A dedicated diribonuclease resolves a key bottleneck for the terminal step of RNA degradation
Figures
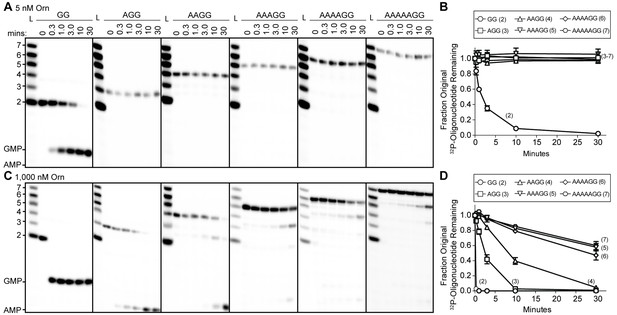
Orn has a stark preference for diribonucleotide cleavage in vitro.
RNA nucleotides two to seven residues in length (1 μM, containing the corresponding 32P-labeled RNA tracer) were each subjected to cleavage over time with 5 nM (A, B) or 1000 nM OrnVc (C, D). Aliquots of each reaction were stopped at indicated times (min), and assessed by denaturing 20% PAGE (A, C). Quantification of the intensities of bands corresponding to the amount of uncleaved initial oligonucleotide over time are plotted as the average and SD of three independent experiments (B, D).
-
Figure 1—source data 1
Numerical data for Figure 1B and D.
- https://cdn.elifesciences.org/articles/46313/elife-46313-fig1-data1-v5.xlsx
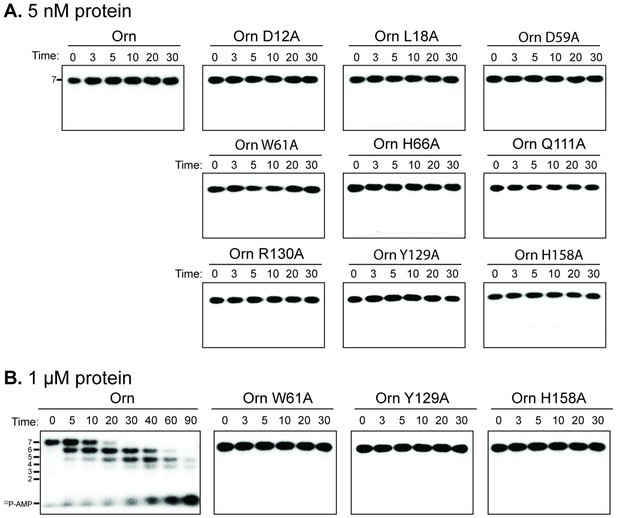
Catalytic residues of OrnVc are required for degradation for 32P-AAAAAGG in vitro.
Degradation of 32P-AAAAAGG by purified indicated His10-MBP-OrnVc variants with 5 nM (A) or 1 µM (B). Samples were stopped at indicated time (min) and analyzed by denaturing 20% PAGE. Representative gel images of two independent assays are shown.
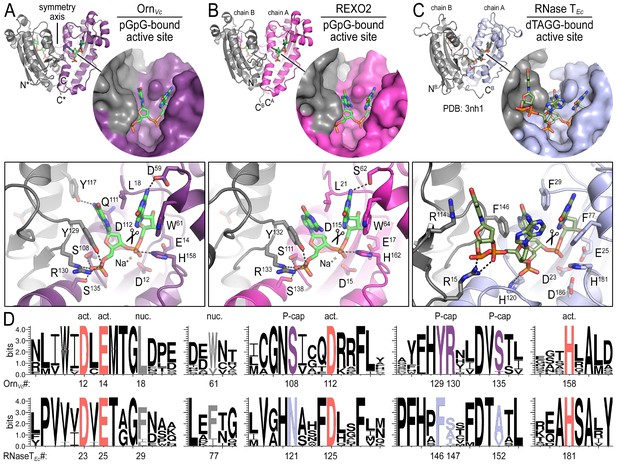
Structures reveal Orn’s conserved substrate preference for diribonucleotides.
Crystal structures of pGpG-bound V. cholerae Orn (A) and human REXO2 (B) are shown in comparison to E. coli RNase T bound to substrate (PDB 3nh1; Hsiao et al., 2011) (C), another DnaQ-fold 3’−5’ exoribonuclease with a DEDD(h) active site motif. The top panels show ribbon representations of the dimeric enzymes. The insets are surface representations of the enzymes’ active sites shown in similar orientations. The bottom panel describes the active site residues involved in RNA binding and catalysis. Residue numbering for REXO2 refers to its cytosolic isoform lacking the mitochondria-targeting pre-sequence. The sequence logos in (D) were constructed based on multi-sequence alignments of Orn and RNase T orthologs. Overall sequence identity ranges from 43 to 70% for Orn and 46 to 69% for RNase T. Sequence identifiers are provided in Figure 2—source data 2. Sequence logos were plotted using WebLogo (Crooks et al., 2004). Conserved residues of the active site’s DEDD motif (‘act.’; red), for ribonucleotide base binding (‘nuc.’; gray), and of the phosphate cap (‘P-cap’; purple) are highlighted.
-
Figure 2—source data 1
Data collection and refinement statistics.
- https://cdn.elifesciences.org/articles/46313/elife-46313-fig2-data1-v5.xlsx
-
Figure 2—source data 2
Sequences used to calculate surface conservation and generate Weblogos.
- https://cdn.elifesciences.org/articles/46313/elife-46313-fig2-data2-v5.xlsx
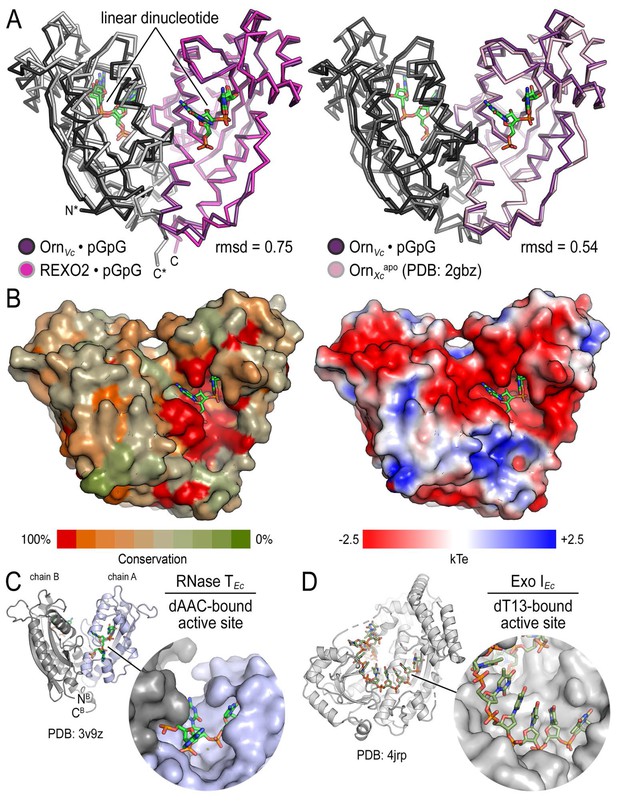
Structural comparison of Orn orthologs, RNase T and Exo I.
(A) Superposition of OrnVc with REXO2 (left) and the substrate-free state of Orn from Xanthomonas campestris (PDB 2gbz; Chin et al., 2006) (right). Rmsd values are reported for the alignment of a monomer. (B) Surface properties of Orn enzymes. An alignment of Orn orthologs was used to map conservation scores onto the solvent accessible surface of OrnVc (left). High degree of conservation overlaps with the acidic active site observed in the electrostatic potential map calculated with the APBS software (Baker et al., 2001) and based on OrnVc (right). (C) Active-site view of E. coli RNase T bound to dAAC (PDB 3v9z; Hsiao et al., 2012). (D) Active-site view of E. coli Exo I bound to dT13 (PDB 4jrp; Korada et al., 2013).
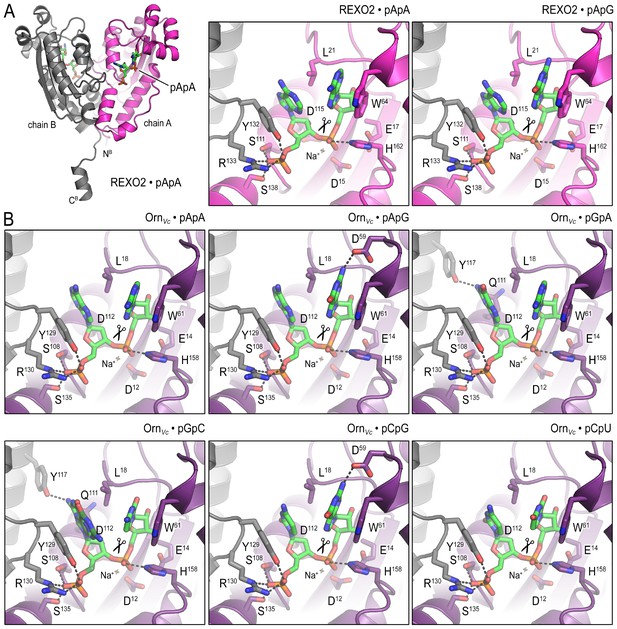
Structural comparison of OrnVc and REXO2 bound to diverse diribonucleotides.
(A) Crystal structures of REXO2 bound to pApA and pApG. The structural overview (left) shows a C-terminal helix that is unique to Orn orthologs in higher eukaryotes and in the current crystal forms is stabilized by crystal packing contacts. (B) Crystal structures of OrnVc bound to di-purine (pApA, pApG, pGpA), purine-pyrimidine (pGpC, pCpG), and di-pyrimidine (pCpU) substrates. Views are identical to those shown in Figure 2 for the REXO2:pGpG and Orn:pGpG complexes.
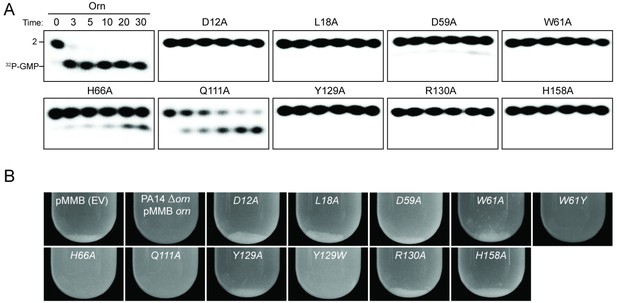
Functional importance of active-site and phosphate-cap residues for Orn function.
(A) In vitro enzyme activity. Degradation of 32P-pGpG (1 μM) by purified wild-type OrnVc or variants with alanine substitutions (5 nM) at the indicated sites was assessed. Samples were stopped at the indicated times (min) and analyzed by denaturing 20% PAGE. Representative gel images are shown with the indicated RNA size. Figure 3—figure supplement 4 shows the graphs of the means and SD of three independent experiments. (B) In vivo activity of alanine substituted ornVc alleles to complement P. aeruginosa Δorn. Overnight cultures of the indicated strains were allowed to stand for 10 min without agitation to allow bacterial aggregates to sediment. Representative images of the cultures of triplicated assays are shown.
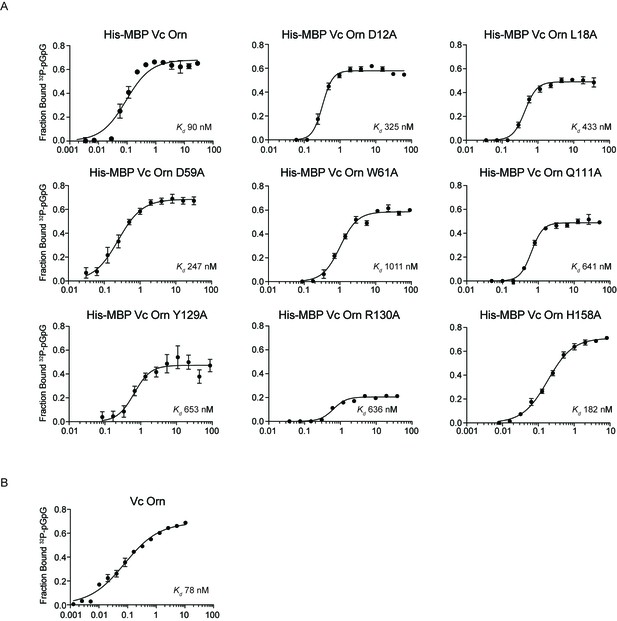
Contribution of catalytic residues and His10-MBP tag to binding interaction between OrnVc and pGpG.
The dissociation constants (Kd) of indicated purified (A) His10-MBP-OrnVc variants and (B) untagged OrnVc to pGpG were measured by DRaCALA. Fraction bound from data was plotted and determined by nonlinear regression. All data shown represent average and SD of three independent experiments.
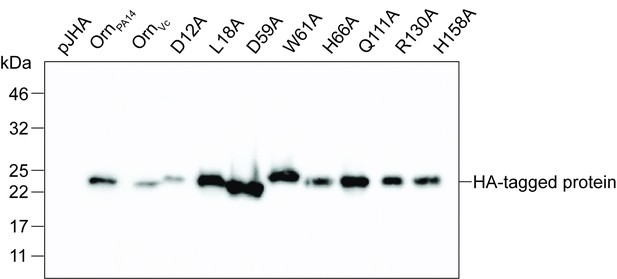
Immunoblot analysis of Orn in P. aeruginosa ∆orn expressing indicated orn alleles.
Proteins in whole cell lysates of strains expressing the indicated HA-tagged orn allele were separated by 12% SDS-PAGE and probed with anti-HA antibodies. All images were performed in duplicate independently. A representative image of two independent experiments is shown.
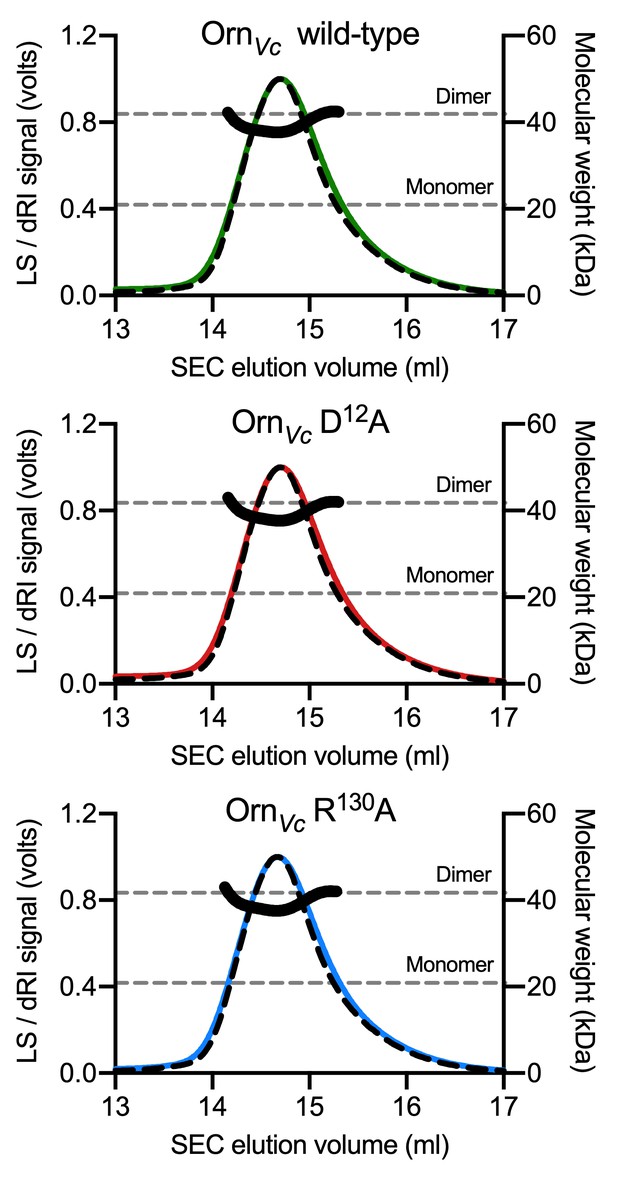
Molecular weight determination indicates that OrnVc variants with point mutations at the phosphate cap and active site remain dimeric.
(A) Absolute molecular weights (black data points across elution peaks are plotted on the right axis; theoretical monomer and dimer molecular weights, horizontal dashed lines) of wild-type OrnVc and point mutants were determined using SEC-MALS (90°-light scattering: colored, solid lines; refractive index signal: black, dashed lines; plotted on the left axis).
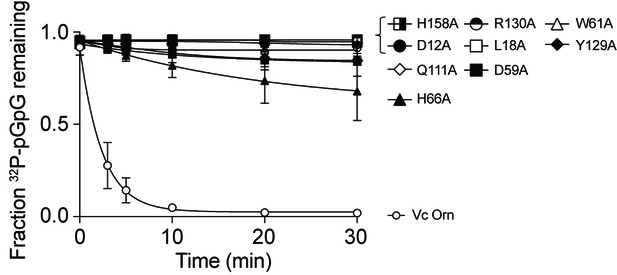
pGpG degradation by purified wild-type OrnVc or variants with indicated alanine substitutions.
1 µM pGpG was treated with 5 nM of the indicated Orn protein and sampled at indicated time points. The graphs show quantification of three independent data sets, of which representative radiographs are shown in Figure 3A.
-
Figure 3—figure supplement 4—source data 1
Numerical data for Figure 3—figure supplement 4.
- https://cdn.elifesciences.org/articles/46313/elife-46313-fig3-figsupp4-data1-v5.xlsx
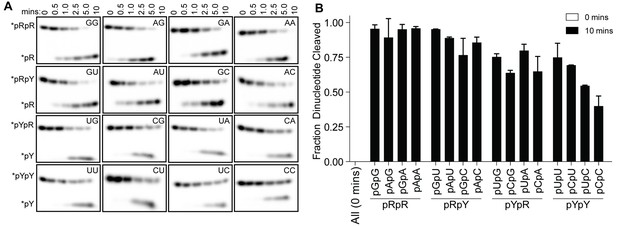
OrnVc cleaves all diribonucleotides.
(A) OrnVc (5 nM) was incubated with di-purine (pRpR), purine-pyrimidine (pRpY or pYpR), or di-pyrimidine (pYpY) diribonucleotides (1 μM) containing the corresponding 32P-labeled RNA tracer. Aliquots of each reaction were stopped at the indicated times (min) and assessed by denaturing 20% PAGE. (B) Quantification of the intensities of bands corresponding to the amount of diribonucleotide cleaved at the 10 min time point. Results are the average and SD of duplicate independent experiments. Orn cleaves all diribonucleotides to nucleoside monosphosphates, albeit to varying extents. Diribonucleotides consisting of two purines (pRpR) were hydrolyzed most efficiently, with over 90% of starting RNAs processed by 10 min. A majority (>75%) of diribonucleotides with a 5' purine (pRpY) were also processed by the 10 min endpoint. However, diribonucleotides with a 5' pyrimidines exhibited moderately reduced levels of cleavage. Di-pyrimidine (pYpY) substrates, and in particular pUpC and pCpC, showed the slowest turnover from the substrates tested. These results demonstrate that while all diribonucleotides are acceptable substrates for Orn, the enzyme is likely to exhibit moderate preferences for diribonucleotides that contain a 5' purine.
-
Figure 4—source data 1
Numerical data for Figure 4B.
- https://cdn.elifesciences.org/articles/46313/elife-46313-fig4-data1-v5.xlsx
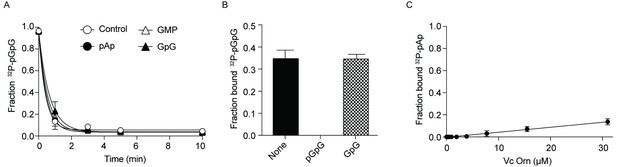
Effect of GpG and pAp on Orn activity.
(A) Cleavage of 130 nM 32P-pGpG by 5 nM Orn in the absence or presence of unlabeled pAp, GpG or GMP (100 µM). (B) Fraction bound of 32P-pGpG to 200 nM Orn in presence of no competitor, 100 µM pGpG or 100 µM GpG. (C) Fraction bound of 32P-pAp to different concentration of OrnVc was determined by DRaCALA. Kd could not be measured as 32P-pAp binding to Orn was not saturated. (B) (C) All data shown represent duplicate-independent experiments.
-
Figure 4—figure supplement 1—source data 1
Numerical data for Figure 4—figure supplement 1.
- https://cdn.elifesciences.org/articles/46313/elife-46313-fig4-figsupp1-data1-v5.xlsx
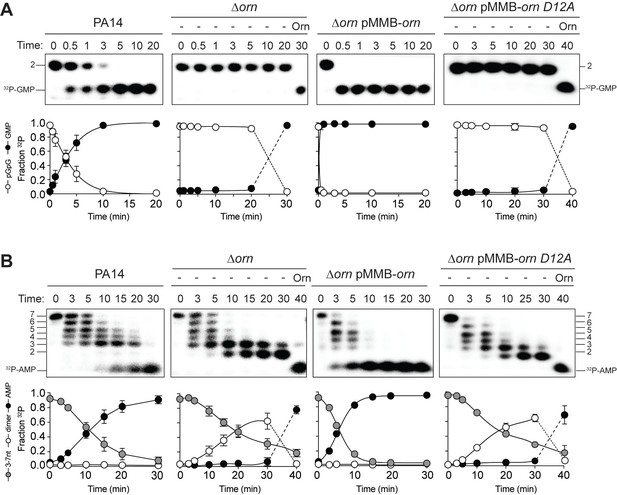
Orn acts as a diribonuclease in cell lysates.
Degradation of 32P-GG (A) and 32P-AAAAAGG (B) by whole cell lysates of wild-type, orn mutant, orn mutant complemented with ornVc, or ornVc D12A. For ∆orn and ∆orn complemented with ornVc D12A,100 nM of purified OrnVc was added at 30 min time point and incubated for an additional 10 min. Samples were stopped at the indicated time and analyzed by 20% denaturing PAGE. Representative gel images of triplicated assays are shown with the indicated RNA size. Graphs show quantitation of triplicate data for indicated RNA species over time.
-
Figure 5—source data 1
Numerical data for Figure 5.
- https://cdn.elifesciences.org/articles/46313/elife-46313-fig5-data1-v5.xlsx
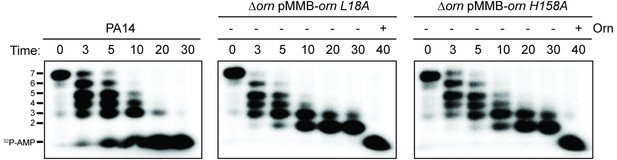
Catalytic residues of OrnVc are required for degradation for 32P-AAAAAGG in whole-cell lysates.
Degradation of 32P-AAAAAGG by whole-cell lysates of the indicated strain (PA14, ∆orn mutant complemented with pMMB-ornVc L18A or pMMB-ornVc H158A. For ∆orn expressing ornVc L18A or ornVc H158A, 100 nM of purified, wild-type OrnVc protein was added at the 30 min time point and reactions were stopped 10 min thereafter. Samples were stopped at indicated time and analyzed by denaturing 20% PAGE. Representative gel images of two independent experiments are shown.
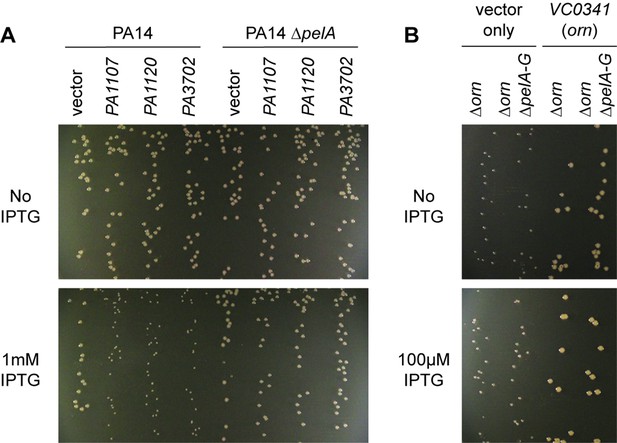
Small-colony phenotype of ∆orn is independent of c-di-GMP signaling.
Bacterial cultures were diluted and dripped on LB agar plates with the indicated concentration of IPTG. After overnight incubation, representative images of the plates were taken and shown for (A) PA14 and PA14 ∆pelA harboring pMMB vector with the indicated diguanylate cyclase gene and (B) PA14 ∆orn and PA14 ∆orn ∆pelA-G with pMMB and pMMB-orn. Experiments were performed in triplicate.
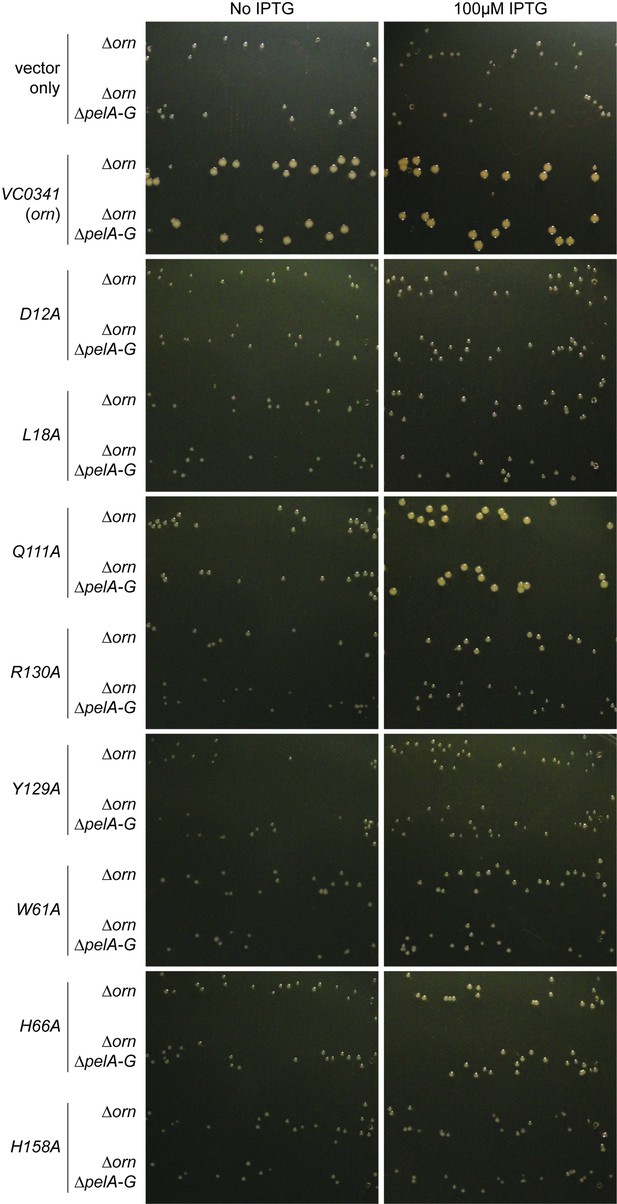
Small-colony phenotype of ∆orn is complemented by functional ornVc alleles.
Bacterial cultures were diluted, 10 µL was spotted on LB agar plates with the indicated concentration of IPTG and drip to form parallel lines of bacteria. After overnight incubation, representative images of the plates were taken of PA14 ∆orn and PA14 ∆orn ∆pelA-G with pMMB alone or containing the indicated allele of ornVc. Experiments were performed in triplicate and one representative image of the plates is shown.
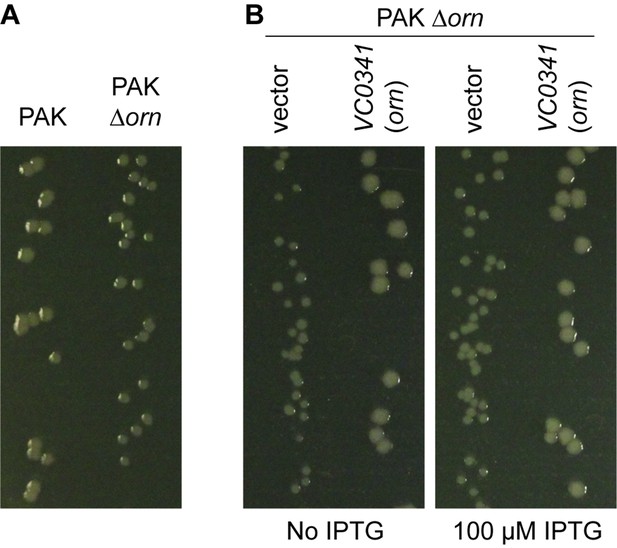
P. aeruginosa PAK ∆orn mutants show a small-colony phenotype.
Indicated bacterial strains were diluted and dripped on (A) LB agar plates or (B) LB agar plates containing 50 µg/mL carbenicillin with indicated concentration of IPTG and incubated overnight at 37°C. All experiments were performed in triplicate and one representative image of the plates is shown.
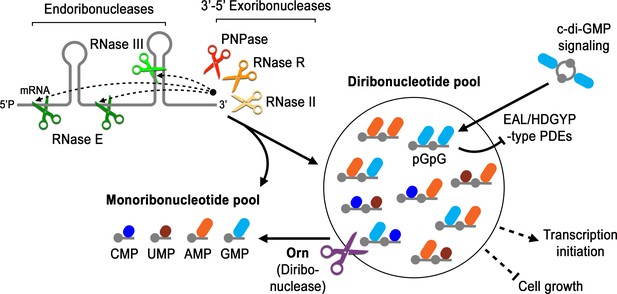
Model for Orn’s cellular function as a diribonuclease.
RNA degradation is initiated by fragmentation via endoribonucleases (e.g. RNase E and RNase III) that cleave unstructured or structured RNA sequences. RNA fragments are processed further at their 3’ termini by 3’−5’ exoribonucleases (e.g. PNPase, RNase R, and RNase II). Their combined activity produces mononucleotides and various terminal diribonucleotides from the original RNA fragments. The pGpG (GG) linear diribonucleotide is also produced by linearization of c-di-GMP by specific phosphodiesterases, EAL- and HD-GYP-domain-containing enzymes, which terminate c-di-GMP signaling. In Pseudomonas aeruginosa and likely other organisms that rely on Orn for growth, Orn is the only diribonuclease that cleaves diribonucleotides to mononucleotides. In the absence of Orn, diribonucleotides accumulate with a drastic impact on cellular physiology, ranging from transcriptional changes, small-colony phenotypes and growth arrest, depending on the organism. Orn is also unique because it acts as the second phosphodiesterase in the degradation of c-di-GMP by clearing the pGpG intermediate. In an orn mutant, c-di-GMP levels are elevated through feedback inhibition of the c-di-GMP-degrading phosphodiesterases by pGpG, leading to the associated biofilm phenotypes.
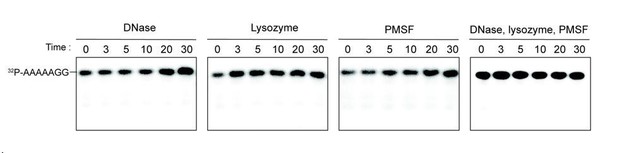
Additives to cell lystaes do not contribute to cleavage of 32P-AAAAAGG oligoribonucleotide.
10 µg/ml DNase, 25 µg/ml lysozyme, 1 mM PMSF, and the combination of all three additives were test for cleavage of 32P-AAAAAGG. The samples were stopped at indicated times and analyzed by 20% Urea gel. All data shown represent duplicate independent experiments.
Tables
Quantitative measurement of length-dependent oligoribonucleotide affinities.
Substratea | Dissociation constant Kd (nM)b |
---|---|
GG | 90 -/+9 |
AGG | 630 -/+80 |
AAGG | 890 -/+90 |
AAAGG | 2,560 -/+170 |
AAAAGG | 3,830 -/+370 |
AAAAAGG | 3,750 -/+530 |
-
a: RNAs were labeled with 32P at their 5’ end. b: Affinities were measured using an established binding assay for radiolabeled RNAs (Roelofs et al., 2011; Patel et al., 2014).
Reagent type or resource | Designation | Source or reference | Identifiers | Additional information |
---|---|---|---|---|
Strain (P. aeruginosa) | PA14 | Rahme et al., 1995; PMID 7604262 | ||
Strain (P. aeruginosa) | PA14 ∆pelA | Lee et al., 2007; PMID: 17824927 | ||
Strain (P. aeruginosa) | PA14 ∆orn | Orr et al., 2015; PMID: 26305945 | ||
Strain (P. aeruginosa) | PA14 ∆orn ∆pelA-G | Orr et al., 2015; PMID: 26305945 | ||
Strain (P. aeruginosa) | PAK | Bradley, 1974; PMID: 4206974 | ||
Strain (P. aeruginosa) | PAK ∆orn | This study | Generated using pEX-Gn-∆orn (PAK) | |
Strain (E. coli) | XL10-Gold | Agilent | ||
Strain (E. coli) | Stellar cells | Takara/Clontech | ||
Strain (E. coli) | BL21(DE3) | New England Biolabs | ||
Strain (E. coli) | NEB T7Iq | New England Biolabs | ||
Genetic reagent (plasmid) | pET28-His6-SUMO-OrnVc | This study | cloned from custom DNA fragment (see below) for purification of His6-SUMO-OrnVc, OrnVc | |
Genetic reagent (plasmid) | pET28-His6-SUMO-Rexo2 | This study | cloned from custom DNA fragment (see below) for purification of His6-SUMO-Rexo2, Rexo2 | |
Genetic reagent (plasmid) | pDONR221-VC0341 (ornVc) | Rolfs et al., 2008; PMID: 18337508 | ||
Genetic reagent (plasmid) | pDONR221-ornVc D12A | This study | site-directed mutagenesis to introduce alanine at D12 position | |
Genetic reagent (plasmid) | pDONR221-ornVc L18A | This study | site-directed mutagenesis to introduce alanine at L18 position | |
Genetic reagent (plasmid) | pDONR221-ornVc D59A | This study | site-directed mutagenesis to introduce alanine at D59 position | |
Genetic reagent (plasmid) | pDONR221-ornVc Q111A | This study | site-directed mutagenesis to introduce alanine at Q111 position | |
Genetic reagent (plasmid) | pDONR221-ornVc R130A | This study | site-directed mutagenesis to introduce alanine at R130 position | |
Genetic reagent (plasmid) | pDONR221-ornVc Y129A | This study | site-directed mutagenesis to introduce alanine at Y129 position | |
Genetic reagent (plasmid) | pDONR221-ornVc Y129W | This study | site-directed mutagenesis to introduce tryptophan at Y129 position | |
Genetic reagent (plasmid) | pDONR221-ornVc W61A | This study | site-directed mutagenesis to introduce alanine at W61 position | |
Genetic reagent (plasmid) | pDONR221-ornVc W61Y | This study | site-directed mutagenesis to introduce tyrosine at W61 position | |
Genetic reagent (plasmid) | pDONR221-ornVc H66A | This study | site-directed mutagenesis to introduce alanine at H66 position | |
Genetic reagent (plasmid) | pDONR221-ornVc H158A | This study | site-directed mutagenesis to introduce alanine at H158 position | |
Genetic reagent (plasmid) | pVL847-ornVc | Orr et al., 2015; PMID: 26305945 | OrnVc from pDONR cloned into pVL847 for purification of His-MBP-OrnVc, OnrVc | |
Genetic reagent (plasmid) | pVL847-ornVc D12A | This study | D12A from pDONR cloned into pVL847 for purification of His-MBP-OrnVc D12A, OrnVc D12A | |
Genetic reagent (plasmid) | pVL847-ornVc L18A | This study | L18A from pDONR cloned into pVL847 for purification of His-MBP-OrnVc L18A, OrnVc L18A | |
Genetic reagent (plasmid) | pVL847-ornVc D59A | This study | L18A from pDONR cloned into pVL847 for purification of His-MBP-OrnVc D59A, OrnVc D59A | |
Genetic reagent (plasmid) | pVL847-ornVc Q111A | This study | Q111A from pDONR cloned into pVL847 for purification of His-MBP-OrnVc Q111A, OrnVc Q111A | |
Genetic reagent (plasmid) | pVL847-ornVc R130A | This study | R130A from pDONR cloned into pVL847 for purification of His-MBP-OrnVc R130A, OrnVc R130A | |
Genetic reagent (plasmid) | pVL847-ornVc Y129A | This study | Y129A from pDONR cloned into pVL847 for purification of His-MBP-OrnVc Y129A, OrnVc Y129A | |
Genetic reagent (plasmid) | pVL847-ornVc W61A | This study | W61A from pDONR cloned into pVL847 for purification of His-MBP-OrnVc W61A, OrnVc W61A | |
Genetic reagent (plasmid) | pVL847-ornVc H66A | This study | H66A from pDONR cloned into pVL847 for purification of His-MBP-OrnVc H66A, OrnVc H66A | |
Genetic reagent (plasmid) | pVL847-ornVc H158A | This study | H158A from pDONR cloned into pVL847 for purification of His-MBP-OrnVc H158A, OrnVc H158A | |
Genetic reagent (plasmid) | pMMB-Gn | Fürste et al., 1986; PMID: 3549457 | ||
Genetic reagent (plasmid) | pMMB-Gn-PA1107 | Kulasakara et al., 2006; PMID: 16477007 | ||
Genetic reagent (plasmid) | pMMB-Gn-PA1120 | Kulasakara et al., 2006; PMID: 16477007 | ||
Genetic reagent (plasmid) | pMMB-Gn-PA3702 (wspR) | Kulasakara et al., 2006; PMID: 16477007 | ||
Genetic reagent (plasmid) | pMMB-Ap | Fürste et al., 1986; PMID: 3549457 | ||
Genetic reagent (plasmid) | pMMB-Ap-VC0341 (ornVc) | This study | Orn from pDONR cloned into pMMB for expressing in P. aeruginosa | |
Genetic reagent (plasmid) | pMMB-Ap-ornVc D12A | This study | D12A from pDONR cloned into pMMB for expressing in P. aeruginosa | |
Genetic reagent (plasmid) | pMMB-Ap-ornVc L18A | This study | L18A from pDONR cloned into pMMB for expressing in P. aeruginosa | |
Genetic reagent (plasmid) | pMMB-Ap-ornVc Q111A | This study | Q111A from pDONR cloned into pMMB for expressing in P. aeruginosa | |
Genetic reagent (plasmid) | pMMB-Ap-ornVc R130A | This study | R130A from pDONR cloned into pMMB for expressing in P. aeruginosa | |
Genetic reagent (plasmid) | pMMB-Ap-ornVc Y129A | This study | Y129A from pDONR cloned into pMMB for expressing in P. aeruginosa | |
Genetic reagent (plasmid) | pMMB-Ap-ornVc Y129W | This study | Y129W from pDONR cloned into pMMB for expressing in P. aeruginosa | |
Genetic reagent (plasmid) | pMMB-Ap-ornVc W61A | This study | W61A from pDONR cloned into pMMB for expressing in P. aeruginosa | |
Genetic reagent (plasmid) | pMMB-Ap-ornVc W61Y | This study | W61Y from pDONR cloned into pMMB for expressing in P. aeruginosa | |
Genetic reagent (plasmid) | pMMB-Ap-ornVc H66A | This study | H66A from pDONR cloned into pMMB for expressing in P. aeruginosa | |
Genetic reagent (plasmid) | pMMB-Ap-ornVc H158A | This study | H158A from pDONR cloned into pMMB for expressing in P. aeruginosa | |
Genetic reagent (plasmid) | pEX-Gn-∆orn (PAK) | This study | ||
Genetic reagent (plasmid) | pJHA-Gn | This study | ||
Genetic reagent (plasmid) | pJHA-Gn-ornPa | This study | OrnPA (Orr, et al, PNAS 2015) cloned into pJHA for expressing in P. aeruginosa | |
Genetic reagent (plasmid) | pJHA-Gn-ornVc | This study | Orn from pDONR cloned into pJHA for expressing in P. aeruginosa | |
Genetic reagent (plasmid) | pJHA-Gn-ornVc D12A | This study | D12A from pDONR cloned into pJHA for expressing in P. aeruginosa | |
Genetic reagent (plasmid) | pJHA-Gn-ornVc L18A | This study | L18A from pDONR cloned into pJHA for expressing in P. aeruginosa | |
Genetic reagent (plasmid) | pJHA-Gn-ornVc D59A | This study | Q111A from pDONR cloned into pJHA for expressing in P. aeruginosa | |
Genetic reagent (plasmid) | pJHA-Gn-ornVc W61A | This study | R130A from pDONR cloned into pJHA for expressing in P. aeruginosa | |
Genetic reagent (plasmid) | pJHA-Gn-ornVc H66A | This study | Y129A from pDONR cloned into pJHA for expressing in P. aeruginosa | |
Genetic reagent (plasmid) | pJHA-Gn-ornVc Q111A | This study | W61A from pDONR cloned into pJHA for expressing in P. aeruginosa | |
Genetic reagent (plasmid) | pJHA-Gn-ornVc R130A | This study | H66A from pDONR cloned into pJHA for expressing in P. aeruginosa | |
Genetic reagent (plasmid) | pJHA-Gn-ornVc H158A | This study | H158A from pDONR cloned into pJHA for expressing in P. aeruginosa | |
Antibody | Mouse anti-HA monoclonal antibody | Sigma; catalog number H9658 | 1:5000 | |
Antibody | Goat Anti-Mouse IgG-HRP conjugate | Sigma; catalog number A9917 | 1:5000 | |
DNA fragment | 5'-GGATCCATGGCAGCCGGTGAAAG CATGGCACAGCGTATGGTTTGGGTT GATCTGGAAATGACCGGTCTGGATA TTGAAAAAGATCAGATTATTGAAATG GCCTGCCTGATTACCGATAGCGATCT GAATATTCTGGCAGAAGGTCCGAAT CTGATTATCAAACAGCCGGATGAACT GCTGGATAGCATGAGCGATTGGTGT AAAGAACATCATGGTAAAAGCGGTCT GACCAAAGCAGTTAAAGAAAGCACCA TTACACTGCAGCAGGCCGAATATGAAT TTCTGAGCTTTGTTCGTCAGCAGACC CCTCCGGGTCTGTGTCCGCTGGCAGG TAATAGCGTTCATGAAGATAAAAAGTT TCTGGATAAGTATATGCCGCAGTTTAT GAAGCATCTGCATTATCGCATTATTGAT GTGAGCACCGTTAAAGAACTGTGTCGT CGTTGGTATCCGGAAGAATATGAGTTT GCACCGAAAAAAGCAGCAAGCCATCGT GCACTGGATGATATTAGCGAAAGCATC AAAGAGCTGCAGTTTTATCGCAACAAC ATCTTCAAAAAGAAAATCGACGAGAAAA AACGCAAAATCATCGAAAACGGCGAAAA CGAAAAAACCGTTAGCTAAGCGGCCGC-3' | GeneArt | custom DNA fragment for REXO2 that is codon-optimized for E. coli | |
DNA fragment | 5'-GGATCCATGAGCTTTAGCGATCAGAAT CTGATTTGGATTGATCTGGAAATGACCG GTCTGGACCCGGAAATGCATAAAATCAT TGAAATGGCAACCATCGTGACCGATAGCG AACTGAATATTCTGGCAGAAGGTCCGGTT ATTGCAATTCATCAGCCGGAAAGCGAACT GGCAAAAATGGATGAATGGTGTACCACCA CCCATACCGCAAGCGGTCTGGTTGCACGT GTTCGTCAGAGCCAGGTTAGCGAAGAAGA AGCAATTGATCAGACCCTGGCATTTCTGAA ACAGTGGGTTCCGGAAGGTAAAAGCCCGAT TTGTGGTAATAGCATTGGTCAGGATCGTCG CTTTCTGTATAAACATATGCCTCGTCTGGAA GCCTATTTCCATTATCGTTATATTGATGTGAG CACCATCAAAGAACTGACCCGTCGTTGGCAG CCGGAAGTTCTGAAAGAATTTAGCAAAACCG GTAGCCATCTGGCACTGGATGATATTCGTGA AAGCATTGCAGAGCTGCAGTTTTATCGTAAA GCCGTGTTTAAAATCTAAGCGGCCGC-3' | GeneArt | custom DNA fragment for OrnVc that is codon-optimized for E. coli | |
RNA primer | 5'-GG-3' | Sigma | ||
RNA primer | 5'-AGG-3' | Sigma | ||
RNA primer | 5'-AAGG-3' | Sigma | ||
RNA primer | 5'-AAAGG-3' | Sigma | ||
RNA primer | 5'-AAAAGG-3' | Sigma | ||
RNA primer | 5'-AAAAAGG-3' | Sigma | ||
RNA primer | 5'-pGG-3' | Biolog; catalog number P023-01 | ||
RNA primer | 5'-pAA-3' | Biolog; catalog number P033-01 | ||
RNA primer | 5'-pAG-3' | GE Healthcare Dharmacon | ||
RNA primer | 5'-pGA-3' | GE Healthcare Dharmacon | ||
RNA primer | 5'-pGC-3' | GE Healthcare Dharmacon | ||
RNA primer | 5'-pCG-3' | GE Healthcare Dharmacon | ||
RNA primer | 5'-pCU-3' | GE Healthcare Dharmacon | ||
Software | Prism | GraphPad | ||
Software | XDS | Kabsch, 2010; PMID: 20124693 | Distributed through SBGrid | |
Software | Pointless | Evans, 2006; PMID: 16369096 | Distributed through SBGrid | |
Software | Scala | Evans, 2006; PMID: 16369096 | Distributed through SBGrid | |
Software | Phenix | Adams et al., 2010; PMID: 20124702 | Distributed through SBGrid | |
Software | Coot | Emsley et al., 2010; PMID: 20383002 | Distributed through SBGrid | |
Software | Pymol | Schrödinger | ||
Software | Fujifilm Multi Gauge software v3.0 | Fujifilm |
Additional files
-
Supplementary file 1
Primers.
- https://cdn.elifesciences.org/articles/46313/elife-46313-supp1-v5.xlsx
-
Transparent reporting form
- https://cdn.elifesciences.org/articles/46313/elife-46313-transrepform-v5.pdf