A conserved Mcm4 motif is required for Mcm2-7 double-hexamer formation and origin DNA unwinding
Figures
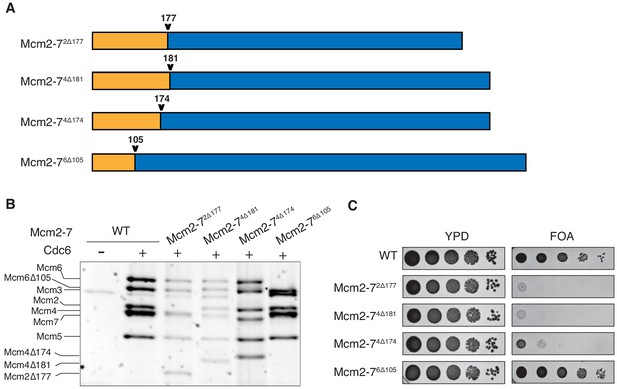
N-terminal deletions of Mcm2 and Mcm4 NTEs are defective for Mcm2-7 loading and essential for viability.
(A) Diagram of Mcm2, Mcm4, and Mcm6 deletions. (B) Mcm2-72Δ177 and Mcm2-74Δ181 are defective in helicase loading onto origin-containing DNA. Helicase loading of the indicated Mcm2-7 N-terminal-deletions was monitored by incubating with purified helicase-loading proteins and bead-attached origin DNA followed by a high-salt wash (0.5M NaCl). All loading was dependent on Cdc6. (C) Mcm2Δ177 and Mcm4Δ181 deletions are lethal. The indicated genes were tested for complementation of a deletion of the corresponding wild-type gene before (YPD) and after (FOA) selecting against a plasmid with the corresponding wild-type gene. Endogenous MCM2, MCM4 or MCM6 gene is deleted, and a copy of the corresponding wild-type MCM is present on a URA3-containing plasmid. Indicated MCM mutants were integrated into the genome at the corresponding TRP1 locus. Five-fold serial dilutions of cells were spotted on the indicated media.
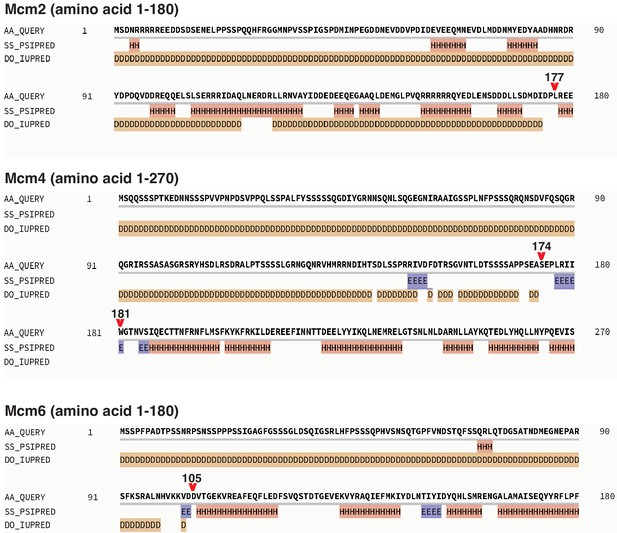
Secondary structure prediction of Mcm2, 4, and 6 NTE by Quick2D, using the indicated analysis tools (PSIPRED, Protein secondary structure prediction based on position-specific scoring matrices as in Jones, 1999; IUPRED, the pairwise energy content estimated from amino acid composition discriminates between folded and intrinsically unstructured proteins as in Dosztányi et al., 2005; SS, alpha-helix/beta-strand; DO, Disorder).
The resulting secondary structure predictions are labeled as following; H, alpha-helix; E, beta-strand; D, Disorder. Red arrows indicate the last amino acid of the NTE deletion regions.
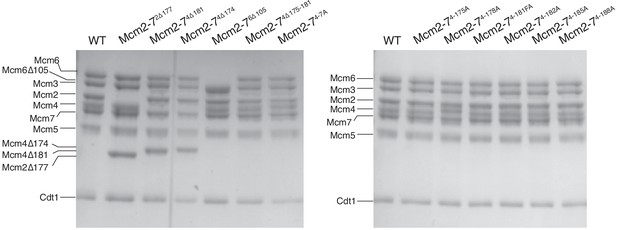
Mcm2-7 complexes containing all wild-type or one mutant subunit (indicated) assembled into stable Mcm2-7 heterohexamers that bound to Cdt1.
After protein purification and gel filtration column (see Materials and Methods), Mcm2-7 complexes were separated on 8% acrylamide gel and stained with Coomassie Blue.
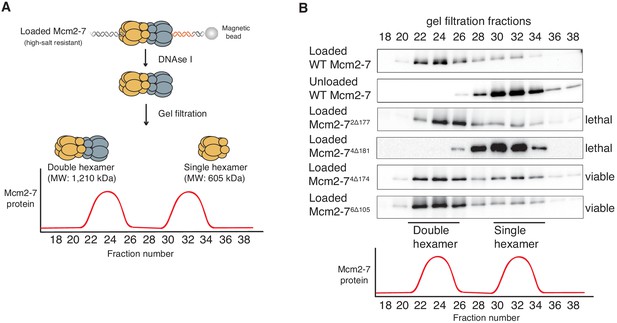
Deletion of the Mcm4 N-terminal region inhibits Mcm2-7 double-hexamer formation.
(A) Double-hexamer assay scheme. After performing a helicase-loading reaction with purified proteins and high-salt wash, loaded Mcm2-7 complexes were released from the bead-bound DNA by DNase I treatment. Released Mcm2–7 complexes were fractionated by gel filtration to separate double from single hexamers. (B) Mcm2-74Δ181 is defective for double-hexamer formation. Mcm2-7 complexes including the indicated N-terminal deletions were tested for their ability to form double hexamers as described in (A). The Flag-Mcm3 subunit of the Mcm2-7 complexes in the indicated fractions was detected by immunoblot. Wild-type Mcm2-7 double hexamers eluted early (fraction 22–26), whereas purified Mcm2-7 eluted later (fraction 30–34). Mutant viability data (right) are from Figure 1C.
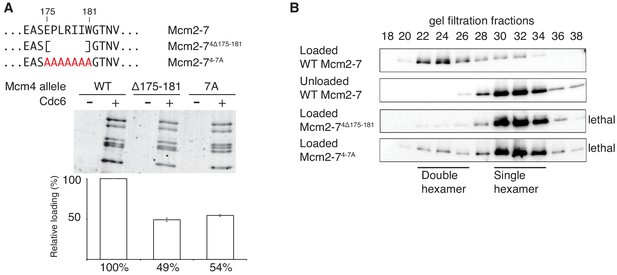
Amino acids 175–181 of Mcm4 are important for Mcm2-7 loading and double-hexamer formation.
(A) Top, diagram of Mcm4 amino acids 175–181 deletion and alanine-substitution mutations. Bottom, Mcm2-74 Δ175-181 and Mcm2-74-7A are defective for helicase loading. Helicase loading of wild-type or Mcm2-7 complexes including the indicated Mcm4 mutant was monitored as described in Figure 1. The associated graph shows the relative loading of the Mcm4 mutants compared to wild-type Mcm2–7 based on three independent experiments. Error bars indicate the SD. (B) Mcm2-74Δ175-181 and Mcm2-74-7A are defective in double-hexamer formation. Double-hexamer formation by Mcm2–7 complexes containing the indicated Mcm4 mutation was tested as described in Figure 2. Mutant viability data (right) are from Figure 3—figure supplement 1.
-
Figure 3—source data 1
Primary data and quantification for graph in Figure 3A.
- https://doi.org/10.7554/eLife.45538.008
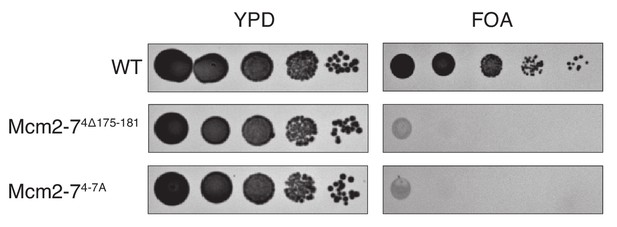
Elimination of the Mcm4 DoHM motif is lethal.
Deletion (Mcm4Δ175–181) or alanine substitution (Mcm4-7A) of Mcm4 amino acids 175–181 are lethal. In all strains, endogenous MCM4 gene is deleted, and a copy of wild-type MCM4 is present on a URA3-containing plasmid. The indicated MCM4 mutants were integrated into the TRP1 locus. Five-fold serial dilutions of cells were spotted on the indicated media.
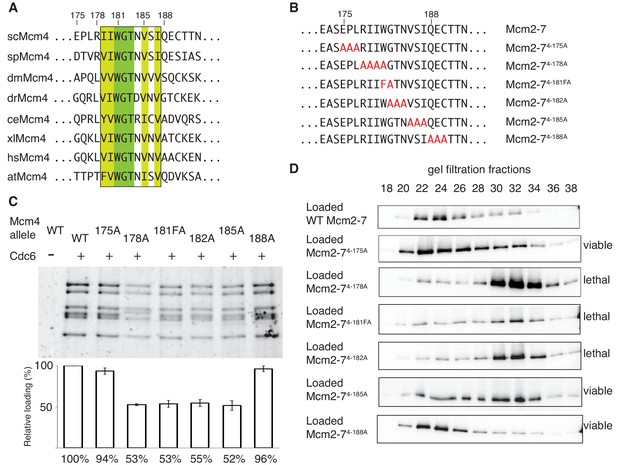
The DoHM is an essential and conserved region within Mcm4 that is required for Mcm2-7 double-hexamer formation.
(A) Protein-sequence comparison of amino acids (175 to 194) surrounding the of S. cerevisiae DoHM (boxed) with Mcm4 of the indicated species (sp, S. pombe; dm. D. melanogaster; dr, D. rerio; ce, C. elegans; xl, X. laevis; hs, H. Sapien; at, A. thaliana). Identical amino acids are shaded dark green, and conserved amino acids are shaded light green. (B) Diagram of alanine mutations spanning the DoHM and the flanking amino acids. (C) Mcm4 mutations within the DoHM show helicase-loading defects. Helicase loading of Mcm2–7 complexes containing indicated Mcm4 mutants was monitored as described in Figure 1. The associated graph shows the relative loading of the Mcm4 mutants compared to wild-type Mcm2-7, based on three independent loading experiments. Error bars indicate the SD. (D) Mcm4 mutations within the DoHM are defective for double-hexamer formation. Double-hexamer formation by Mcm2-7 complexes containing indicated Mcm4 mutant was tested as described in Figure 2. Mutant viability data are indicated to the right and are from Figure 4—figure supplement 1.
-
Figure 4—source data 1
Primary data and quantification for graph in Figure 4C.
- https://doi.org/10.7554/eLife.45538.012
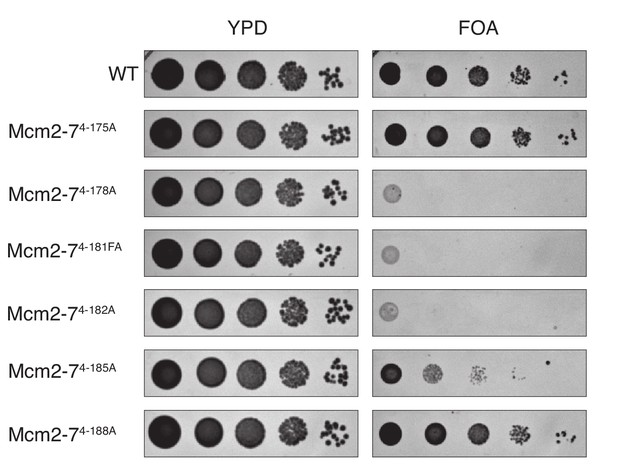
Mutation of the DoHM is lethal.
Mcm4 alanine mutations that disrupted the DoHM (Mcm2-74-178A, Mcm2-74-181FA, Mcm2-74-182A and Mcm2-74-185A) or that did not (Mcm2-74-175A and Mcm2-74-188A) were tested for complementation of a MCM4 deletion. Mcm2-74-178A, Mcm2-74-181FA, Mcm2-74-182A are fully defective for double-hexamer formation and are lethal. Mcm2-74-185A, which has partial defect in double-hexamer formation, is viable but shows a growth defect in this test. In all strains, endogenous MCM4 gene is deleted, and a copy of wild-type MCM4 is present on a URA3-containing plasmid. The indicated MCM4 mutants were integrated into the TRP1 locus. Five-fold serial dilutions of cells were spotted on the indicated media.
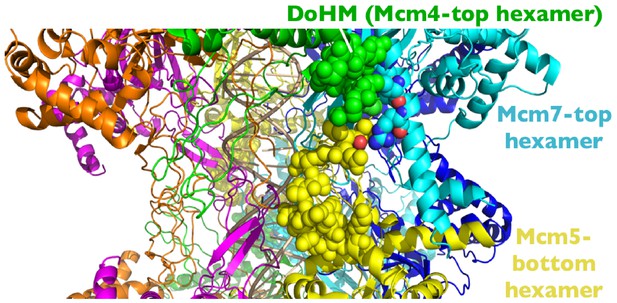
Location of the Mcm4 DoHM in the context of the Mcm2-7 double hexamer bound to DNA (Abid Ali et al., 2017).
The double-hexamer interface is located at the horizontal midpoint of the image. The DoHM of Mcm4 from the top Mcm2-7 hexamer (green), the region of Mcm7 from the top hexamer that interacts with the DoHM (light blue), and the region of Mcm5 from the bottom hexamer that interacts with the DoHM (yellow) are all shown in space filling representation. The remainder of the structure is shown as a ribbon structure with Mcm6 in orange, Mcm2 in magenta, and Mcm3 in dark blue.
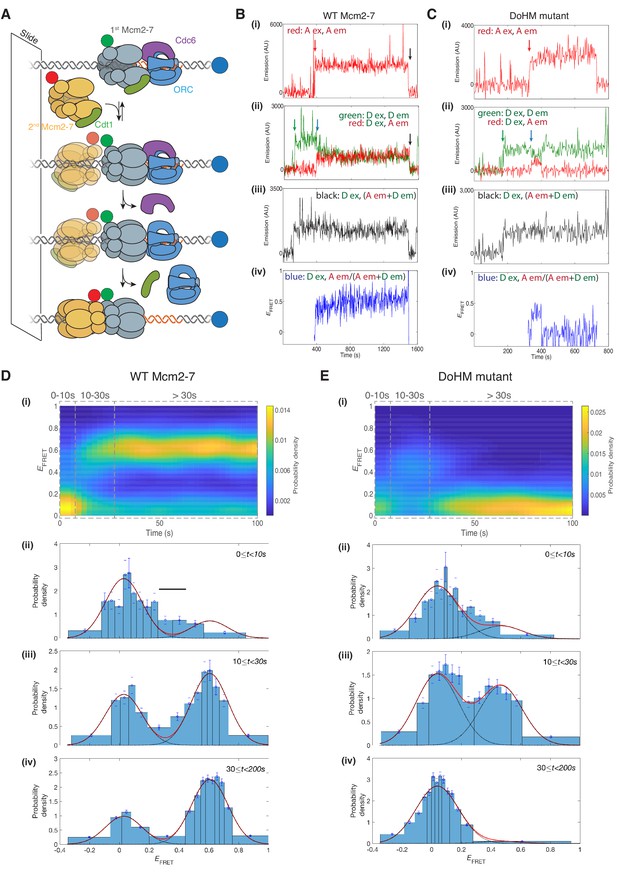
The DoHM is required for double-hexamer formation and stability.
(A) Schematic of the single-molecule helicase-loading assay. Alexa-Fluor-488-labeled (blue circle) 1.3 kb origin DNAs were coupled to a passivated microscope slide. Mcm2-7 was fluorescently labeled with donor (Dyomic-549, green circle) or acceptor (Dyomic-649, red circle) fluorophores. Purified ORC, Cdc6, and Cdt1/Mcm2–7 were incubated with slide-coupled DNA. Colocalization of fluorescently-labeled proteins with the DNA and any associated FRET signal were monitored. (B) Wild-type Mcm2-7 forms long-lasting FRET signals. Representative fluorescence records for an experiment using a 1:1 mixture of wild-type donor- and acceptor-labeled Mcm2-7 showed FRET after arrival of the second Mcm2–7. Records of fluorescence intensity for (i) acceptor excitation; acceptor emission (Dyomic-649-labeled Mcm2-7, red arrow marks arrival of acceptor-labeled Mcm2-7), (ii) donor excitation; donor emission (Dyomic-549-labeled Mcm2-7, green arrow marks arrival of donor-labeled Mcm2-7) and FRET (donor excitation; acceptor emission, blue arrow marks initiation of FRET), (iii) total emission (donor excitation; donor emission + acceptor emission), and (iv) calculated EFRET are shown. Black arrows indicate both donor and acceptor release due to the double hexamer sliding off the end of the DNA. (C) Representative fluorescence records for experiments using Mcm2-74-178A (labels and arrows as in B) show a short-lived FRET signal. (D) (i) Time evolution of the EFRET distribution for 89 wild-type Mcm2-7 complexes. Only complexes with one donor-labeled and one acceptor-labeled Mcm2-7 were selected. EFRET values were measured only after arrival of the second Mcm2-7, which was taken to be time zero in each record. The plot is a two-dimensional histogram (see Materials and Methods) with Nt = 2688 measurements within the time and EFRET range shown. (ii-iv) Histograms (probability density ± S.E.) of EFRET values recorded during the indicated time intervals after association of the second Mcm2–7 with origin DNA (black bar indicates possible intermediate EFRET state). EFRET values were globally fit to the sum (dashed cyan curves) of two Gaussians (red curves) constrained to have the same peak positions and widths at all times. (E) Analyses analogous to (D) for the DoHM mutant (114 complexes; Nt = 2584 in range shown in (i). For (ii) - (iv) in (D, E), fit parameters and numbers of observations are reported in Supplementary file 1 - table 1.
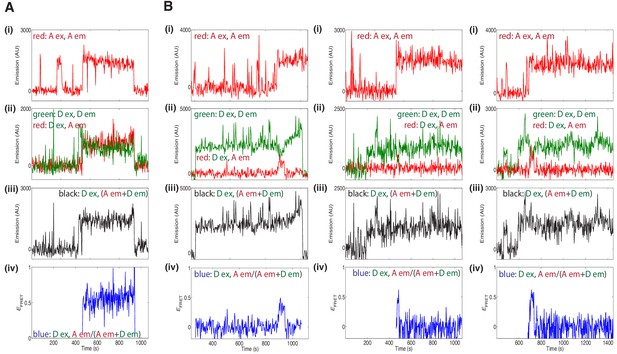
Additional fluorescence records for experiments using a 1:1 mixture of donor- and acceptor-labeled wild type Mcm2-7 (A) or the DoHM mutant (B) showed FRET after arrival of the second Mcm2–7.
Data represented in panels i-iv are as described in Figure 5B and C.
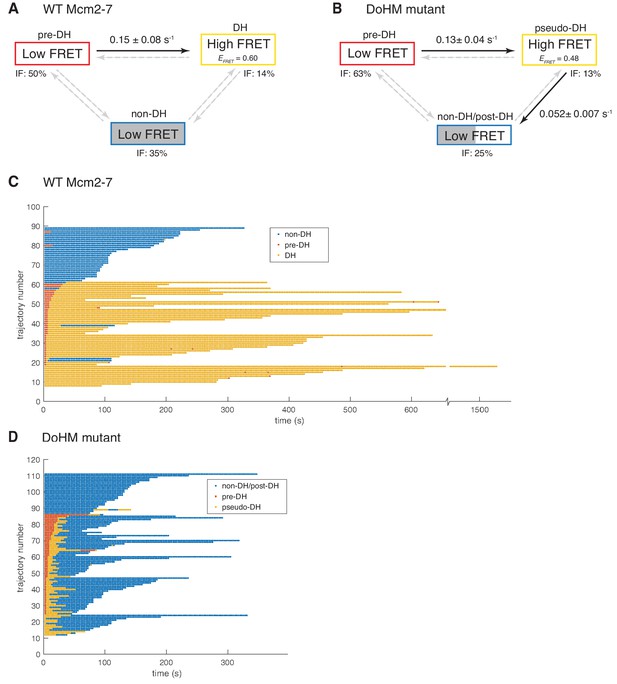
Wild-type Mcm2-7 and the DoHM mutant attempt double-hexamer formation only once.
To focus on double-hexamer formation, analysis was restricted to the time interval from second Mcm2-7 binding to the time of one or both Mcm2-7 departure. (A–B) Kinetic models of transition among the three indicated EFRET states. Pooled single-molecule fluorescence records from helicase loading experiments using (A) Mcm2-7 or (B) Mcm2-74-178A were globally fit (see Materials and methods) to generalized three-state kinetic models (i.e. all possible inter-state transitions were allowed). Black arrows indicate kinetically significant reaction steps; gray arrows indicate steps with rate constants that are not significantly greater than zero (see Figure 6—figure supplement 1). Fits also yielded the fraction (IF) of molecules in each state at the time of second hexamer binding. In the wild-type Mcm2-7 model (A), the transition from pre-DH to DH is the only process that occurs at an appreciable rate, while in the DoHM-mutant model (B) both pre-DH to pseudo-DH and pseudo-DH to post-DH steps are significant. (C–D) State rastergrams from the kinetic models. Each trajectory (horizontal line) indicates the state assigned by the model to a single-molecule record with wild-type Mcm2-7 (C) or the DOHM mutant (D), starting from the time of second hexamer binding. Pre-DH (red lines), DH or pseudo-DH (yellow lines) and post-DH or non-DH (blue lines) states are indicated. Trajectories are sorted by the onset time of the DH or pseudo-DH state, then by record length. Each record ends either due to the end of the experiment or loss of signal of one or both Mcm2-7 molecules. The blank region at the bottom of each rastergram represents low signal-to-noise records excluded from analysis (see Materials and Methods). Rare transitions (e.g. occasional transitions into or out of non-DH in wild-type Mcm2-7) which do not occur at statistically significant rates may reflect ambiguities in assigning time segments, particularly when the transitions are between two states with the same (zero) EFRET.
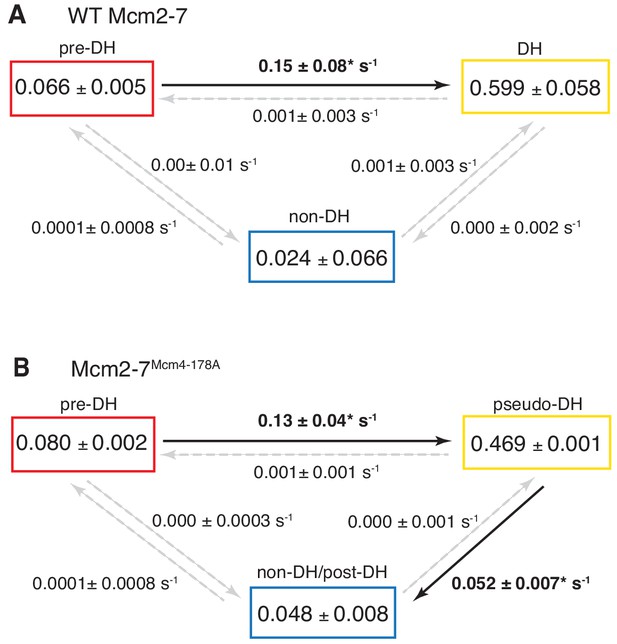
Complete set rate constants (s−1) and EFRET values derived from the kinetic model fits (Figure 6).
Asterisks indicate significantly non-zero rate constants.
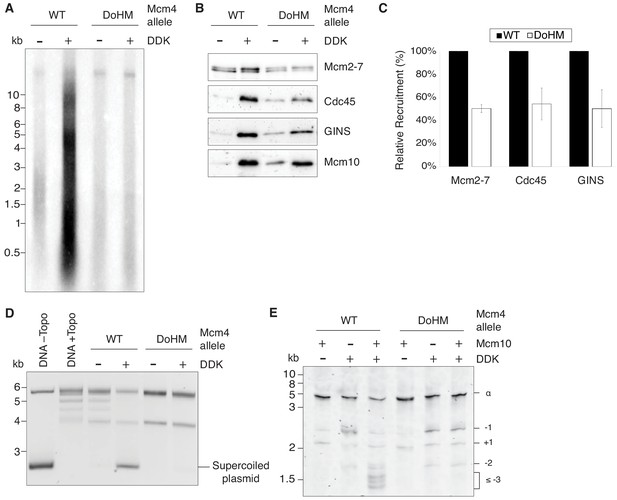
Double-hexamer formation is essential for DNA replication initiation.
(A) The DoHM mutant is defective in DNA replication. The Mcm2-7 complexes were tested in a reconstituted replication assay. The helicase was loaded onto DNA templates and subsequently phosphorylated by DDK. Replication proteins, dNTPs and [α-32P]-dCTP were added to initiate DNA replication, and the replication products were detected by phosphor imaging. Minus-DDK controls show initiation of DNA replication is dependent on DDK phosphorylation. (B) The DoHM mutant can recruit Cdc45, GINS, and Mcm10. Mcm2-7 complexes were tested for CMG formation. The helicase was loaded onto bead-coupled DNA template and subsequently phosphorylated by DDK. The previous reaction mix was removed prior to addition of helicase-activation proteins. Bead-associated proteins were washed with high-salt buffer and detected by immunoblot. Omission of DDK was used as a control for non-specific DNA binding. (Mcm2-7 loading is DDK-independent). (C) The DoHM mutant defect for Cdc45 and GINS recruitment is correlated with the reduced helicase loading observed for this form of Mcm2-7. Data from three independent CMG-formation experiments (including that shown in B) were quantified using ImageJ. Error bars indicate the SD. For each Cdc45 and GINS measurement, the -DDK signal was subtracted from the corresponding +DDK signal. (D) The DoHM mutant is defective for extensive DNA unwinding. Mcm2-7 was loaded onto Topo I-relaxed 3.8 kb plasmids followed by addition of S-CDK, DDK, and helicase-activation proteins to stimulate CMG formation and activation. The reaction was quenched by SDS, separated on an agarose gel, and DNA was detected by ethidium bromide staining. DNA unwinding is detected by the formation of supercoiled DNA and is dependent on DDK phosphorylation. (E) The DoHM mutant allows initial topological changes that occur after CMG formation. Reactions were performed as in (D) except Mcm2-7 complexes were loaded onto a 616 bp circle and unwinding products were detected by SYBR Gold staining. Control reactions lacking DDK or Mcm10 are indicated. Identity of topoisomers is indicated on the right (see Figure 7—figure supplement 1).
-
Figure 7—source data 1
Primary data and quantification for graph in Figure 7C.
- https://doi.org/10.7554/eLife.45538.019
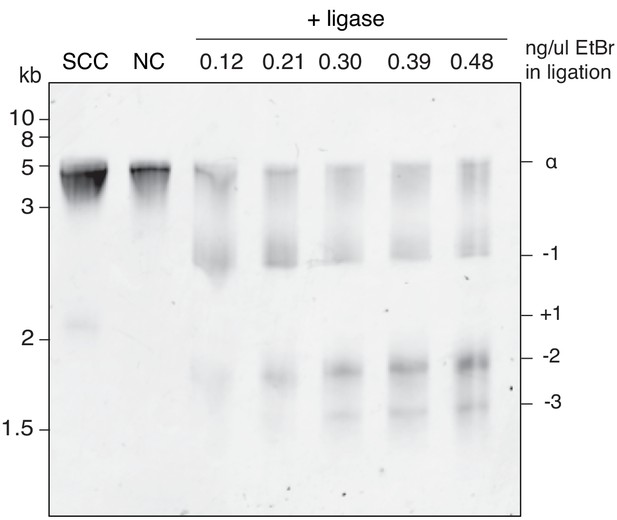
Assignment of relative supercoiling states for Figure 7E.
Nicked, 616 bp DNA circles were ligated in the presence of the indicated concentrations of ethidium bromide (EtBr). DNA circles are increasingly negatively supercoiled as the concentration of EtBr increases. SCC = supercoiled closed-circle before nickase treatment. This state captures the ∝ ground state and +one supercoiling state. NC = nicked circle.
Videos
Illustration of the DoHM interactions in the Mcm2-7 double hexamer (Abid Ali et al., 2017).
The DoHM of Mcm4 (green), the region of Mcm7 that interacts with the DoHM (light blue), and the region of Mcm5 from the opposite hexamer that interacts with the DoHM (yellow) are all shown in space filling representation. The remainder of the structure is shown as a ribbon structure with Mcm6 in orange, Mcm2 in magenta, and Mcm3 in dark blue. Note that there are two DoHM-involved interfaces that are located on opposite sides of the Mcm2-7 double hexamer.
Tables
Reagent type (species) or resource | Designation | Source or reference | Identifiers | Additional information |
---|---|---|---|---|
Antibody | anti-Mcm2-7 | Bell Lab | UM174 | 1:10,000 (rabbit polyclonal) |
Antibody | anti-Cdc45 | Lõoke et al., 2017 | HM7135 | 1:2000 (rabbit polyclonal) |
Antibody | anti-GINS | Lõoke et al., 2017 | HM7128 | 1:2000 (rabbit polyclonal) |
Antibody | anti-Mcm10 | Lõoke et al., 2017 | HM7602 | 1:2000 (rabbit polyclonal) |
Antibody | anti-cMyc agarose | Sigma | Sigma: A7470 | |
Antibody | anti-V5 agarose | Sigma | Sigma: A7345 | |
Chemical compound, drug | DY549-P1-maleimide | Dyomics | Dyomics: 549P1-03 | |
Chemical compound, drug | DY649-P1-maleimide | Dyomics | Dyomics: 649P1-03 | |
Chemical compound, drug | SYBR Gold nucleic acid stain | Invitrogen | Invitrogen: S11494 | |
Commercial assay or kit | illustra MicroSpin G-50 columns | GE Healthcare | GE Healthcare: 27533001 | |
Gene (Saccharomyces cerevisiae) | MCM2 | Saccharomyces Genome Database | SGD: S000000119 | |
Gene (Saccharomyces cerevisiae) | MCM3 | Saccharomyces Genome Database | SGD: S000000758 | |
Gene (Saccharomyces cerevisiae) | MCM4 | Saccharomyces Genome Database | SGD: S000006223 | |
Gene (Saccharomyces cerevisiae) | MCM5 | Saccharomyces Genome Database | SGD: S000004264 | |
Gene (Saccharomyces cerevisiae) | MCM6 | Saccharomyces Genome Database | SGD: S000003169 | |
Gene (Saccharomyces cerevisiae) | MCM7 | Saccharomyces Genome Database | SGD: S000000406 | |
Other | TransFluoSpheres Streptavidin-Labeled Microspheres | Thermo Fisher Scientific | Thermo Fisher: T10711 | |
Other | Amersham Hybond-XL | GE Healthcare | GE Healthcare: RPN303S | Membrane for imaging replication assay |
Peptide, recombinant protein | DNase I | Worthington | Worthington: LS006333 | |
Peptide, recombinant protein | Proteinase K | Thermo Fisher Scientific | Thermo Fisher: AM2548 | |
Peptide, recombinant protein | Nb.BsrDI | New England Biolabs | New England Biolabs: R0648S | |
Peptide, recombinant protein | Peptide for coupling to dyes for Sortase labeling | Ticau et al., 2015 | NH2-CHHHHHHHHHHLPETG | |
Sequence-based reagent | oligo for 1.3 kb template | IDT | 5'-Biotin-GATCGGTGCGGGCCTCTTCGC-3' | |
Sequence-based reagent | oligo for 1.3 kb unlabeled template | IDT | 5'-GGAAAGCGGGCAGTGAGCGC-3' | |
Sequence-based reagent | oligo for 1.3 kb fluorescently-labeled template | IDT | 5'-Alexa488-GGAAAGCGGGCAGTGAGCGC-3' | |
Software, algorithm | Matlab | Mathworks |
Additional files
-
Supplementary file 1
This file contains supplementary table 1 (fit parameters for EFRET distributions), supplementary table 2 (genotypes of yeast and bacterial expression strains used in this study), and supplementary table 3 (plasmids used in this study).
- https://doi.org/10.7554/eLife.45538.021
-
Transparent reporting form
- https://doi.org/10.7554/eLife.45538.022