Retrograde Ret signaling controls sensory pioneer axon outgrowth
Figures
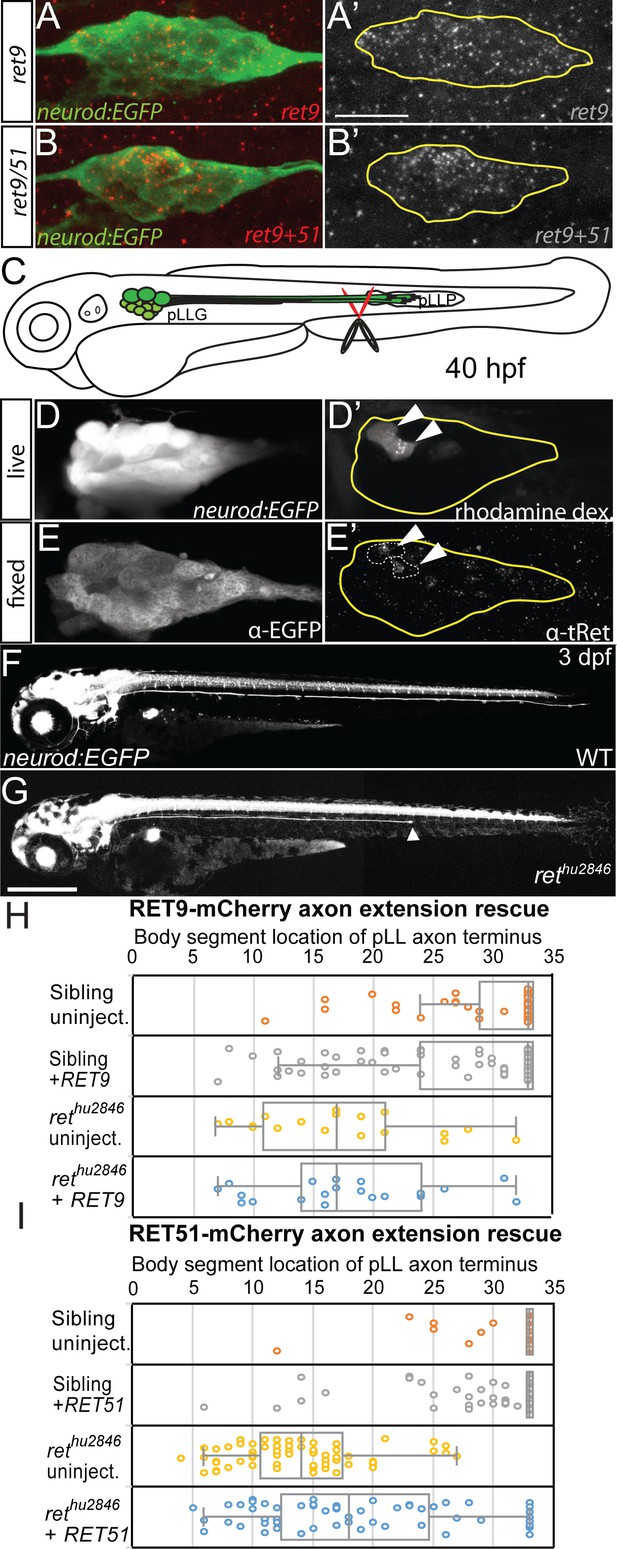
ret is highly expressed in pLL pioneer neurons and required for pLL axon outgrowth.
(A,B) Lateral view of ret9 and ret9+51 probes, visualized by fluorescence in situ hybridization in neurod:EGFP embryos, reveals both are expressed mostly in dorsal cells during axon extension (30 hpf) in the pLLG (yellow line = pLLG outline, scale bar = 20 µm; probe design schematized in Figure 1—figure supplement 1A). (C) Diagram of pLL nerve sever experiment. Distal pioneer axons of neurod:EGFP transgenic embryos were severed during extension using rhodamine dextran soaked scissors. pLLP = posterior lateral line primordium. Live image of EGFP (D) and rhodamine-labeled pLLG pioneer neuron cell bodies (D’) 3 hr post-sever. Immunostaining for EGFP (E) and α-total Ret (E’, tRet) shows enriched Ret protein present in the rhodamine-positive cells (arrowheads) (8 of 10 cells analyzed from n = 5 embryos; Ret antibody validated in Figure 1—figure supplement 1B,C). (F,G) Live lateral view of wild-type siblings (F) and rethu2846 mutants (G) with neurons labeled by the neurod:EGFP transgene. rethu2846 mutants display truncated pLLG axons (arrowhead; scale bar = 200 µm; axon length and pioneer growth cone number over time displayed in Figure 1—figure supplement 2A–D). (H,I) Whisker plots comparing mRNA rescue of pLLG axon truncation defects within the same clutches of rethu2846 mutants with injection of RET9-mCherry (H) or RET51-mCherry (I). RET9-mCherry injection did not significantly ameliorate ret mutant pLLG axon truncation (p=1.00 by one-way ANOVA with post-hoc Tukey test; sibling uninjected n = 50, sibling + RET9 n = 98, rethu2846 uninjected n = 18, rethu2846 + RET9 n = 21); in contrast, RET51-mCherry significantly increased mean pLLG axon length as well as cases of bilateral, full-length axon extension (p<0.001; sibling uninjected n = 70, sibling + RET51 n = 166, rethu2846 uninjected n = 61, rethu2846 + RET51 n = 87).
-
Figure 1—source data 1
Quantification of ret mutant axon truncation rescue with RET9 or RET51 mRNA.
These data can be opened with Microsoft Excel or with open-source alternatives such as OpenOffice.
- https://doi.org/10.7554/eLife.46092.005
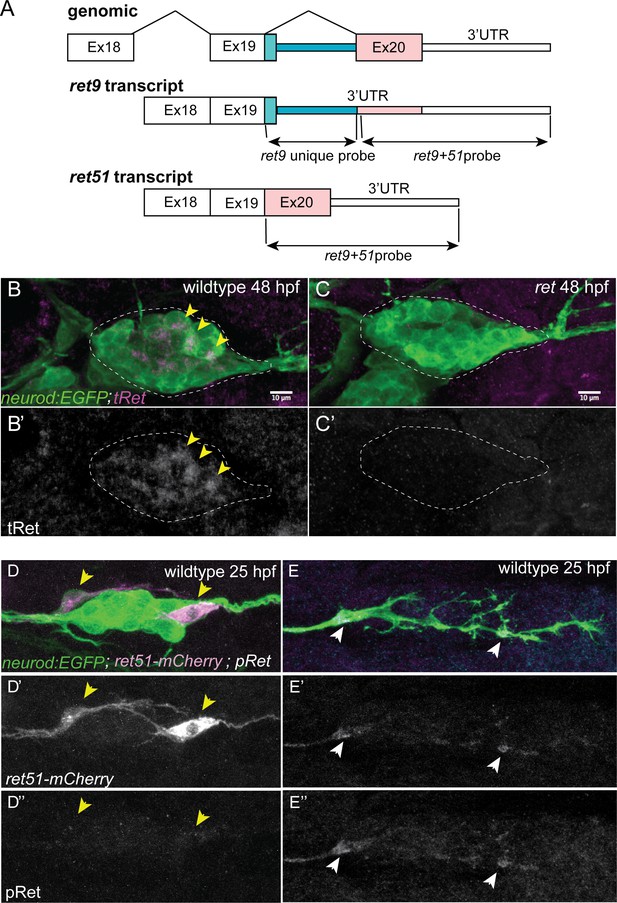
ret mRNA probe design and antibody validation.
(A) Schematic of ret genomic locus, spliced ret9 and ret51 isoforms, and anti-sense mRNA probe targets for fluorescence in situ hybridization in Figure 1. ret9 in situ probe contains the unique portion of exon 19, specific to this isoform, and intron 19–20 sequence, whereas ret9+51 in situ probe encompasess exon 20 (contained within ret9 transcript 3’UTR) and 3’UTR common to both isoforms. (B,C) Whole-mount immunolabeling for total Ret protein of wild-type sibling (B) and rethu2846 mutant (C) with anti-tRet antibody at 48 hpf. neurod:EGFP transgene marks all neurons in the pLL ganglion (outlined). tRet immunoreactivity (arrowheads) is present a subset of wild-type neurons but is completely absent from the rethu2846 mutant. N = 8 wild-type sibs and N = 6 mutants from two independent experiments. (D,E) Whole-mount immunolabeling of wild-type siblings with anti-phospho-Ret (p905) antibody. neurod:egfp-positive embryos were injected with 5 pg of neurod5kb:RET51-mCherry fusion plasmid and fixed at 25 hpf. Anti-pRet antibody recognizes overexpressed RET51-mCherry fusion protein. Panels show immunolabeling of pLL ganglion (D) and pioneer growth cones (E) in wild-type embryo with pRet antibody. Note absence of the signal in the ganglion or cell bodies of neurons that express Ret51-mCherry (yellow arrowheads). However, pRet signal was reliably detected in growth cones (white arrowheads). N = 12 cells from two independent experiments.
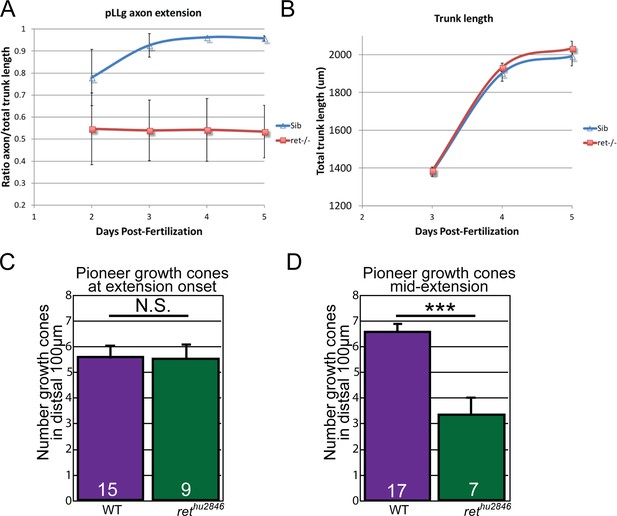
rethu2846 mutants lose distal pioneer growth cones over time but do not display axon retraction.
(A) Ratio of length of extended pLLG axon in neurod:EGFP transgenic embryos over full trunk length from 2 to 5 dpf. rethu2846 mutants (n = 6) display obvious axon truncation by two dpf compared to wild-type siblings (Sib, n = 8), but mutant axons do not retract or degenerate over time (not significant by repeated measures ANOVA with post-hoc Bonferroni test for pairwise comparison; F(3,2)=0.043, p=0.985). (B) Measure of total trunk length sibling and rethu2846 mutant embryos from 3 to 5 dpf. No difference was found, indicating changing axon/trunk length ratio values are not altered by gross trunk morphology changes. (C,D) Number of pioneer pLLG axon growth cones in sibling and rethu2846 mutant embryos at 24 hpf (C) and 30 hpf (D) detected by α-SCG10 immunostaining. rethu2846 mutants display a significantly lower number of pioneer growth cones at 30 hpf, but not 24 hpf. Error bars represent S.E.M., *** = p < 0.001, N.S. = not significant.
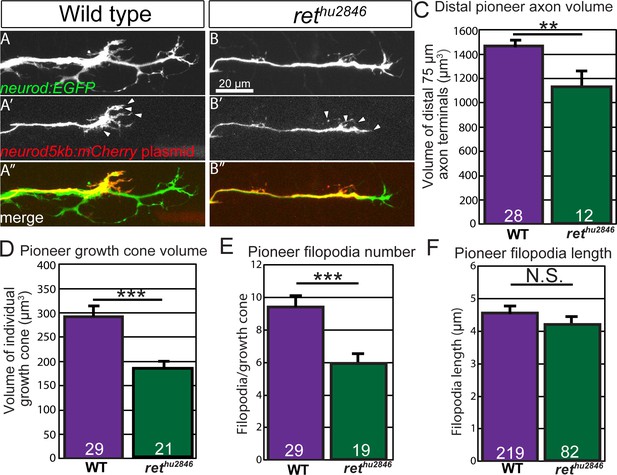
Ret loss-of-function alters pioneer axon growth cone morphology and protrusion.
(A,B) Lateral view, live image of collective pioneer axon terminals of wild-type siblings (A) and rethu2846 mutants (B) labeled by neurod:EGFP transgene at 30 hpf. (A’,B’) Individual pioneer axons labeled by mosaic expression of neurod5kb:mCherry plasmid (Arrowheads = examples of counted filopodia). (C,D) Quantification of distal collective axon terminal volume (C) or individual pioneer growth cone volume (D). rethu2846 mutants have significantly reduced collective axonal and individual growth cone volume. (E,F) Quantification of number (E) and length (F, n = filopodia counted) of filopodia (≥1 μm) per individual pioneer axon. Note that rethu2846 mutants have significantly reduced number of filopodia but not length. Error bars represent S.E.M.,**=p < 0.01, ***=p < 0.001, N.S. = not significant.
-
Figure 2—source data 1
Individual embryo scoring data for scoring of growth cone and filopodia metrics in ret mutants.
These data can be opened with Microsoft Excel or with open-source alternatives such as OpenOffice.
- https://doi.org/10.7554/eLife.46092.007
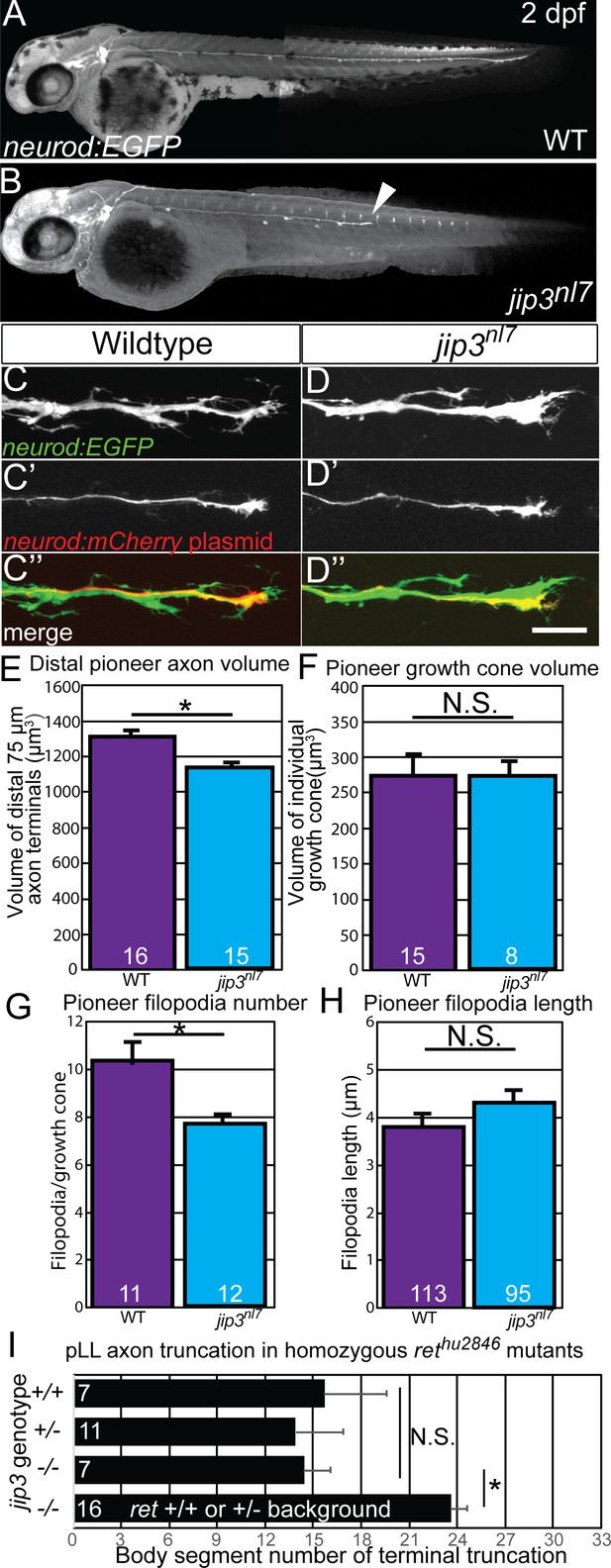
Loss of Jip3 phenocopies ret mutant pioneer axon defects.
(A,B) Lateral view, live image of wild-type sibling (A) and jip3nl7 mutant (B) containing the neurod:EGFP transgene at two dpf. Jip3nl7 mutants display pLLG axon truncation defects (arrowhead; mutant truncation phenotype plotted in Figure 3—figure supplement 1). (C,D) Lateral view, live image of collective pioneer axon terminals of wild-type siblings (C) and jip3nl7 mutants (D) labeled by neurod:EGFP transgene at 30 hpf. Scale bar = 20 μm (C’,D’) Individual pioneer axons in the same animal labeled by mosaic expression of neurod5kb:mCherr plasmid. (E,F) Quantification of distal collective axon terminal volume (E) or individual pioneer growth cone volume (F). Jip3nl7 mutants have significantly reduced collective axonal volume but not individual growth cone volume. (G,H) Quantification of number (G) and length (H) of filopodia (≥1 μm) per individual pioneer axon. Jip3nl7 mutants have significantly reduced number of filopodia but not length. (I) Quantification of axon truncation defects of sibling, heterozygous, and homozygous jip3nl7 mutants in homozygous rethu2846 background shows no significant difference in axon truncation phenotype between genotypes. Jip3nl7 homozygous mutants with +/+ or +/rethu2846 backgound had significantly less severe axon truncations. Error bars represent S.E.M., *=p < 0.05, N.S. = not significant.
-
Figure 3—source data 1
Individual embryo scoring data for scoring of growth cone and filopodia metrics in jip3 mutants and axon truncation in jip3/ret double mutants.
These data can be opened with Microsoft Excel or with open-source alternatives such as OpenOffice.
- https://doi.org/10.7554/eLife.46092.010
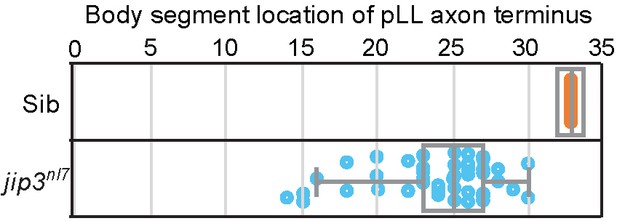
Quantification of jip3nl7 homozygous mutant axon truncation defects.
Whisker plot of body segment location of axon termination at 72 hpf. Siblings (n = 43) display no truncation compared to significant truncation in jip3 mutants (n = 39).
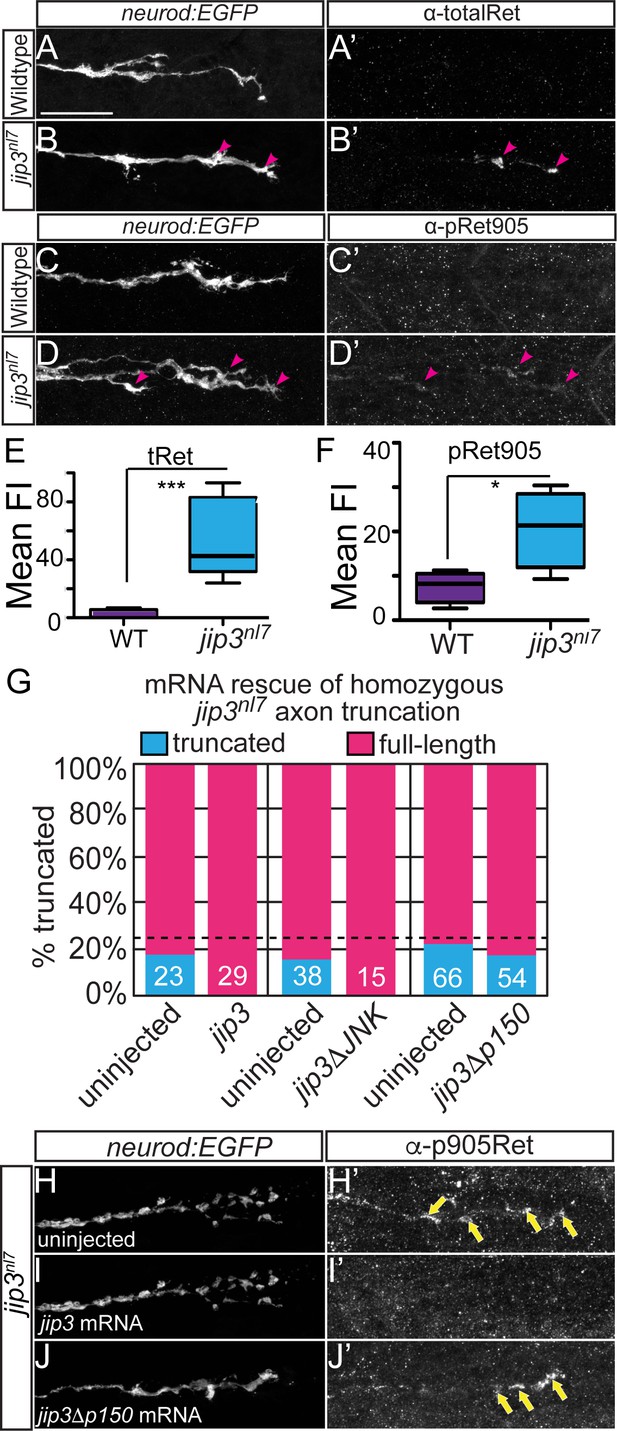
Jip3 is required for clearance of activated Ret from pioneer axon growth cones.
(A–D) Lateral view, immunostained pioneer axon growth cones labeled by neurod:EGFP transgene at 30 hpf. Immunostaining for total Ret receptor (A’,B’, WT n = 8, jip3nl7 n = 5) and activated pRet905 receptor (C’,D’, WT n = 4, jip3nl7 n = 4) shows no signal in wild-type sibling pioneer growth cones (A’,C’) but a notable accumulation of total and pRet905 receptor in jip3nl7 mutants (B’,D’). Scale bar = 30 μm. (E,F) Quantification of immunostaining signal in pioneer growth cones displayed in whisker plots. Both total (tRet) and pRet905 signal is significantly higher in jip3nl7 mutant pioneer growth cones. *=p < 0.05, ***=p < 0.001. (G) Embryos derived from jip3nl7/+ heterozygous crosses were injected with wild-type jip3, jip3 lacking the JNK-binding domain (jip3ΔJNK), or jip3 lacking the p150-binding (jip3Δp150) mRNA. Expression of wild-type and jip3ΔJNK mRNA completely rescued pLLG axon truncation defects but jip3Δp150 did not (phosph-JNK staining in ret mutants quantified in Figure 4—figure supplement 1). Dotted line is drawn at 25%, expected fraction of axon truncation in a heterozygous incross. Numbers represent n embryos. (H–I) Fixed and immunostained jip3nl7 growth cones at 30 hpf from embryos injected with jip3 mRNA constructs. Full-length jip3 mRNA injection (I) rescues pRet accumulated in uninjected pLLG growth cones (H, yellow arrows) but jip3Δp150 (J) does not.
-
Figure 4—source data 1
Ret/phospho-Ret immunostaining quantification and jip3 mutant construct rescue.
These data can be opened with Microsoft Excel or with open-source alternatives such as OpenOffice.
- https://doi.org/10.7554/eLife.46092.013

Anti-pJNK antibody immunostaining of pioneer growth cones.
(A,B) Immunostaining for phosphorylated JNK in pioneer axons in wild type (A) and rethu2846 mutants (B) at 30 hpf. pJNK signal in extending pioneer growth cones looks similar between siblings (A’) and mutants (B’). (C) Mean fluorescence intensity measured for the most distal pLLG growth cones (visualized by neurod:EGFP transgene) was compared between sibling (306 ± 26, n = 14) and rethu2846 mutants (290 ± 51, n = 6) at 30 hpf, as not significantly different (p=0.78 by Mann-Whitney U test). Error bars represent S.E.M.
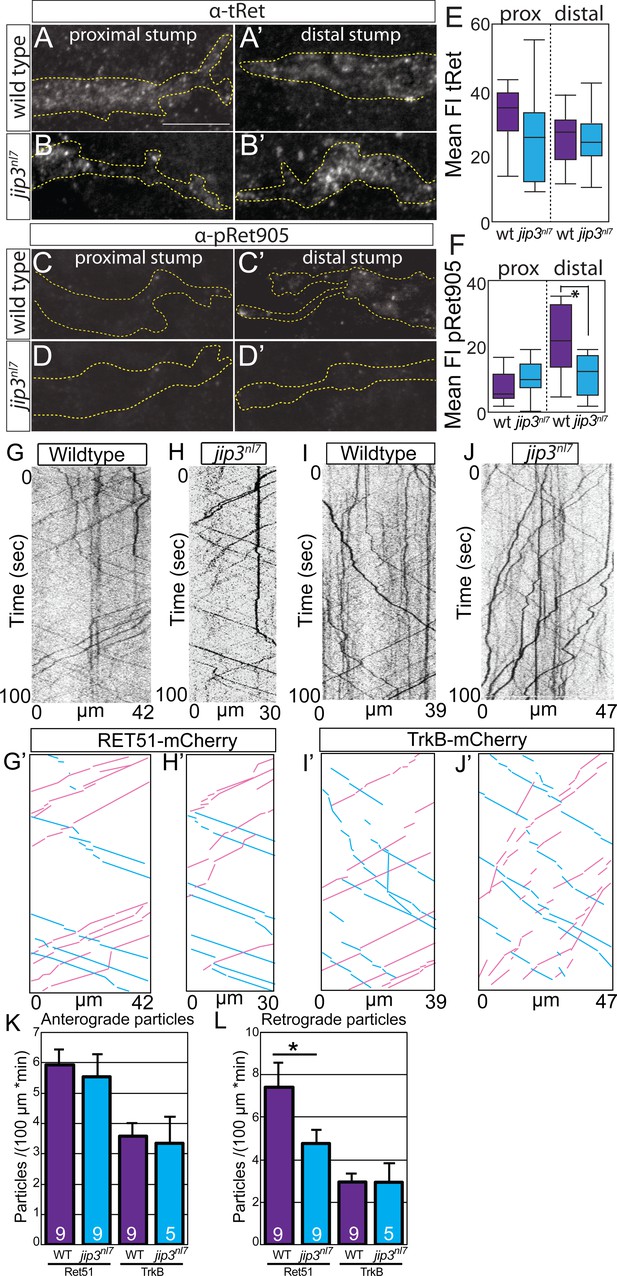
Jip3 mediates retrograde axonal transport of activated Ret51.
Lateral view, immunostaining of severed pLL nerves proximal (A–D) and distal (A’–D’) to injury site, 3 hr post-injury; dotted line = nerve outline. (A,B) tRet immunostaining is not notably different between wild-type siblings (A,A’) and jip3nl7 mutants (B,B’) on either side of injury site. (C,D) p905Ret staining is mostly absent in wild-type siblings proximal to injury site (C) and jip3nl7 mutants on both sides of the injury site (D,D’). Scale bar = 10 μm. Stronger p905Ret staining signal is present in wild-type siblings distal to the injury site (C’), indicating presence of retrograde transport of activated Ret receptor in the axon. (E,F) Quantification of tRet (E; WT n = 12, jip3nl7 n = 14) and p905Ret (F; WT n = 12, jip3nl7 n = 12) staining following axon sever displayed by whisker plot (*=p < 0.05). Note p905Ret signal in wild-type siblings is significantly higher than in jip3nl7 mutants, suggesting a failure of retrograde transport of pRet receptor in mutants. (G–J) Kymographs of trafficking in individual pLLG axons of Ret51-mCherry (G,H; Ret51 construct trafficking further shown in Figure 5—figure supplement 1) and TrkB-mCherry fusions (I,J) in wild-type siblings and jip3nl7 mutants. (G’–J’) Kymograph traces of scored anterograde (blue) and retrograde (magenta) transported particles. (K) Quantification of normalized anterograde particle counts from kymograph analysis (wild type Ret51 = 5.93 ± 0.52 vs. jip3nl7 Ret51 = 5.53 ± 0.76, p=0.44 by Mann-Whitney U test; wild type TrkB = 3.58 ± 0.42 vs. jip3nl7 TrkB = 3.36 ± 0.86, p=0.82). (L) Quantification of normalized retrograde particle counts from kymograph analysis (wild type Ret51 = 7.33 ± 1.17 vs. jip3nl7 Ret51 = 4.73 ± 0.53, p=0.03; wild type TrkB = 3.07 ± 0.31 vs. jip3nl7 TrkB = 3.06 ± 0.75, p=1.00). jip3nl7 mutants show a significant decrease in the number of retrogradely transported Ret51-mCherry particles but no change in TrkB-mCherry particle counts or anterograde Ret51-mCherry particles. *=p < 0.05. Error bars represent S.E.M.
-
Figure 5—source data 1
Quantification of immunostaining and kymograph analysis.
These data can be opened with Microsoft Excel or with open-source alternatives such as OpenOffice.
- https://doi.org/10.7554/eLife.46092.016
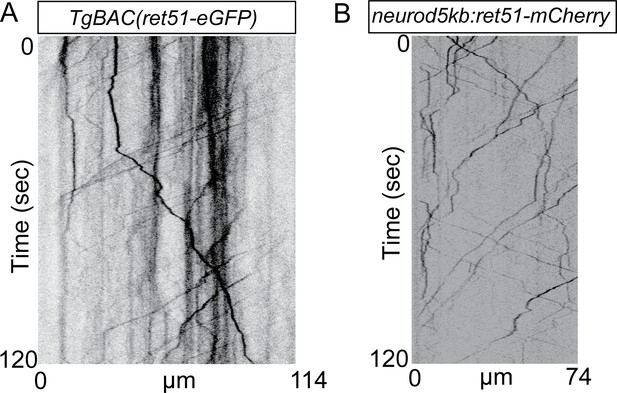
In vivo imaging of Ret51 trafficking.
(A) Kymographs generated from the pLLG pioneer axons of embryos injected with BAC expressing GFP-tagged zebrafish Ret51. (B) Kymograph generated from the pLLG axons of embryos injected with plasmid expressing mCherry-tagged RET51. Discrete tagged particles of Ret51 are trafficked in both cases anterogradely and retrogradely.
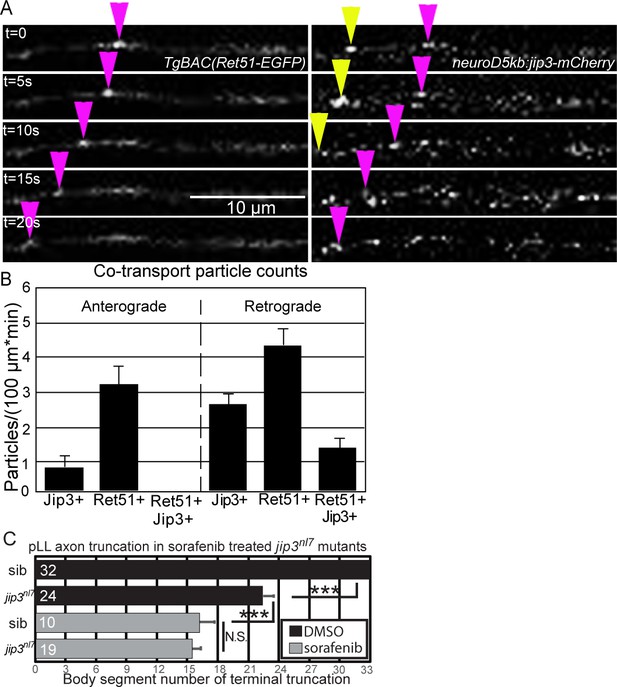
Ret51 and Jip3 co-transport in extending pLLG axons in vivo.
(A) Still images from Figure 6—video 1 of pLLG axonal transport in embryos co-injected with neurod5kb:jip3-mCherry and Tg(BAC)Ret51-egfp plasmids. Retrograde transport of mCherry+ eGFP+ particles can be seen (magenta arrowhead) as well as solely GFP+ particles (yellow arrowhead). (B) Quantification of Jip3-mCherry and Ret51-eGFP normalized particle counts (n = 5 embryos). Anterograde Ret51+ Jip3+ particles were not observed, however retrograde Ret51+ Jip3+ particles were seen. (C) Quantification of axon truncation defects of wild-type sibling and jip3nl7 mutant larvae at 72 hpf treated with DMSO or 5 μM sorafenib from 24 to 72 hpf (Sorafenib specificity confirmed with ret mutants in Figure 6—figure supplement 1). Sorafenib treatment produces a more severe truncation phenotype than the jip3nl7 mutation alone, but loss of Jip3 does not exacerbate the sorafenib-induced truncation phenotype. ***=p < 0.001, N.S. = not significant. Error bars represent S.E.M.
-
Figure 6—source data 1
Kymograph analysis of Jip3/Ret51 co-transport experiments.
These data can be opened with Microsoft Excel or with open-source alternatives such as OpenOffice.
- https://doi.org/10.7554/eLife.46092.019
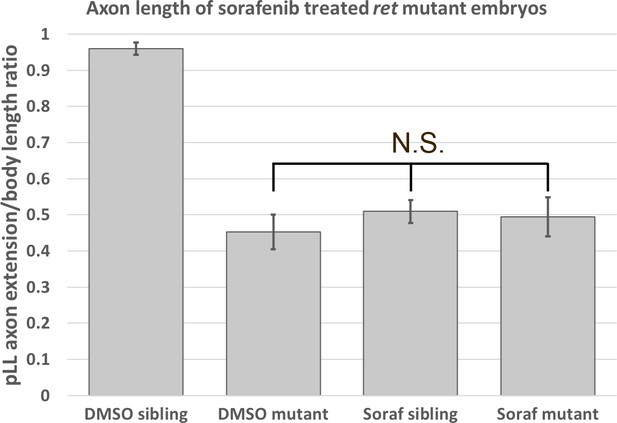
Sorafenib induces pLLG axon truncation similar to that found in Ret genetic loss-of-function at 72 hpf.
Treatment with 5 μM sorafenib from 24 to 72 hpf induced axon truncations in wild-type siblings (0.50 ± 0.06; n = 44) not significantly different than untreated ret homozygous mutants (0.53 ± 0.03; n = 17; p=0.95 by one way ANOVA with post-hoc Tukey test) or sorafenib-treated ret homozygous mutants (0.50 ± 0.05; n = 16; p=0.92). Error bars represent S.E.M., N.S. = Not significant.
Time lapse image of Ret51-Jip3 co-transport.
Merged time lapse of Ret51-EGFP (green channel) and Jip3-mCherry (red channel) axonal transport acquired at three frames/s. Retrograde transport of Ret51+ particles is marked by yellow arrowhead, anterograde transport of Ret51+ particle is marked by white arrowhead, and retrograde co-transport of Jip3+ Ret51+ particles is marked by magenta arrowhead. Scale bar = 5 μm.
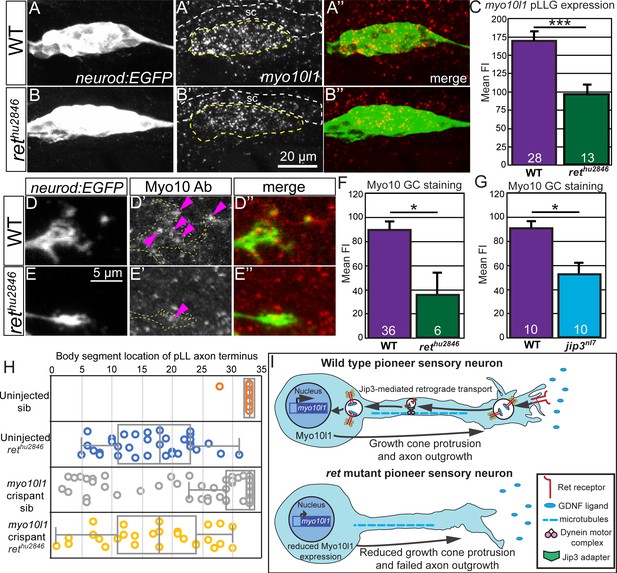
Myosin-X expression and growth cone localization are reduced in rethu2846 mutants.
(A,B) Lateral view, pLL ganglia marked by neurod:EGFP of wild type and rethu2846 mutants at 30 hpf. (A’,B’) Fluorescent in situ hybridization shows decreased expression of myosin-10l1 in ret mutants (yellow dotted line, ganglion; sc and white dotted line, spinal cord). (C) Quantification of mean fluorescence intensity of fluorescent in situ hybridization reveals significantly lower expression of myosin-10l1 in ret mutant pLL ganglia. (D,E) pLL pioneer axon growth cones at 30hpf marked by neurod:EGFP expression. (D’,E’) Immunostaining for Myo10 shows a decrease in overall fluorescence and puncta (magenta arrowheads) in rethu2846 mutants (Antibody confirmation shown in Figure 7—figure supplement 1, panel A). (F,G) Quantification of mean fluorescence intensity of Myo10 immunostaining in the distal 50 µm of pLL pioneer axons shows a significant decrease in Myo10 localization in rethu2846 (F) and jip3nl7mutants (G). (H) Whisker plot of sibling and ret mutant larvae (neurod:EGFP) injected with myo10l1 gRNA/Cas9. Myo10l1 CRISPR injection in wild-type ret siblings-induced axon truncation in many cases but did not significantly exacerbate axon truncation in ret mutants (myo10l1 transcript and CRISPR targeting shown in Figure 7—figure supplement 1, panels B,C). p. Error bars represent mean S.E.M., *=p < 0.05, ***=p < 0.001 (one-way ANOVA with post hoc Tukey test). (I) Proposed model of the contribution of long-range retrograde Ret signaling to axon outgrowth of pioneer sensory neurons.
-
Figure 7—source data 1
Quantification of FISH/immunostaining and scoring ret mutant/myo10l1 crispant axon truncation.
- https://doi.org/10.7554/eLife.46092.023
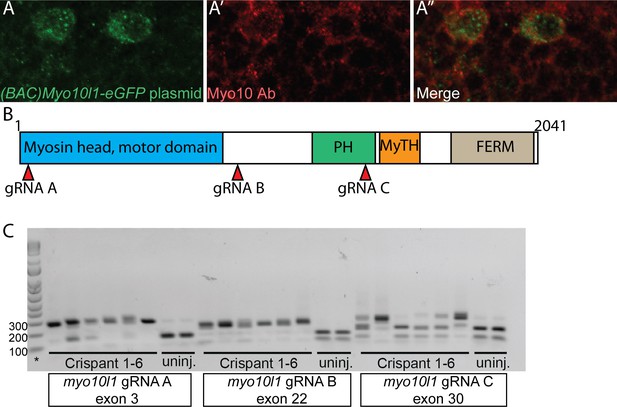
Assessment of anti-Myo10 antibody and myo10l1 gRNA efficiency.
(A) Myo10 antibody staining for neurons in embryos injected with myo10l1-eGFP BAC. Rohon-Beard neurons expressing Myo10l1-eGFP (A) are also positive for Myo10 antibody staining (A’). (B) Scale diagram of zebrafish Myo10l1 protein. gRNAs target distinct sites in myo10l1 exons corresponding to specific regions of the protein (red arrowheads). PH = Pleckstrin homology domain, MyTH = Myosin tail homology four domain, FERM = 4.1 protein, Ezrin, Radixin, Moesin domain. (C) Amplification of targeted CRISPR cut target regions containing restriction cut sites. Gel electrophoresis (2% agarose gel) of PCR amplification of each CRISPR cut site from genomic DNA of individual four dpf larvae injected with gRNA triplex cocktail that display significant pLLG truncation or uninjected embryos. Following PCR, amplicons are incubated with restriction enzymes that cut near CRISPR target sites. Uninjected embryos have near complete digest of amplicons at each CRISPR target site, but triplex-injected crispants that display pLLG truncation show substantial amounts of resistance to digest. *=1 kb plus ladder.
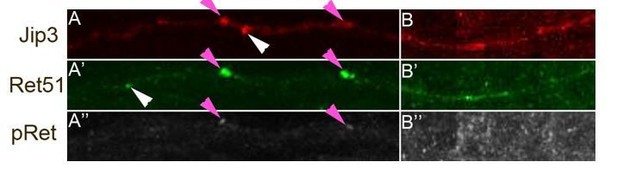
Triple antibody staining for Jip3-mcherry, Ret51-eGFP, and phospho-Ret (Y905).
(A) We detected instances of axonal puncta that were triple positive (magenta arrowheads), as well as Jip3 and Ret51 particles that were not positive for the other markers (white arrowheads). However, in most cases (B), staining was variable and distinguishing puncta and reducing background were difficult to achieve. Note that in this case Jip3 and Ret51 signal is not localized to distinct puncta, as observed during live imaging.
Tables
Reagent type (species) or resource | Designation | Source or reference | Identifiers | Additional information |
---|---|---|---|---|
Strain (Danio rerio) | TgBAC(neurod:EGFP)nl1; neurod:EGFP | doi: 10.1523/JNEUROSCI.5230–07.2008 | ||
Strain (Danio rerio) | rethu2846 | doi: 10.1242/dev.061002 | ZIRC Catalog ID: ZL3218 | |
Strain (Danio rerio) | jip3nl7 | doi: 10.1371/journal.pgen.1003303 | ||
Antibody | SCG10(STMN2) antibody (rabbit polyclonal) | ProteinTech | Cat. No.: 10586-AP | 1:100 |
Antibody | GFP (rabbit polyclonal) | Invitrogen | Cat. No.: A11122 | 1:1000 |
Antibody | GFP (chick polyclonal) | Aves Labs | Cat. No.: GFP-1020 | 1:1500 |
Antibody | Phospho-Ret (pTyr905) (rabbit polyclonal) | Invitrogen | Cat. No.: PA517761 | 1:50 |
Antibody | Ret (rabbit polyclonal) | Santa Cruz Biotechnology | Cat. No.: sc-167 | 1:100 |
Antibody | Neurofilament (mouse monoclonal) | Developmental Studies Hybridoma Bank | Designation: 3A10 | 1:500 |
Antibody | Myosin-X (rabbit polyclonal) | ProteinTech | Cat. No.: 24565–1-AP | 1:500 |
Antibody | Phospho-JNK (Thr183/Tyr185) (rabbit polyclonal) | Cell Signaling | Cat. No.: 9251S | 1:100 |
Antibody | Goat anti-chicken Alexa Fluor 488 secondary antibody | Invitrogen | Cat. No.: A-11039 | 1:1000 |
Antibody | Goat anti-rabbit Alexa Fluor 568 secondary antibody | Invitrogen | Cat. No.: A-11008 | 1:1000 |
Antibody | Goat anti-mouse Alexa Fluor 568 secondary antibody | Invitrogen | Cat. No.: A-11004 | 1:1000 |
Antibody | Cy5 Goat anti-mouse IgG secondary antibody | Jackson Immunoresearch Laboratories | Cat. No.: 115-175-003 | 1:1000 |
Antibody | Goat anti-rabbit Alexa Fluor 647 secondary antibody | Jackson Immunoresearch Laboratories | Cat. No.: A-27040 | 1:1000 |
Recombinant DNA reagent | pCDNA3.1-RET9-mCherry | doi: 10.1111/tra.12315 | ||
Recombinant DNA reagent | pCDNA3.1-RET51-mCherry | doi: 10.1111/tra.12315 | ||
Recombinant DNA reagent | neurod5kb:mCherry | This paper | Gateway destination plasmid pDestTol2pA2, recombination described in ‘Plasmids and tagged constructs’ in Materialsand methods | |
Recombinant DNA reagent | neurod5kb:RET51-mcherry | This paper | Gateway destination plasmid pDestTol2pA2, recombination described in ‘Plasmids and tagged constructs’ in Materialsand methods | |
Recombinant DNA reagent | TgBAC(ret51-eGFP) | This paper | Contains DKEY-192P21 clone, with 49.89 kb upstream and 68.78 kb downstream sequence of ret | |
Recombinant DNA reagent | TgBAC(myo10l1-eGFP) | This paper | Conatins clone DKEY-10E5, with 20.98 kb upstream and 48.7 kb downstream sequence of myo10l1 | |
Chemical compound, drug | sorafenib | Cell Signaling | Cat. No.: 8705 | 5 μM in 0.5% DMSO in embryo medium |
Software, algorithm | Imaris | Bitplane | v8.4.2 | |
Software, algorithm | SPSS Statistics | IBM | v25 |
PCR primers and oligonucleotides.
https://doi.org/10.7554/eLife.46092.024Δp150forward | GGGAAAGAAGTGGAAAATGAGGAGCTGGAATCGGTA |
Δp150reverse | TTTACCGATTCCAGCTCCTCATTTTCCACTTCTTTC |
ΔJNKforward | GAGGAAAAGTAAAACAGGTGGAGATGGCATGGAGGA |
ΔpJNKreverse | CCATGCCATCTCCACCTGTTTTACTTTTCCTCCAGT |
CRISPR Myo10l1 A | aattaatacgactcactataGGTGGTGTACCTGCAGCAGGgttttagagctagaaatagc |
CRISPR Myo10l1 B | aattaatacgactcactataGGAGGATACCCGCCAGATGGgttttagagctagaaatagc |
CRISPR Myo10l1 C | aattaatacgactcactataGGACAAGAGTTCCTGGTCAGgttttagagctagaaatagc |
Myo10l1 A primer F | TCCTCTCCCCTTTTGTGAAGTA |
Myo10l1 A primer R | TGTCTTTCTGGTATCGCTGATG |
Myo10l1 B primer F | AATCCTTTCAGAGTTGCAGACA |
Myo10l1 B primer R | CGTGGACACACTGTCTTCTCTT |
Myo10l1 C primer F | CTGTAACTCAGACCTGCCAGAA |
Myo10l1 C primer R | CCTGTGTCACAAAAGCAACATT |
Ret 3’RACE-d(T) | GACCACGCGTATCGATGTCGACTTTTTTTTTTTTTTTTV |
Ret 3’RACE anchor | GACCACGCGTATCGATGTCGAC |
Ret-3'RACE-F | CGAACCCTCCCCTCCACTTG |
Ret9 unique 3’RACE F | AGAATTTCCCATGCATTTACTAGA |
Ret9+51 3’RACE F | CGCTCAACAGACTCGATGCC |
Ret9 unique probe R | CTGCTGCGGTGACATTGTAT |
Ret51 BAC rec F | ggctttgcattctcccgcaaaaatcgtggacacaatcgatagtatggtgagcaagggcga |
Ret51 BAC rec R | caccccccgtgtcttttccgccattgattttggcttgcgttttgcagaattcgcccttga |
My10l1 BAC rec F | cgctgcagcatcatgtccatcaccagcaacagcagcgcctggaatggtgagcaagggcga |
My10l1 BAC rec R | ttgtgtagctgtacatttggagctctgggtggtgtcgatcacctgcagaattcgcccttg |
Additional files
-
Transparent reporting form
- https://doi.org/10.7554/eLife.46092.025