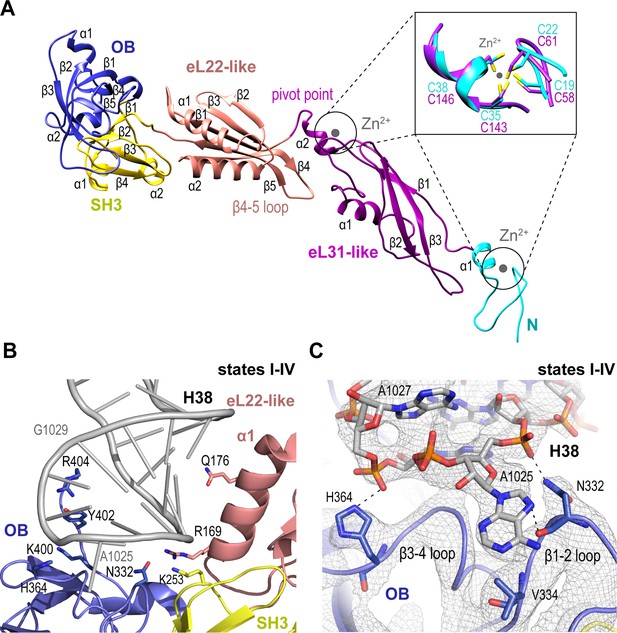
Nmd3 maintains the closed conformation of helix 38.
(A) The modular atomic structure of Nmd3 with the individual domains (OB, SH3, eL22-like, eL31-like and the two treble clef zinc fingers) indicated by colour coding. Inset shows the superposition of the two treble clef zinc fingers (RMSD ~1 Å). (B) Interaction of the Nmd3 OB, SH3 and eL22-like domains with the tip of H38. (C) Nmd3 stabilises an A1025 base-flip. Atomic model, derived from the state III map (grey mesh), shows the interaction between Nmd3 and the flipped-out base A1025.
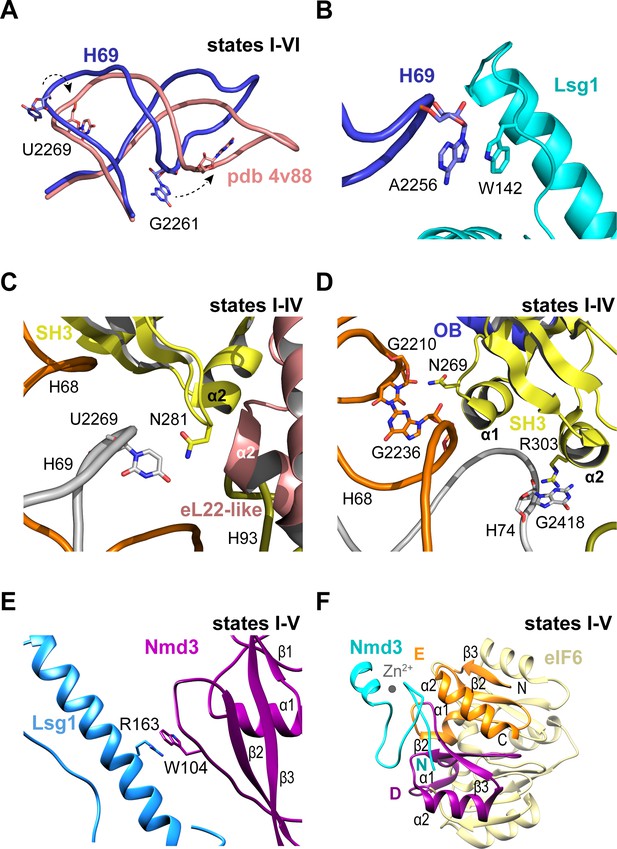
Nmd3 interacts with Lsg1 and eIF6 throughout 60S maturation.
(A) Conformation of H69 (states I-VI) differs from the mature 60S subunit (4v88, Ben-Shem et al., 2011). (B) Interaction of Lsg1 with the tip of H69. (C) Contact between Nmd3 residue N281 and base U2269 (H69). (D) Interactions of Nmd3 SH3 domain with H68 and H74. (E) Interaction between Lsg1 and the Nmd3 eL31-like domain. (F) Interaction of the Nmd3 N-terminal domain with the D and E domains of eIF6.
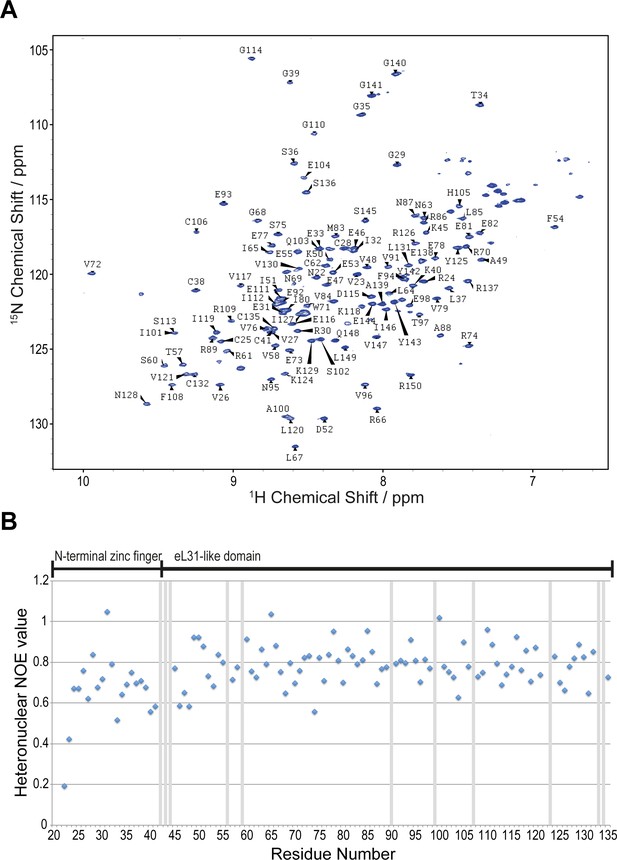
NMR spectroscopic analysis of A.fulgidus Nmd3.
(A) Assigned 1H-15N BEST-TROSY spectrum of residues 22–150 in solution. (B) Heteronuclear 1H{15N} NOE analysis of A. fulgidus Nmd3. Values close to one indicate rigid secondary structure and values < 1 have individual flexibility on the picosecond timescale. Exchange broadened residues are marked with grey bars.
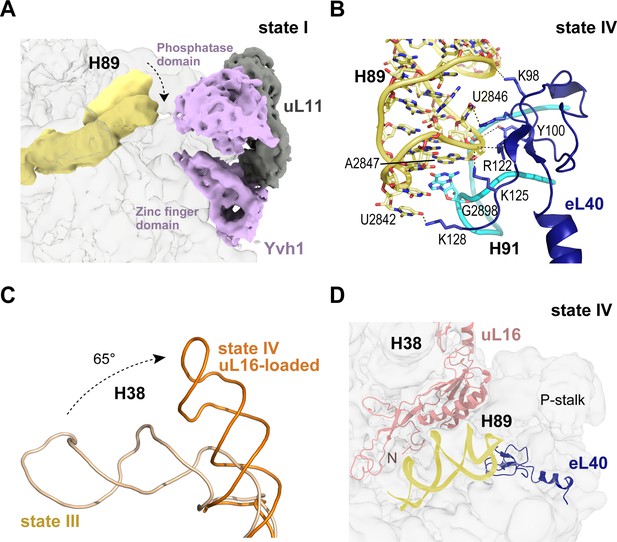
Binding of eL40 stabilises H89 to form the uL16 binding site.
(A) H89 is flexible in states I-II. Cryo-EM density map of Yvh1 is shown in purple; uL11 in grey. The mature conformation of H89 is shown in ribbon representation for comparison. (B) Atomic model of the interaction between eL40 and the tip of H89 and H91 (state IV). (C) Loading of uL16 induces a rotation of H38. The conformations of H38 in state III (wheat) compared with state IV (orange). (D) Conformation of H89 with eL40 and uL16 bound (state IV).
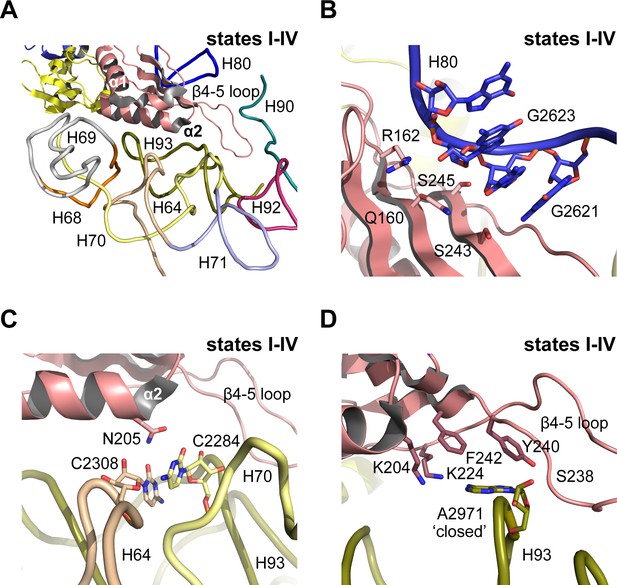
Nmd3 masks base A2971 in the peptidyltransferase centre.
(A) Interactions of Nmd3 with the PTC. (B) Interactions of the Nmd3 eL22-like domain β-sheet with H80. (C) Nmd3 residue N205 (helix α2) stabilises the junction between bases C2308 (H64) and C2284 (H70). (D) The β4–5 loop of the Nmd3 eL22-like domain maintains base A2971 (H93) in the ‘closed’ conformation.
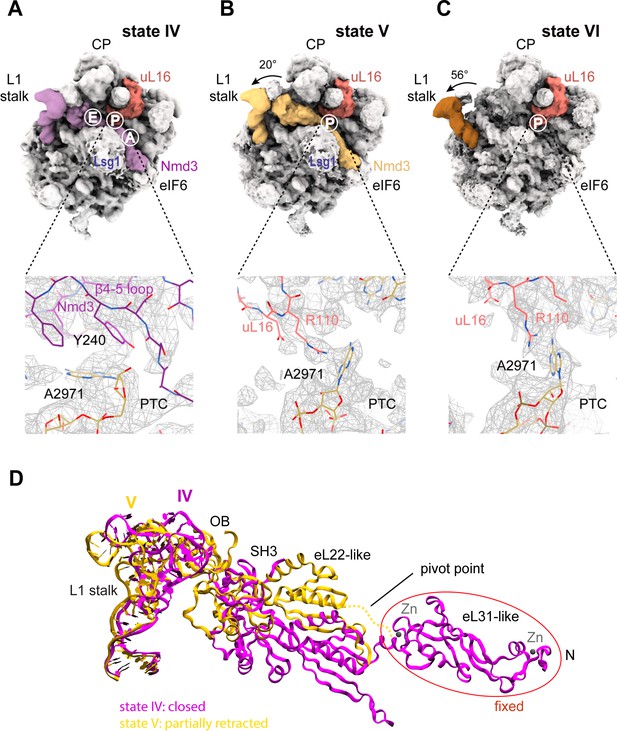
Mechanism of Nmd3 release.
(A) The Nmd3 β4–5 loop masks base A2971 (state IV). Insert shows base A2971 in the ‘closed’ position. H38 is ‘open’, the L1 stalk is ‘closed’ and uL16 is loaded. The A, P and E tRNA binding sites are indicated. (B) The uL16 P-site loop competes with the Nmd3 β4–5 loop for binding to base A2971 (state V). The L1 stalk is partially retracted (rotated by 20° relative to the fully closed L1 stalk); the Nmd3 β4–5 loop is displaced from the PTC. The uL16 P-site loop is bound to base A2971 in the flipped-out conformation. (C) The completed PTC (state VI). The L1 stalk is fully open (rotated by 56°), Nmd3 and Lsg1 have dissociated and the uL16 P-site loop is bound to base A2971 in the flipped-out conformation. (D) L1 stalk retraction promotes a conformational switch in the Nmd3 eL22-like domain. Atomic models for the L1 stalk and Nmd3 in states IV (magenta) and V (gold) are superimposed. The rotational pivot point between the eL31- and eL22-like domains is indicated.
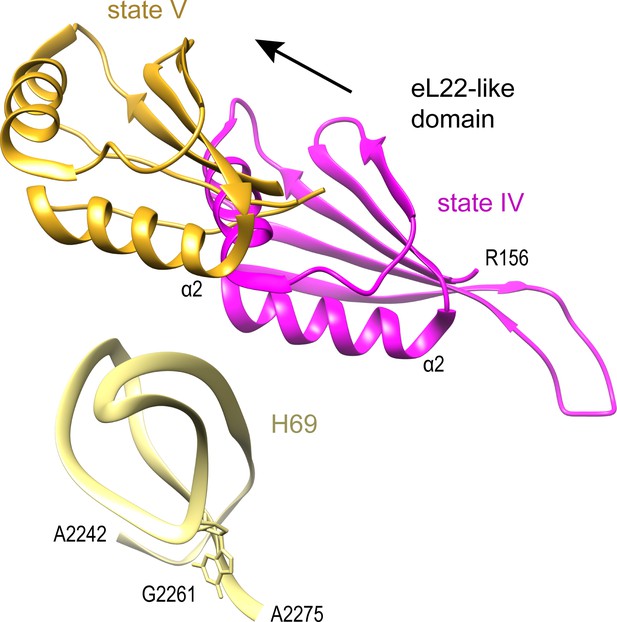
Release of Nmd3 eL22-like domain involves new contacts with H69.
Nmd3 eL22-like domain is shown in magenta (state IV) and gold (state V), H69 is yellow.
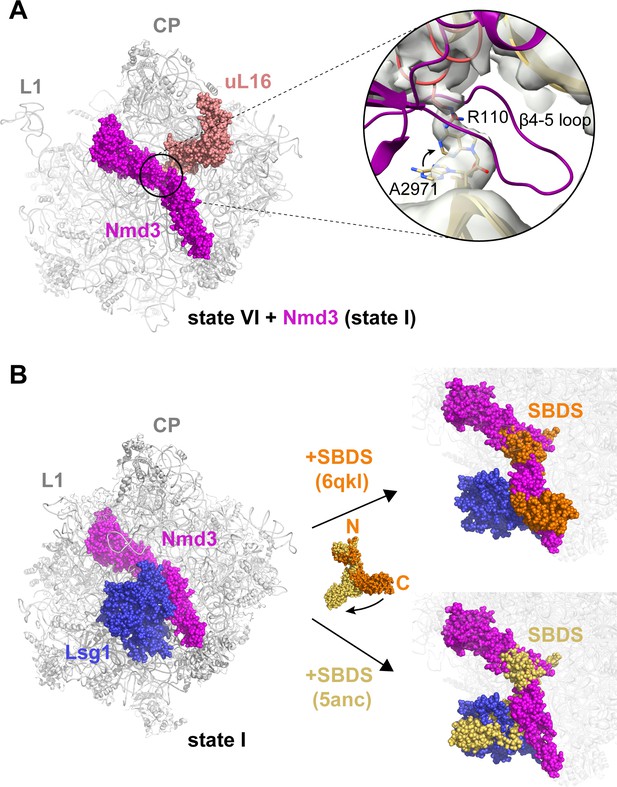
Structural conflicts during the completion of PTC assembly.
(A) Structural conflict between the Nmd3 β4–5 loop and the uL16 P-site loop. Inset shows the Nmd3 β4–5 loop (magenta) from state IV superimposed with the uL16 P-site loop (dark salmon) from state V bound to base A2971 in the flipped-out conformation. Arrow indicates the change in conformation of base A2971 between states IV-V. (B) Lsg1 and Nmd3 are placeholders for SBDS. SBDS (pdb 6qkl, 5anc) is superimposed with the cryo-EM density map of state I. Cryo-EM density for Lsg1 is blue, Nmd3 is magenta, SBDS (pdb 6qkl) is brown, SBDS (pdb 5anc) is gold.
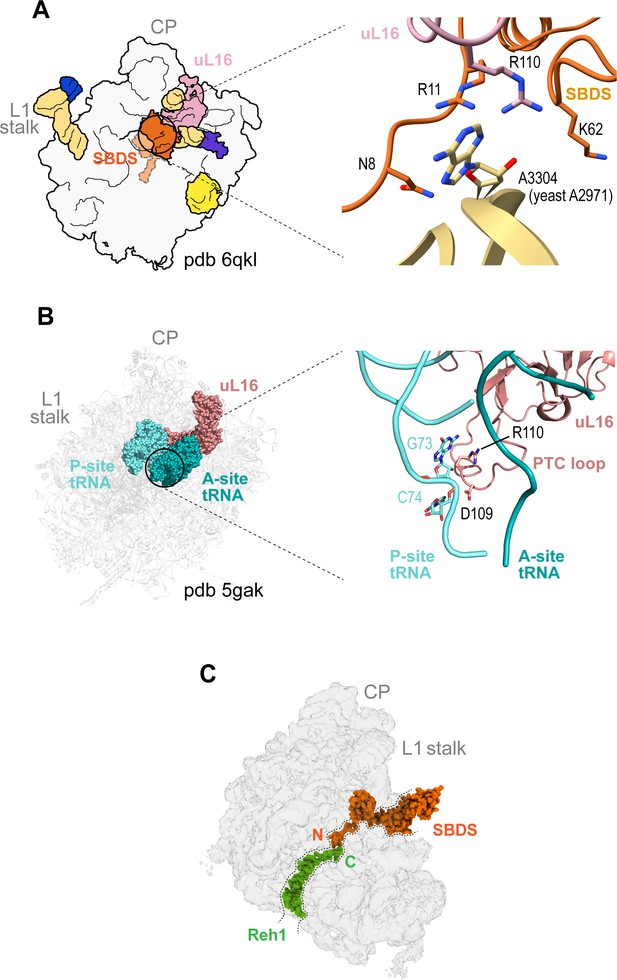
Quality control assessment of PTC assembly by SBDS.
(A) Interactions between uL16 (dark salmon), SBDS (light orange) and Dictyostelium base A3304 (equivalent to yeast A2971) (gold). (B) Interactions of uL16 with the A site (dark green) and P site (cyan) tRNAs (pdb 5gak). (C) Potential cross-talk between SBDS and Reh1 in the peptide exit tunnel. The cryo-EM density for SBDS (orange, pdb code 6qkl) is superimposed with the cryo-EM density map of state VI. Reh1 density is green.
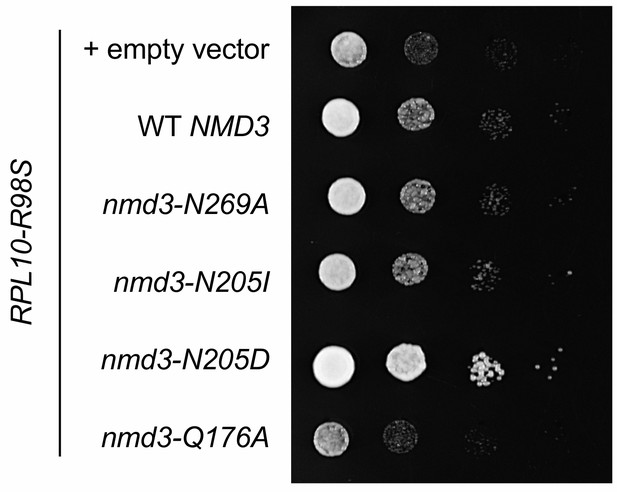
Suppression of the T-ALL associated uL16-R98S variant by mutations in NMD3.
Complementation of GAL10::RPL10 rpl10-R98S cells with nmd3 variant alleles. Ten-fold serial dilutions (from left to right) of the indicated strains are shown.
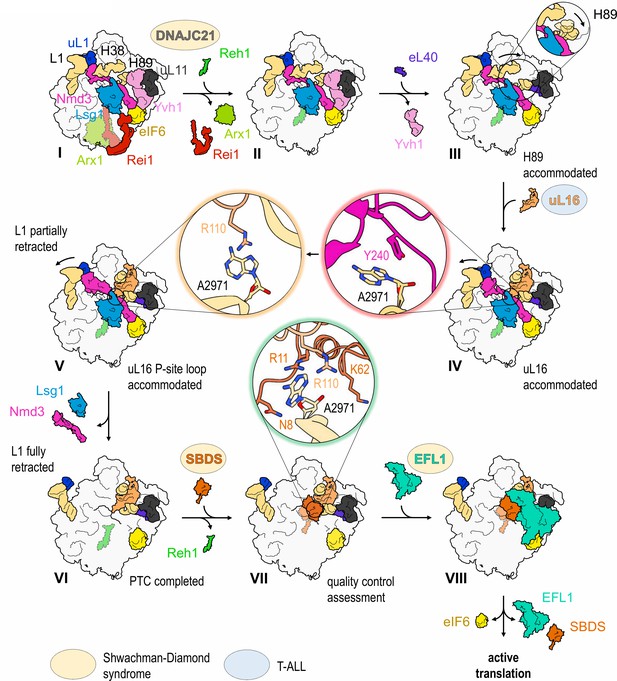
Mechanism of completion of PTC assembly and its quality control.
Insets show the sequential interactions of base A2971 with Nmd3, uL16 and SBDS during PTC completion. Models for 60S-bound EFL1 and SBDS are based on EMD-3145, EMD-3146 and EMD-3147 (Weis et al., 2015). Proteins targeted by mutations in Shwachman-Diamond syndrome (yellow) and paediatric T-ALL (blue) are highlighted.
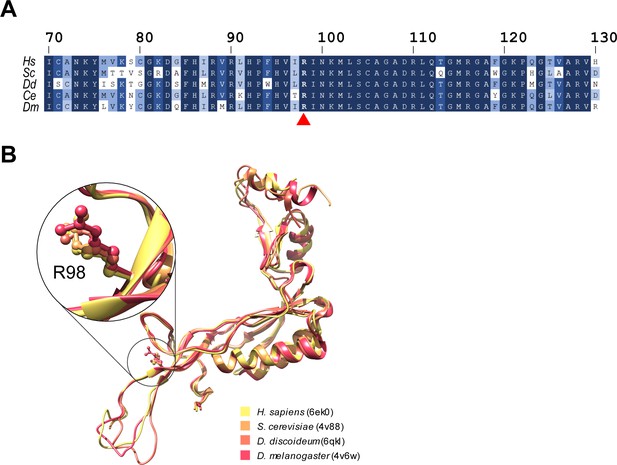
The uL16 sequence and structure are highly conserved.
(A) Protein sequence alignment of uL16 from Homo sapiens, Saccharomyces cerevisiae, Dictyostelium discoideum, Caenorhabditis elegans and Drosophila melanogaster. Residue R98 (mutated in T-ALL) is highlighted in bold and marked with a red arrow (▲). The alignment was performed using Clustal Omega (Sievers et al., 2011). (B) Superposition of corresponding atomic models for uL16 (pdb entries 6ek0 [Natchiar et al., 2017], 4v88 [Ben-Shem et al., 2011], 6qkl [Weis et al., 2015] and 4v6w [Anger et al., 2013]).
Videos
Mechanism of completion of peptidyltransferase centre assembly.
https://doi.org/10.7554/eLife.44904.021Additional files
-
Supplementary file 1
Data collection, model refinement and validation (A), and summary of modelled ribosomal proteins, assembly factors and rRNA (B).
- https://doi.org/10.7554/eLife.44904.022
-
Supplementary file 2
Crosslinks identified by XL-MS.
Data for two independent immunoprecipitation experiments (A, B), including the exact amino acid sequence of the cross-linked peptides and the position of the cross-linked lysine residue ‘crosslinked peptide’, the name of the respective protein ‘protein1’ and ‘protein2’, nature of the cross-link ‘type’, the absolute position of the cross-linked lysine residues within the UniProt or construct sequence ‘Absolute Position 1’ and ‘Absolute Position 2’. ‘deltaS’ gives the delta score of the respective crosslink and is a measure of how close the best assigned hit was scored in regard to the second best, ‘Id-Score’, which is a weighted sum of different scores used to assess the quality of the composite MS2 spectrum as calculated by xQuest.
- https://doi.org/10.7554/eLife.44904.023
-
Supplementary file 3
Plasmids (A), primers (B), and yeast strains (C).
- https://doi.org/10.7554/eLife.44904.024
-
Transparent reporting form
- https://doi.org/10.7554/eLife.44904.025