Electrostatic lateral interactions drive ESCRT-III heteropolymer assembly
Figures
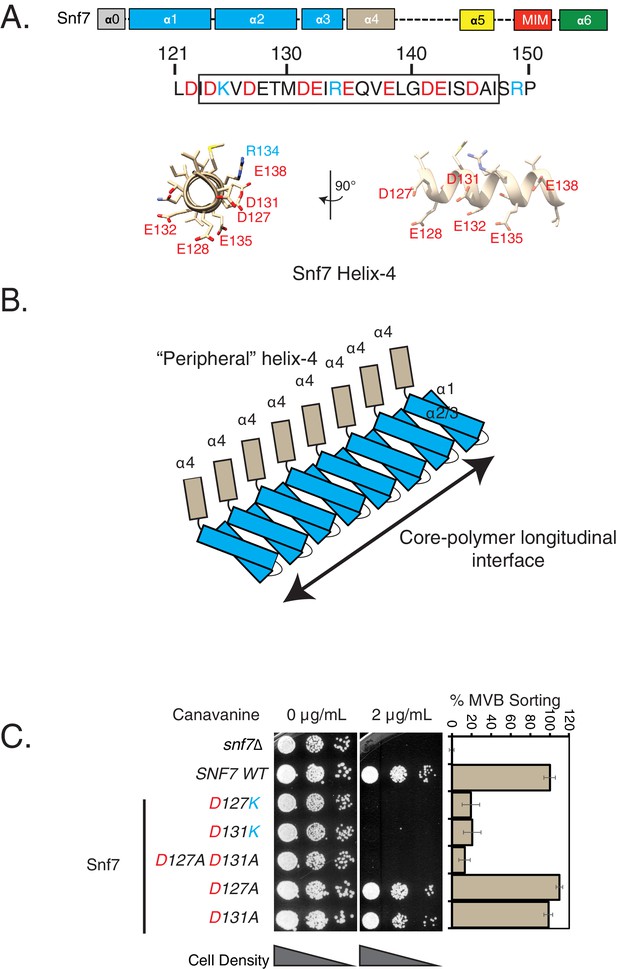
Mutations in helix-4 region of Snf7 induce cargo-sorting defect.
(A) Domain organization of Snf7, depicting the different helices (top). Bottom figure shows the sequence of helix-4, with the predicted helical motif highlighted with a box. Acidic residues are denoted in red, while cyan residues are basic amino acids. Bottom – structure of the helix-4 (from PDB 5fd7) in two orientations, highlighting the acidic residues on one surface. (B) Cartoon model of the polymeric arrangement of Snf7 in its linear form observed in the crystal lattice. (C) Canavanine sensitivity and Mup1-pHluorin flow-cytometry data (right) showing cargo-sorting/endocytosis defects of the helix-4 mutants of Snf7. Mup1-pHluorin data were collected 90 min after methionine addition. Error bars represent standard deviation from 3 to 7 independent experiments.
-
Figure 1—source data 1
Individual data points for data plotted in the figure (Figure 1C) for % MVB sorting.
- https://doi.org/10.7554/eLife.46207.008
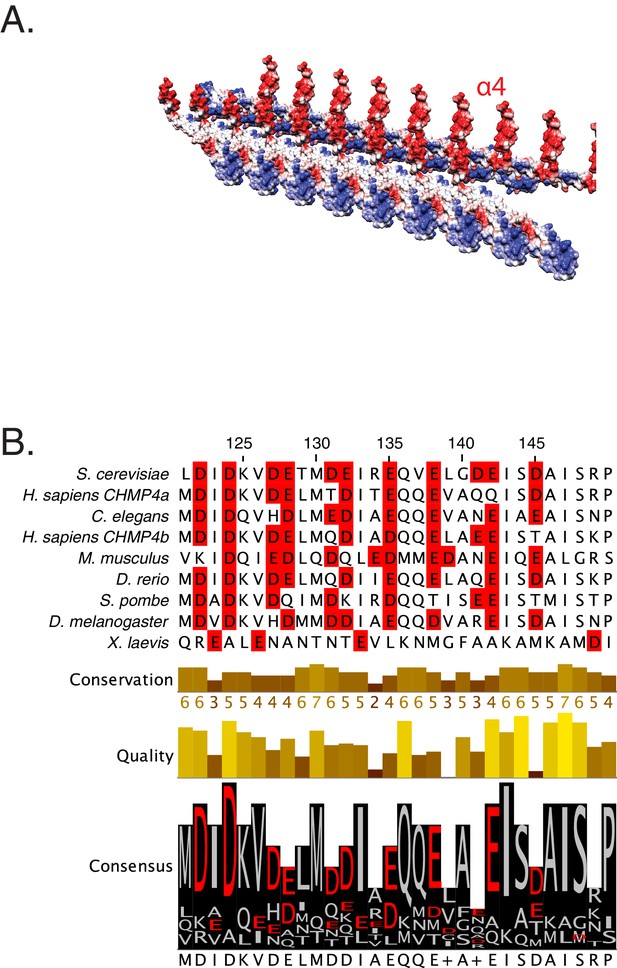
Snf helix-4 consists of conserved acidic residues.
(A) Electrostatic depiction of the crystal lattice of Snf7 showing interaction between two laterally interacting strands of Snf7. Helix-4 (α4) is observed to be predominantly acidic in nature. (B) Sequence conservation of the helix-4 region of Snf7, with acidic residues highlighted in red.
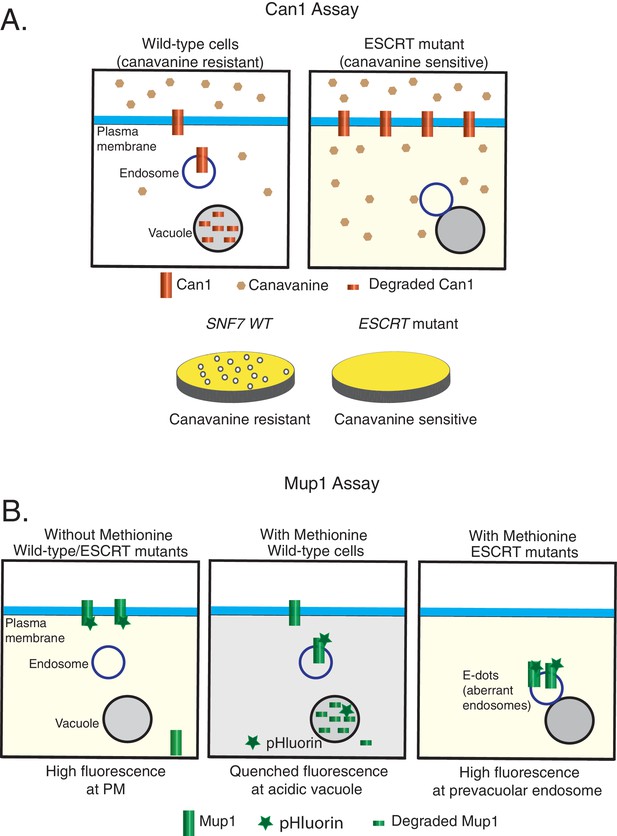
Models of the cargo-sorting assays used in this study using Saccharomyces cerevisiae.
Canavanine-sensitivity assay (A) and Mup1-pHluorin assay (B). In ESCRT mutants, the model membrane proteins are unable to be trafficked to the vacuole for degradation.
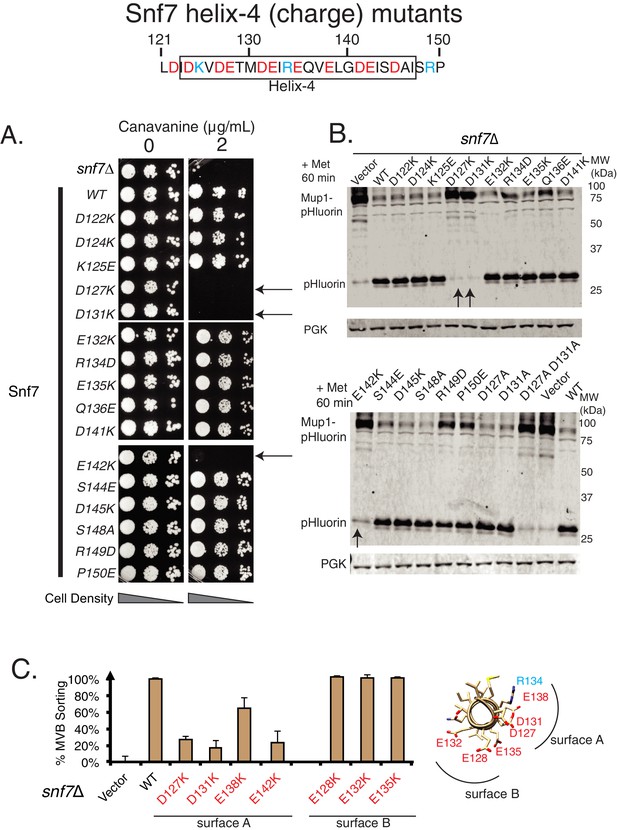
Mutational analysis of helix-4 residues of Snf7.
Canavanine sensitivity assay (A) and Mup1-pHluorin degradation analysis (B) of several charge mutations in helix-4 residues of Snf7. Arrows point to the most sensitive mutations. (C) Mup1-pHluorin flow cytometry data of the mutations in two different surfaces (figure on the right) of Snf7 helix-4. Error bars represent standard deviation from three independent experiments.
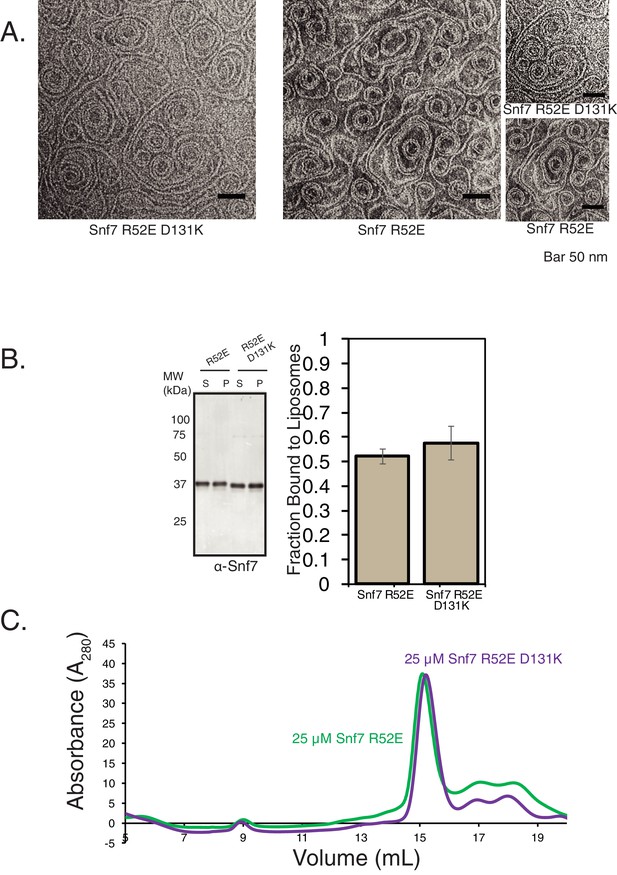
Helix-4 mutation does not affect stability of Snf7.
(A) Electron microscopy (EM) images of Snf7 R52E and Snf7 R52E D131K mutations. Images on the far right are zoomed-in pictures. 10 μM Snf7 R52E and 10 μM Snf7 R52E D131K were used, incubated for 10 min on EM grids. (B) In vitro membrane-binding properties of Snf7 R52E and Snf7 R52E D131K. Experiments were performed with 200 nM of proteins and 0.5 mg/mL liposomes. Quantification on the right represent data from three technical replicates (three experiments with the same protein and lipid preparation samples). (C) Size-exclusion profiles of Snf7 R52E and Snf7 R52E D131K performed using an SD200increase column.
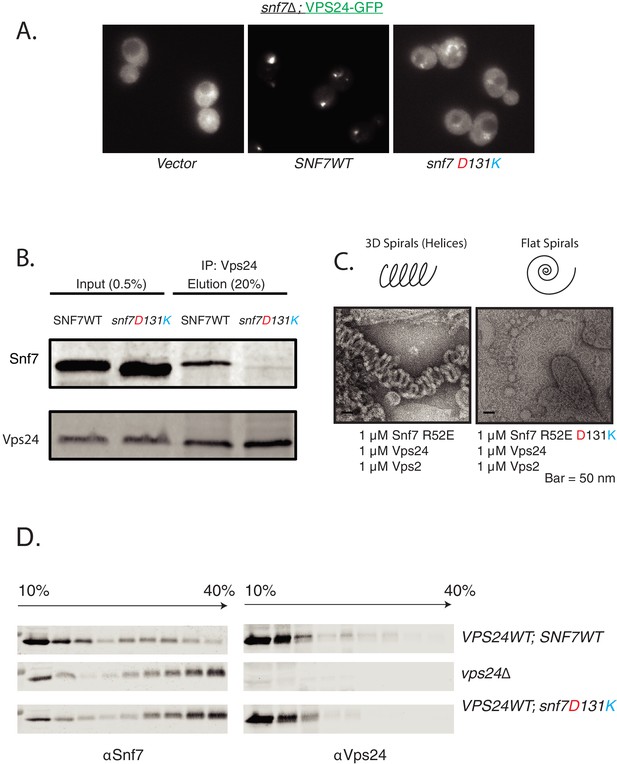
Helix-4 region of Snf7 is involved in recruiting Vps24.
(A) Helix-4 mutants fail to recruit Vps24-GFP. Vps24-GFP is present in endosomal dots in cells expressing SNF7 WT on a plasmid in a snf7Δ;Vps24-GFP strain, while with the mutation on Snf7, Vps24-GFP is more diffuse in the cytoplasm. (B) Coimmunoprecipitation experiments, immunoprecipitating Vps24 and blotting for either wild-type Snf7 or the mutant D131K. (C) Electron microscopy assay on lipid monolayers, depicting co-assembly of Snf7 R52E with Vps24 and Vps2 into helices. Snf7 R52E D131K fails to form similar structures. Experiments were done with 1 μM of each protein incubated for 1 hr on lipid monolayers. (D) ‘In vivo’ glycerol-gradient experiments using a gradient of 10% to 40%, using various mutant strains annotated on the far-right. Western-blots were performed against Snf7 (left) or Vps24 (right).
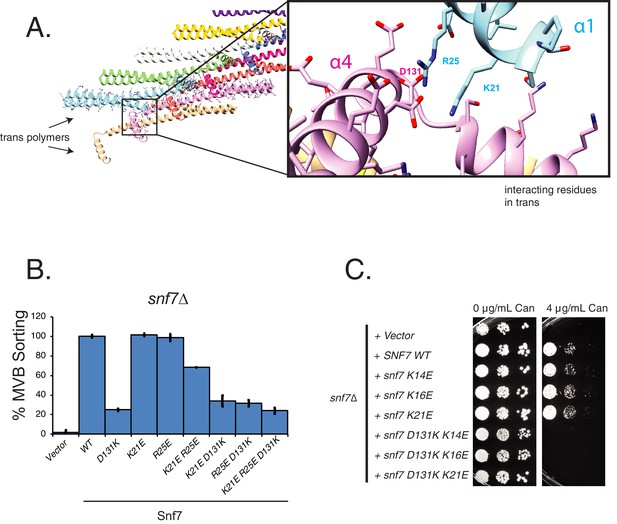
Helix-4 contacts a trans Snf7 polymer in the crystal lattice.
(A) Depiction of the contact between helix-4 of Snf7 with residues of helix-1, a trans polymer in the lattice. Zoomed-in box shows image of the D131 interacting with K21 and R25 of helix-1. (B) MVB-sorting (Mup-pHluorin degradation) of helix-1 mutants of Snf7. Error bars represent standard deviation from three independent experiments. (C) Canavanine sensitivity assay of helix-1 mutants of Snf7.
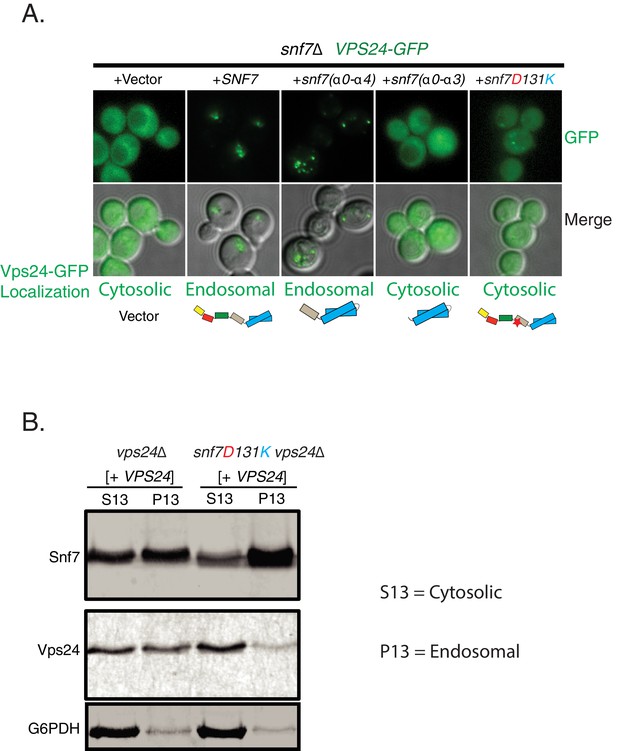
Helix-4 region of Snf7 is involved in recruiting Vps24.
(A) Vps24-GFP recruitment experiment in a snf7Δ;Vps24-GFP strain expressing different mutants of Snf7 on a plasmid. (B) Membrane-fractionation experiments with wild-type or mutant Snf7 D131K showing relative amounts of Vps24 pelleted to endosomal (P13) or cytosolic (S13) fractions.
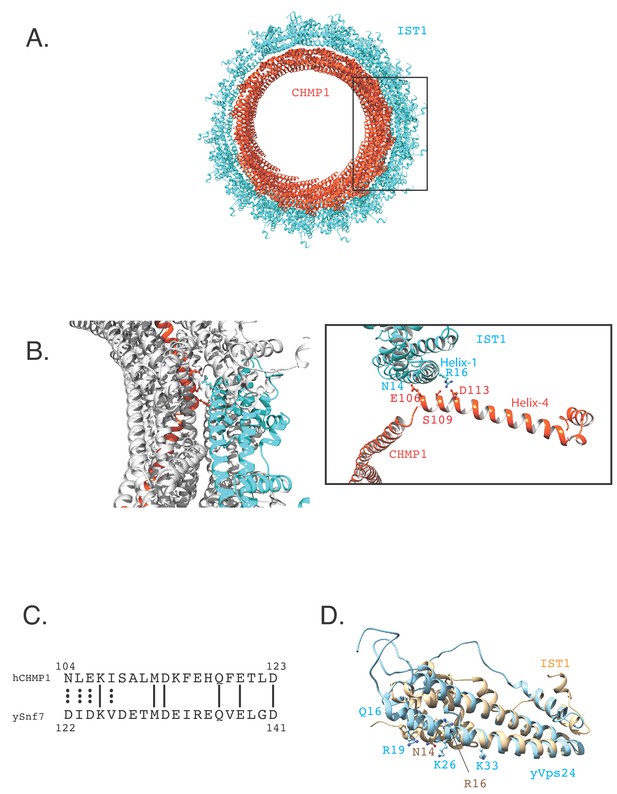
CHMP1B helix-4 contacts the helix-1 of IST1.
(A).The cryo-EM structure of CHMP1B-IST1 copolymer (PDB 3JC1, McCullough et al., 2015). CHMP1B is colored in orange-red and IST1 is depicted in cyan. (B).Zoomed-in image of the contact between the helix-1 residues of IST1 with helix-4 residues of CHMP1B. (C).Sequence alignment of a part of helix-4 of human CHMP1B and yeast Snf7. (D).Overlay of the IST1 structure (PDB 3FRR, Bajorek et al., 2009b) with the homology model of yeast Vps24 (yVps24). The crystal structure of CHMP3 (PDB 3FRT, Bajorek et al., 2009b) was used to create the homology model.
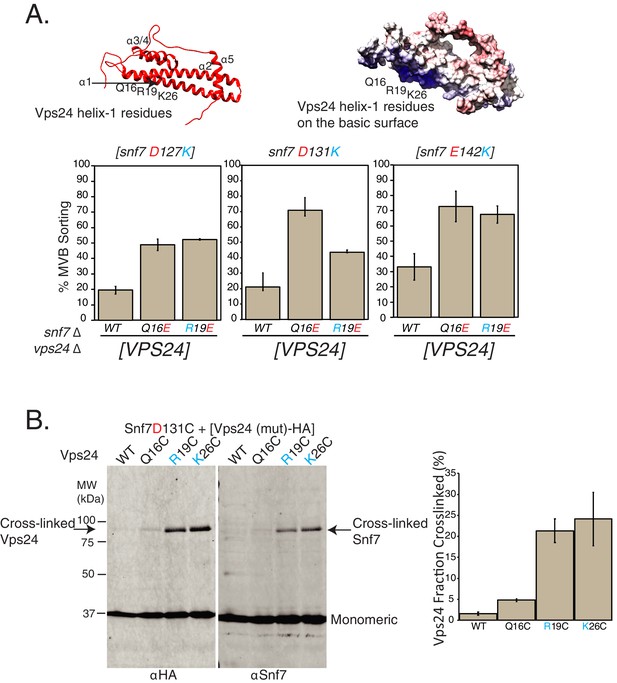
Random mutagenesis selection identified suppressors of Snf7-helix-4 mutations on Vps24.
(A) Locations of random mutations on the homology model of Vps24 (top), obtained through canavanine-resistance selection with mutagenized Vps24. The crystal structure of CHMP3 (PDB 3FRT) was used to create the homology model. Figure on the top-right shows the electrostatics of the same model. Graphs below represent Mup1-pHluorin assays, where the mutations in helix-1 of Vps24 rescue the defects of the indicated Snf7 mutants. Error bars represent standard deviation from three to five independent experiments. (B) Ex vivo BMOE mediated crosslinking assays between cysteines in Snf7 and Vps24 at the indicated positions. Immunoblots were performed against Snf7 or HA (on Vps24). Arrows indicate crosslinked species. Quantification on the right represents three independent replicates of the fraction of Vps24 (mutant) crosslinked with Snf7 D131C.
-
Figure 3—source data 1
Individual data points for data plotted in the figure (Figure 3A) for % MVB sorting.
- https://doi.org/10.7554/eLife.46207.016
-
Figure 3—source data 2
Data plotted in the figure for % Vps24-HA crosslinked in Figure 3B.
- https://doi.org/10.7554/eLife.46207.017
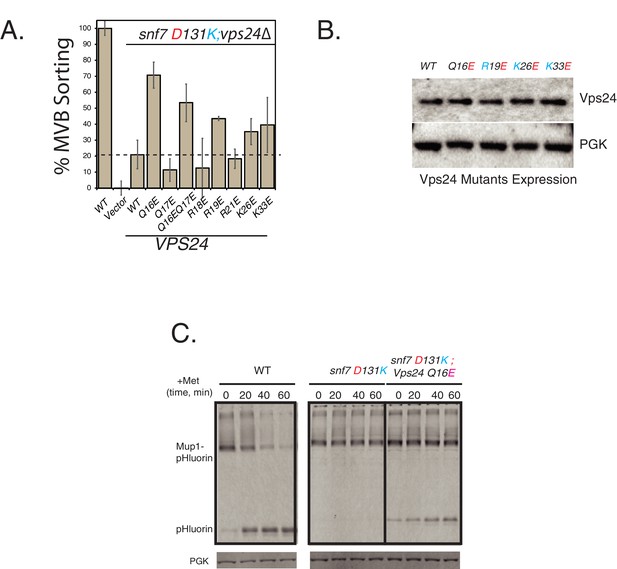
Identification of the surface of Vps24 that interacts with Snf7 helix-4.
(A) Mup1-pHluorin flow-cytometry assay with acidic charge mutations in helix-1 of Vps24 in the background of the snf7 D131K mutant. Error bars represent standard deviation from three independent experiments. (B) Expression levels of various helix-1 mutants of Vps24 used in this study. (C) Kinetic analysis of the suppression of helix-4 mutant snf7 D131K for cargo sorting defects of Mup1-pHluorin. Samples were collected for immunoblots every 20 min after addition of methionine.
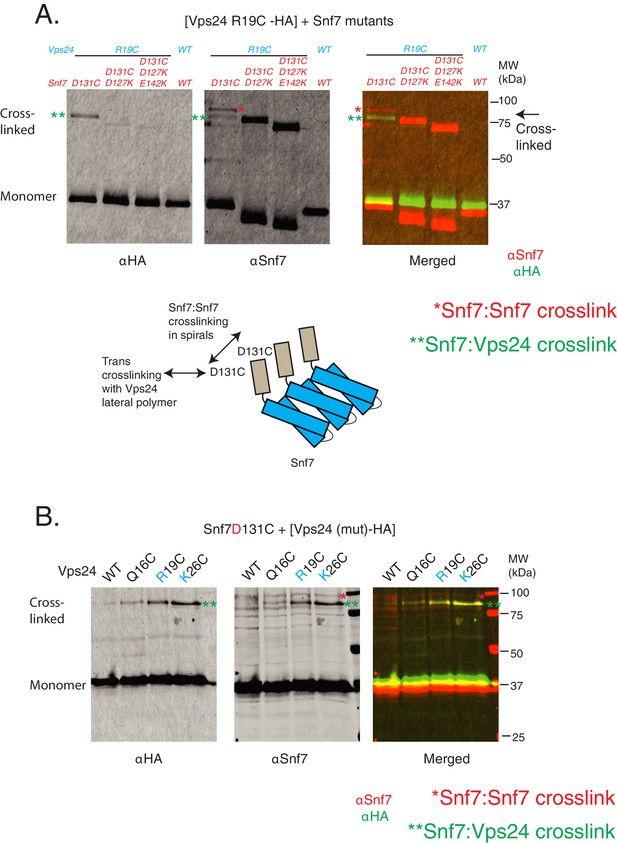
Crosslinking analyses of Snf7 and Vps24.
(A) Ex vivo BMOE mediated crosslinking assays between Snf7 D131C and Vps24-R19C at indicated cysteine mutations, in the presence of additional background mutations in Snf7 helix-4. Immunoblots were performed against HA tag on Vps24 (left) or with Snf7 (middle). The merged image is shown on the right panel, with Snf7 blot in red and the Vps24-HA blot in green. The bottom figure illustrates how the cis-crosslinking of Snf7 with Snf7 is possible with the D131C mutation. (B) BMOE mediated crosslinking between Snf7 D131C and mutants of Vps24-HA (as in Figure 3B). Here, the crosslinked Snf7:Snf7 species are clearly separated from the Snf7:Vps24 species.
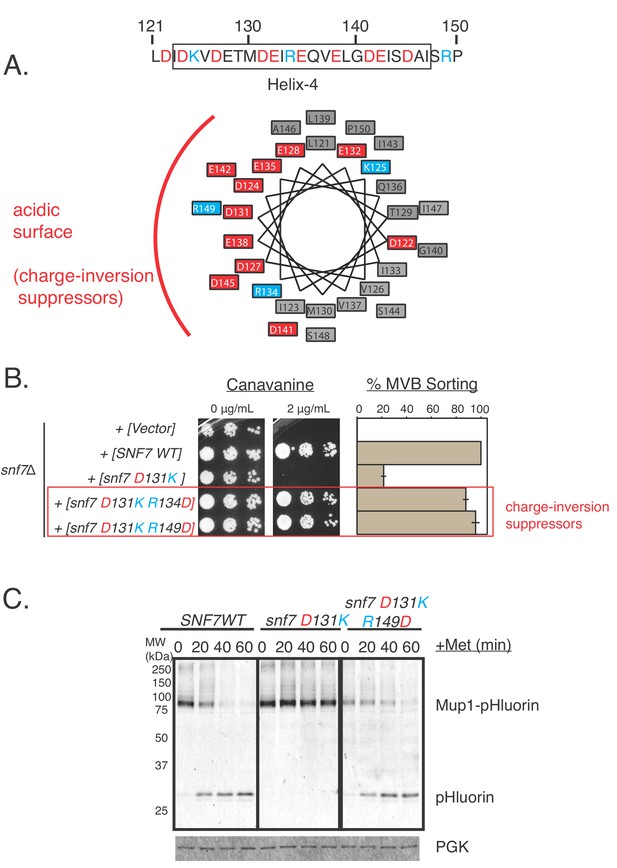
Random mutagenesis approach discovered charge-inversion suppressors in helix-4 of Snf7.
(A) Helical-wheel representation of the helix-4 region of Snf7, highlighting acidic (red) and basic (cyan) residues. (B) Canavanine-sensitivity (left) and Mup1-pHluorin assays (right). Opposite charge mutations (positive to negative charges) rescue cargo-sorting defects of Snf7 mutants. Error bars represent standard deviation from three independent experiments. (C) Similar experiments as in (B - right) showing Mup1-pHluorin degradation over time after addition of methionine with mutants snf7 D131K and snf7 D131K R149D, immunoblotting for pHluorin.
-
Figure 4—source data 1
Individual data points for the %MVB sorting of Snf7 mutant and the charge suppressor in Figure 4B.
- https://doi.org/10.7554/eLife.46207.020
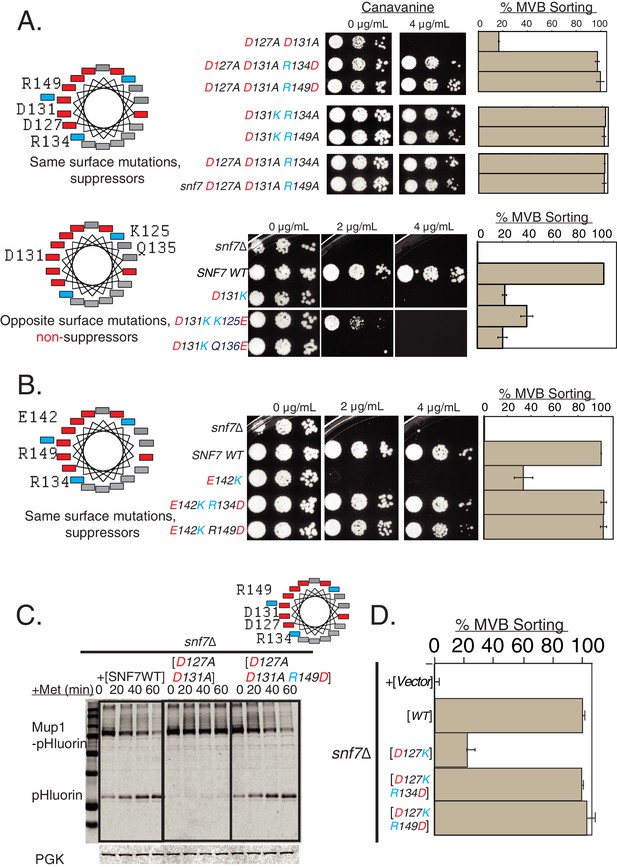
Charge-inversion in helix-4 rescue cargo-sorting defects of mutants.
(A) Helix-wheel on the left indicate the positions of the mutations analyzed in this figure. Canavanine sensitivity or Mup1-pHluorin assays with opposite charge mutations in helix-4. With D127A D131A mutations including the R134D or R149D, the endocytosis defect is restored. Mutating R134 or R149 to alanine also suppress the defect of D131K, and also suppress the mutant D127A D131A. Bottom-panel depict mutations in opposite surface of helix-4, which do not suppress the defects observed in endocytosis. Error bars represent standard deviation from three independent experiments. (B) Similar Can1 and Mup1 assays with mutations at the E142 location of Snf7. The same surface charge-rebalancing mutations (E142K R134D or E142K R149D) are completely functional in cargo-sorting assays. Error bars represent standard deviation from three independent experiments. (C) Mup1-pHluorin degradation over time after addition of methionine with mutants snf7 D127A D131A and snf7 D127A D131A R149D, blotting for pHluorin. Adding a Glu in place of Arg to helix-4 rescues the defect of the double mutation D127A D131A. (D) Quantification of the Mup1-pHluorin assays with mutations at the D127 position of Snf7, whose defect is rescued by charge-balancing mutations in the helix. Error bars represent standard deviation from three independent experiments.
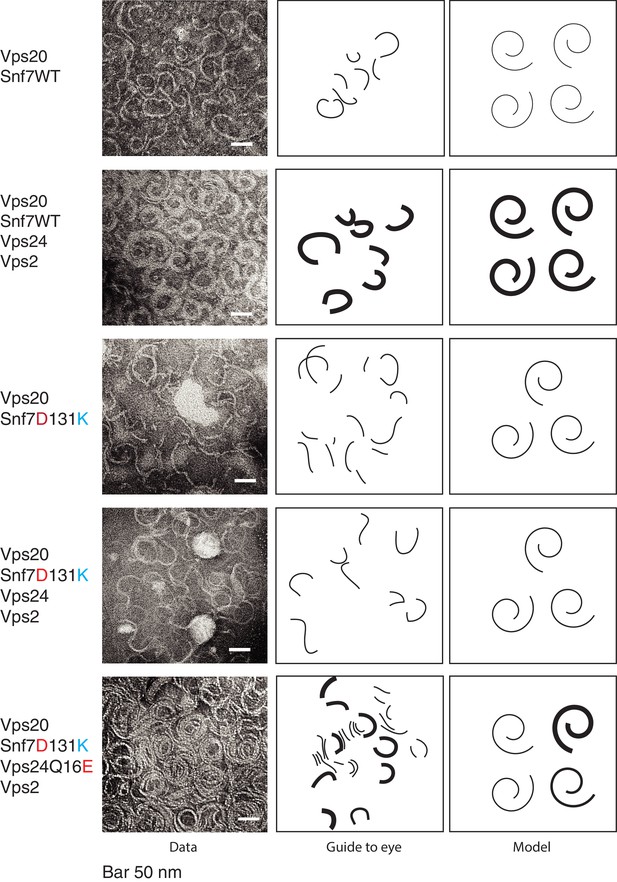
Vps24/Vps2 form lateral copolymer with Snf7.
(A) Electron microscopy analysis of 10 μM Vps20, 1 μM Snf7 or (Snf7 D13K), 1 μM Vps24 (or Vps24 Q16E) and 1 μM Vps2. Experiments were done on lipid monolayers, with incubation of proteins for 1 hr. Painted lines on the middle-panel are guide to the eye to demonstrate differences in the thickness of the copolymers. The right panel shows hypothetical model of the structures of the polymers made from the different proteins.
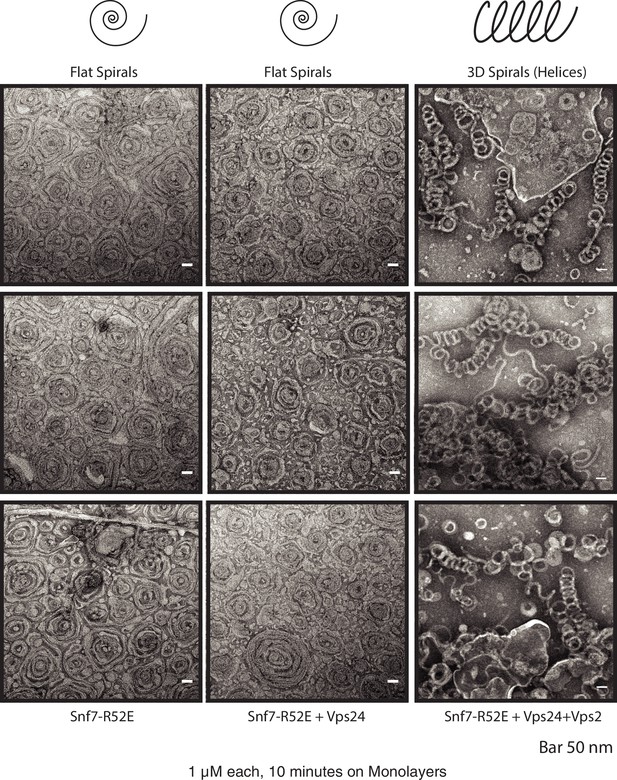
Vps24/Vps2 form 3D helices at higher activation conditions of Snf7.
First column depicts spirals of 1 μM Snf7 R52E. Upon addition of 1 μM Vps24 (second column), the spirals remain 2-dimensional. 3D helices form with 1 μM Snf7 R52E, 1 μM Vps24 and 1 μM Vps2 (right column). Three different images of the polymers under each condition are shown. Images were collected after 10 min of incubation on lipid monolayers.
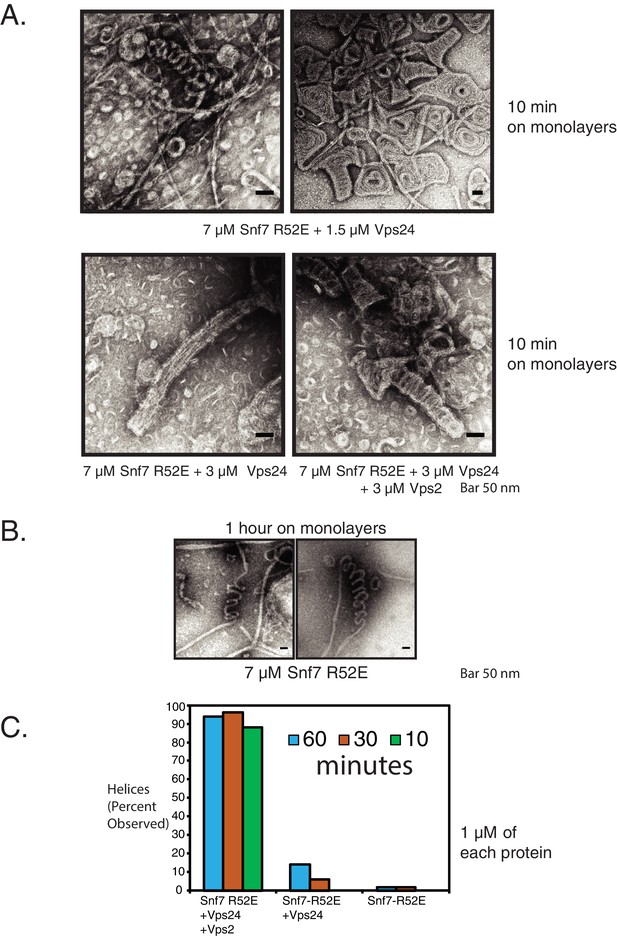
Snf7/Vps24/Vps2 form 3D helices at higher activation conditions.
(A) Snf7 R52E and Vps24 form 3D helices of different morphologies at higher concentrations (7 μM Snf7 R52E). Helix formation is more robust with the inclusion of all three proteins (bottom right). (B) ‘Helical’ structures of Snf7 R52E alone, which form at low frequency (Figure 5—figure supplement 2C). (C) Kinetic analysis of the assembly of Snf7 R52E, Vps24 and Vps2 at different concentrations and time. Quantification indicates percentage of images in which helices were observed. Quantification was performed with 50 images under each condition, with images taken at random spots on the grid.
-
Figure 5—figure supplement 2—source data 1
Snf7/Vps24/Vps2 helices formation is higher when all three proteins are present.
The zip file contains images used to present the quantification in Figure 5—figure supplement 2C. For easy uploading and downloading, the sizes of the images have been downsized by 5.68 fold, using the Adjust size option in ImageJ, constraining the aspect ratio and using the bilinear interpolation option. In the images, 1 pixel equals 4.87 nm.
- https://doi.org/10.7554/eLife.46207.024
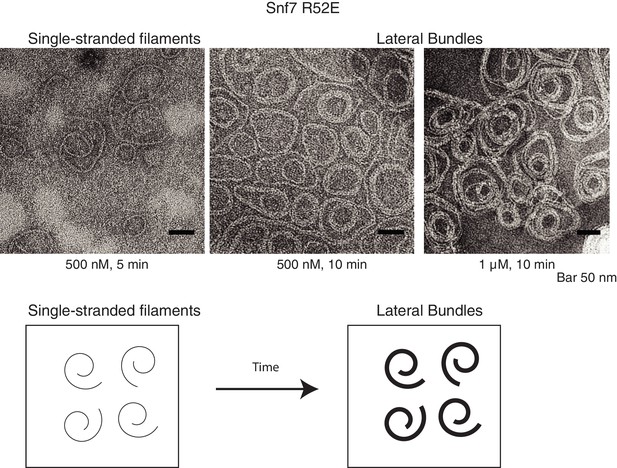
Snf7 filament progresses into lateral bundles over time.
Electron microscopy images of 500 nM Snf7 R52E protein on lipid monolayers at 5 and 10 min. With 1 μM Snf7 R52E, mostly bundles of polymers are seen at 10 min (right).
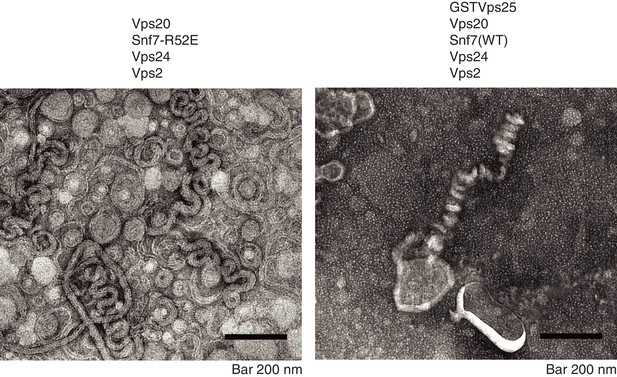
Snf7 activation allows ESCRT-III helix formation.
A)Electron microscopy images of 10 μM Vps20, 1 μM Snf7 R52E, 1 μM Vps24 and 1 μM Vps2, incubated for one hour on lipid monolayers. B) Electron microscopy images of 5 μM GST-Vps25, 10 μM Vps20, 1 μM Snf7 (WT), 1 μM Vps24 and 1 μM Vps2, incubated for one hour on lipid monolayers.
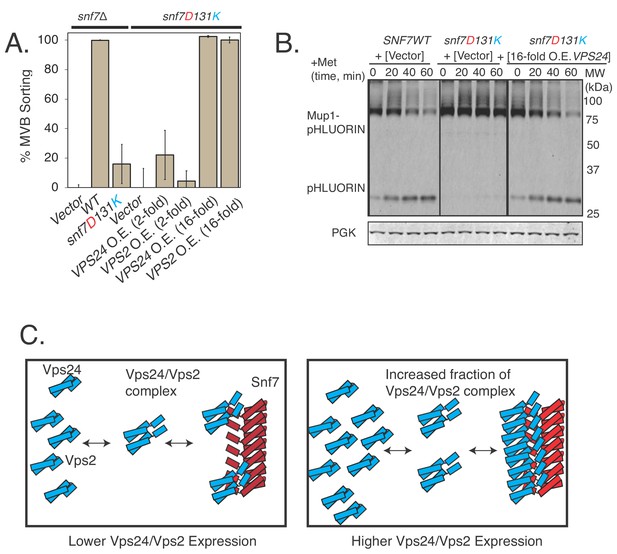
Vps24 and Vps2 cooperatively bind Snf7, as over-expressing them suppresses the defects of Snf7 helix-4 mutants.
(A) Mup1-pHluorin endocytosis data after 90 min of methionine addition, showing overexpression of Vps24 or Vps2 rescuing the defect of the helix-4 mutant D131K. 2-fold overexpression (O.E.) corresponds to expressing either Vps24 or Vps2 with a CEN plasmid, while ~16 fold represents experiments performed with these genes on a CMV promoter with a tet-off operator. Error bars represent standard deviation from three to five independent experiments. (B).Overexpression of Vps24 (on a plasmid with a tet-off operator), rescues Mup1-pHluorin degradation defect of the D131K mutant, as shown immunoblotting of pHluorin over 60 min of methionine addition. (C).Model showing the hypothesized recruitment of Vps24/Vps2 in helix-4 mutant under normal (left) or under overexpressed (right) Vps24/Vps2 conditions.
-
Figure 6—source data 1
Individual data points for the % MVB sorting of Snf7 mutant and suppression through overexpression of Vps24 and Vps2.
- https://doi.org/10.7554/eLife.46207.029
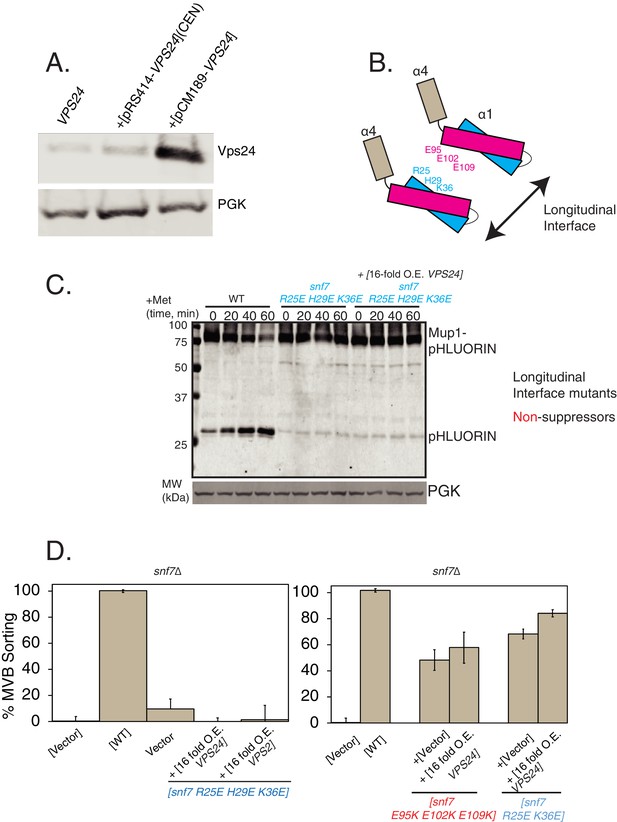
Overexpression of Vps24 or Vps2 do not suppress the defects of longitudinal polymerization mutants of Snf7.
(A) Expression levels of Vps24 at endogenous, overexpressed with CEN plasmid, or overexpressed with the pCM189 plasmid which contains a CMV promoter and a tet-off operator.(B) Cartoon depiction of the locations of the Snf7 mutants used in the subsequent experiments. R25, H29 and K36 fall on helix-1 of Snf7, which make contacts with the Snf7 helix-3 residues E95, E105 and E109 in the longitudinal polymeric arrangement of Snf7. (C) Mup1-pHluorin degradation experiments, blotting for pHluorin over time, with Snf7 mutants, while overexpressing Vps24. (D) Mup1-pHluorin flow-cytometry data under overexpression of Vps24 or Vps2 with Snf7 mutants. Error bars represent standard deviation from three independent experiments.
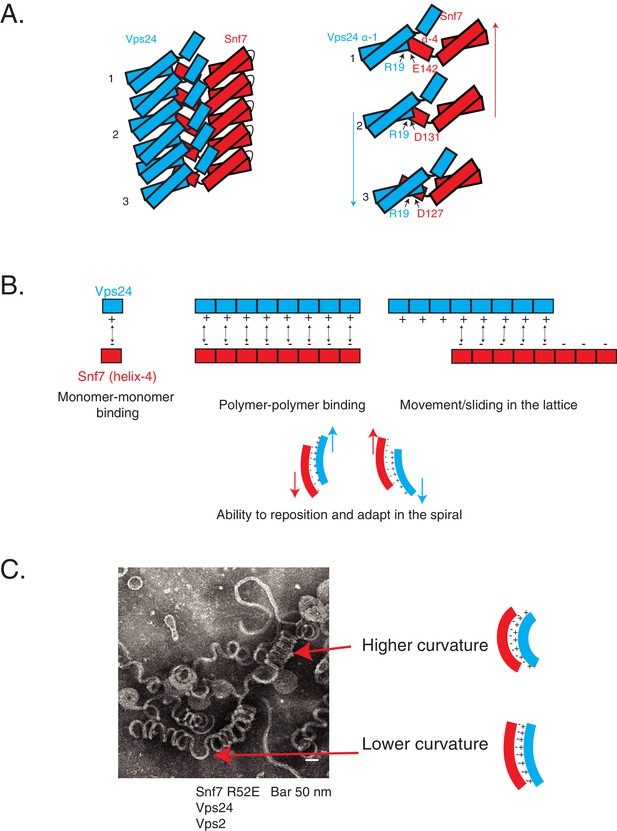
Cooperative lateral associations define ESCRT-III polymers.
(A) Cartoon model of Snf7 filament interacting with Vps24 filament in trans in lateral fashion. On right, as an example, residue R19 of Vps24 is shown to interact with three different acidic residues of Snf7’s helix-4, indicating its different positions along the polymer. Arrows represent possible sliding of the side-by-side polymers. (B) Model depicting laterally interacting polymers, in which multiple electrostatic interaction sites are present between the polymers, with the binding sites devoid of a specific registry between the residues involved in the interaction. The non-specific nature of the interaction could enable the polymers to slide along one another (top-right) and reposition and adapt (bottom) in the copolymer. (C) Electron microscopy image of 3D helices of Snf7 R52E, Vps24 and Vps2. Two positions are highlighted with arrows and corresponding models of the laterally interacting/sliding polymers are depicted on the right.
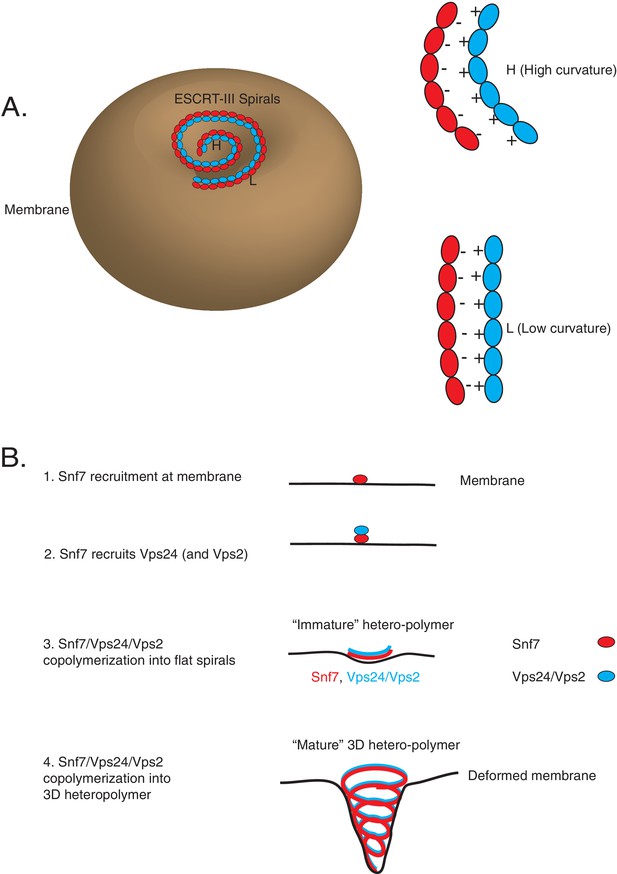
Co-assembly of ESCRT-III proteins into membrane-deforming polymers drive budding reactions.
(A) Figure on the left depicts a model of ESCRT-III spirals budding membranes by utilizing different curvatures. Figure on the right depicts how laterally associating polymers can reposition themselves utilizing electrostatics at different curvatures. In the figures, H represent a position of high curvature and L represents a position of low curvature. (B) Model depicting different steps of the simultaneous recruitment of Snf7/Vps24/Vps2, which form spirals that drive membrane budding reactions.
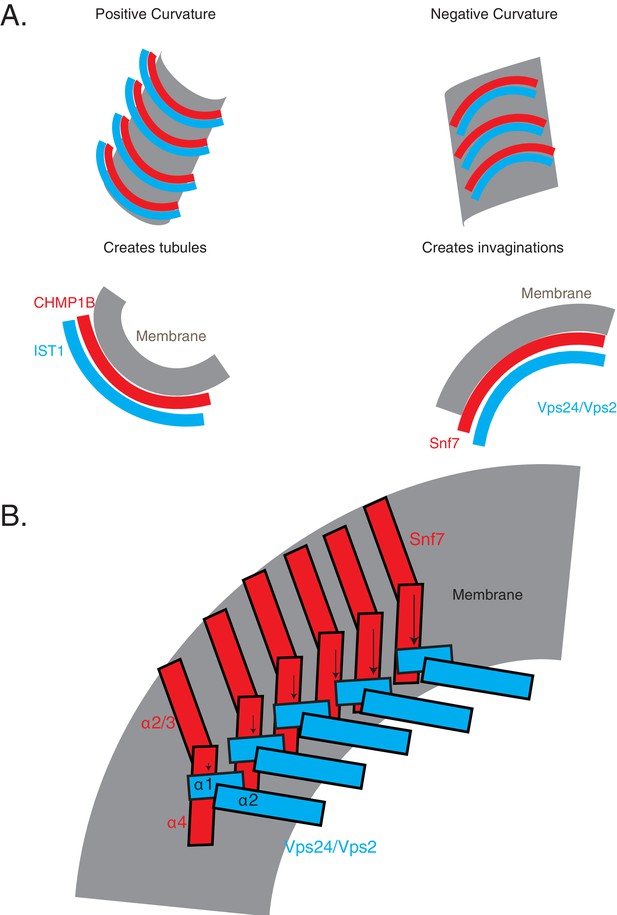
Comparison of inside-out and outside-in tubulation properties of ESCRT-III proteins.
(A) Hypothetical model of how the Snf7/Vps24/Vps2 polymers engage membranes (right), compared to the structure-based model of CHMP1B-IST1 assembly (left). (B) Figure illustrates a hypothetical model of how the helices of Snf7 and its partners may be arranged on the membrane. Note that only helices 1–3 are shown in this model. Arrows point to the different positions of helices along the polymer.
Additional files
-
Supplementary file 1
Key resources table.
- https://doi.org/10.7554/eLife.46207.033
-
Transparent reporting form
- https://doi.org/10.7554/eLife.46207.034