Neuron-specific knockouts indicate the importance of network communication to Drosophila rhythmicity
Figures
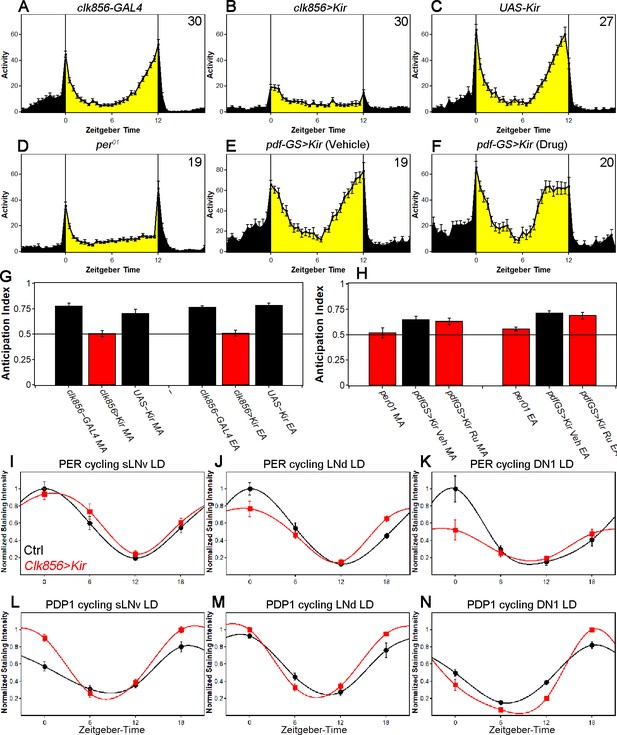
Silencing the clock network has differential effects on behavior and clock protein cycling in LD.
(A–C) Silencing most of the clock network abolishes rhythmic LD behavior. GAL4 control (A) and UAS control (C) show bimodal activity patterns with M anticipation and E anticipation. Silenced flies (B) show no sign of anticipation neither in the morning nor in the evening. Flies show short activity increases at the transitions of day/night and night/day which are considered masking. Values in upper right-hand corner indicate the number of analyzed flies. (D) Behavior of per01 flies in LD 12:12. per01 mutants show behavior similar to clk856>Kir with no M anticipation, reduced E anticipation and short reactions to the light transitions. (E–F) Silencing PDF neurons alters LD behavior. (E) PDF-GS>Kir on Vehicle food does not express Kir. Flies show the typical bimodal activity with M and E anticipation peaks. The M peak is close to lights-on (ZT0) whereas the E peak is close to lights-off (ZT12). (F) Silencing the PDF neurons by adding RU 486 to the food causes an advanced E peak, similar to pdf01 flies. Values indicate the number of analyzed flies. (G) Morning Anticipation (MA) and Evening Anticipation (EA) calculated from A-C. Both controls show values significantly above 0.5 (p<0.0001 for all) indicating prominent anticipation to both peaks. Clk856>Kir flies on the other hand show no signs of anticipation as indicated by anticipation indices indistinguishable from 0.5 (p>0.6937) (H) Morning Anticipation (MA) and Evening Anticipation (EA) calculated from D-E. per01 flies show no MA (p=0.5744), whereas there is a slight increase of activity toward lights-off (p=0.0027). Silencing the PDF neurons did not abolish MA (p=0.0004) and showed a significantly bigger EA compared to per01 flies (p=0.0391). (I-K) PER protein cycling is largely unaffected by neuronal silencing in LD. PER cycling in control brains (black data points ± SEM, pooled GAL4 and UAS, n = 6–8) is highly synchronized with peak levels around ZT0. Silencing the clock network (red data points ± SEM, n = 5–7) had little effect on LD PER rhythms in sLNvs (F(1,49)=0.93, p=0.3391) (I) and LNds (F(1,49)=0.35, p=0.5547) (J). DN1s appear dampened after silencing but 2-way ANOVA shows no difference between control and experimental line (F(1,36)=2.98, p=0.0930) (K). (L-N) PDP1 protein cycling is largely unaffected by neuronal silencing in LD. PDP1 cycling in control brains (black data points ± SEM, pooled GAL4 and UAS, n = 5) is highly synchronized with peak levels around ZT18. Silencing the clock network (red data points ± SEM, n = 5) slightly increased LD PDP1 rhythms in sLNvs (F(1,32)=15.74, p=0.0004) (L) but no effect on LNds (F(1,32)=2.71, p=0.1093) (M) and only a slight effect on DN1s (F(1,32)=6.06, p=0.0194) (N).
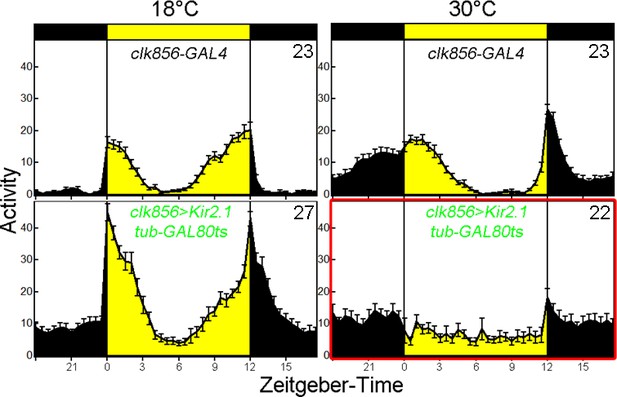
Adult-specific silencing of most clock neurons causes arrhythmic LD behavior.
Control (clk856-GAL4) and experimental (clk856-GAL4 UAS-Kir tub-GAL80ts) flies show bimodal activity at 18 degrees with an M and an E peak of activity. M anticipation is reduced in both cases due to the low temperature. At 30 degrees, control flies (upper panel) show a bimodal activity pattern with an M anticipation peak and an E anticipation peak. The E peak is delayed due to high temperatures. The experimental flies (lower panel) show no M or E peak at 30 degrees.
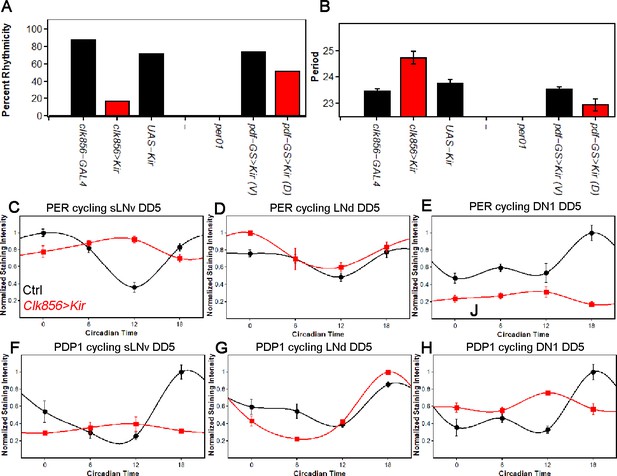
Silencing the clock network strongly affects behavior and molecular rhythms in DD.
(A) Percentage of rhythmic flies in DD. Silencing most of the clock neurons significantly reduces rhythmicity to less than 20 percent, suggesting that clock neuron activity is essential for rhythmic behavior output. None of the per01 flies were rhythmic as expected. Silencing the PDF neurons slightly decreased the level of rhythmicity. Number of flies analyzed same as shown in Figure 1. (B) Free-running period of rhythmic flies from (A). The few rhythmic flies of clk856>Kir show a significantly longer period (F(2,48)=14.355 p<0.001, p<0.01 for UAS and GAL4 control). Adult-specific silencing of the PDF neurons caused a significant period shortening (p=0.0214). Number of flies analyzed same as shown in Figure 1. (C–E) PER protein cycling in DD5. PER cycling in control brains (black data points ± SEM, pooled GAL4 and UAS, n = 8–11) is highly synchronized with peak levels around CT18. Silencing the clock network (red data points ± SEM, n = 5) had variable effects on PER rhythms: The sLNvs (C) dampen strongly upon silencing (p<0.0001). The LNds (D) show no significant differences in cycling amplitude (p=0.8905) and the DN1s strongly dampen (p=0.0195), similar to sLNvs (E). (F–H) PDP1 protein cycling in DD5. PDP1 cycling in control brains (black data points ± SEM, pooled GAL4 and UAS, n = 5) is highly synchronized with peak levels around CT18. Silencing the clock network (red data points ± SEM, n = 5) had similar effects as observed in PER rhythms: The sLNvs (F) dampen strongly upon silencing (p=0.0006). The LNds (G) show a higher cycling amplitude than control brains (p<0.0001) and the DN1s strongly dampen (p=0.0013) and appear to have a phase-advanced PDP1 peak (H).
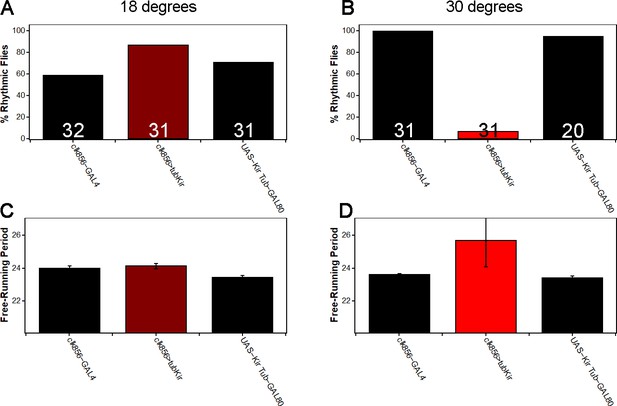
Adult-specific silencing of most clock neurons causes arrhythmic DD behavior.
(A) At 18 degrees we observe no decrease in rhythmicity in clk856>tubKir (dark red) compared to control (black) flies as expected. Increasing the temperature to 30 degrees (B) activates GAL4 and hence silences the neurons. Clk856>tubKir (red) flies get arrhythmic, whereas both controls (black) show high levels of rhythmicity. We observed no effect on free-running period at 18 degrees (C) but experimental flies showed the tendency toward a long period at 30 degrees (D), similar to clk856>Kir (Figure 2B). Values indicate the number of investigated flies.
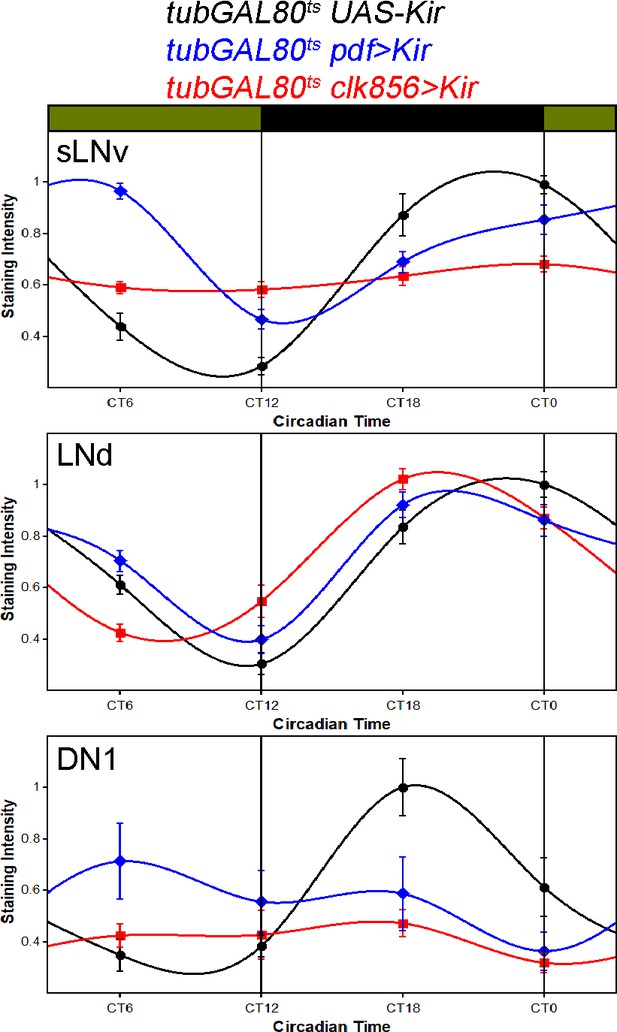
Adult-specific silencing reproduces PER cycling profiles.
Flies were raised at 18 degrees and entrained at 30 degrees to allow network silencing. Flies were collected in 6 hr intervals in the fifth day of constant darkness. Control flies (tub-GAL80ts UAS-Kir, black data points ± SEM, n = 10) show robust cycling with peak expression around ZT0 in LNds and sLNvs and around ZT18 in DN1s. Silencing the PDF neurons (pdf-GAL4 UAS-Kir tubGAL80ts, blue data points ± SEM, n = 10) only slightly affected PER cycling in the sLNvs, which retain robust PER rhythms. Similarly, the LNds remain rhythmic, but the DN1 rhythms get more irregular. Silencing the entire network neurons (clk856-GAL4 UAS-Kir tubGAL80ts, red data points ± SEM, n = 20) causes the sLNvs to get arrhythmic. The LNds show no reduction in cycling amplitude and only a slight shift in protein cycling. The DN1s show a reduction of PER cycling amplitude.
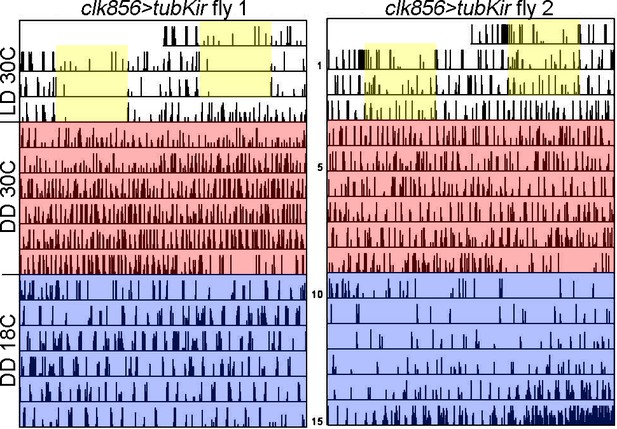
Removing neuronal silencing after 6 days in DD does not re-establish rhythmic behavior.
Actograms of individual flies recorded at 30 degrees for 3 days in LD 12:12 (indicated by yellow boxes). Flies were then transferred into constant darkness at 30 degrees (red area). Flies show no sign of rhythm due to the silencing of the network. We lowered the temperature to 18 degrees (blue area) to stop neuronal silencing. None of the flies re-established rhythmic behavior.
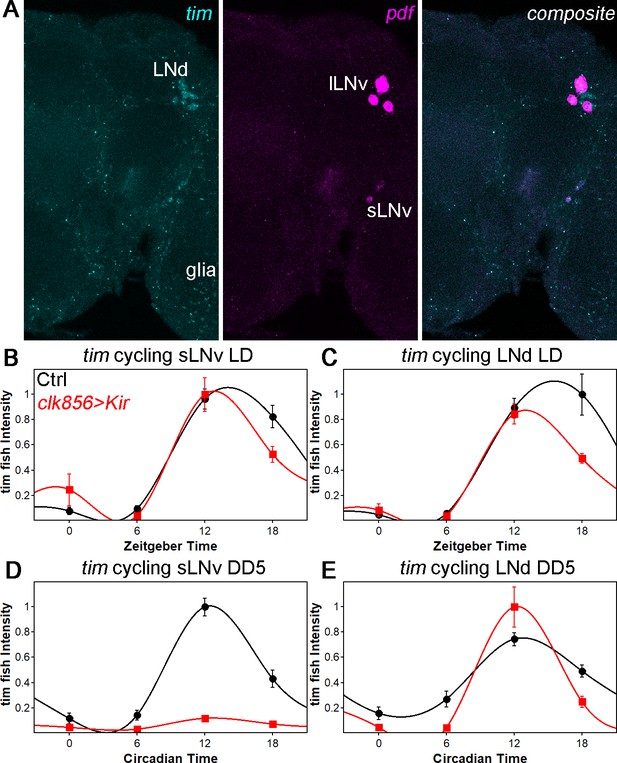
tim mRNA cycling in sLNvs and LNds shows similar trends as protein cycling as observed by FISH.
(A) Representative brain showing tim (left panel), pdf (middle panel) and a composite of both channel (right panel). Tim probes label all clock neurons and glial cells, whereas the pdf probes only label sLNvs and lLNvs. (B–C) tim mRNA cycling in LD 12:12 in sLNvs (B) and LNds (C). Control flies (black data points ± SEM, pooled GAL4 and UAS, n = 10) show high amplitude cycling with peak levels at the beginning of the night. Silencing the clock network (red data points ± SEM, n = 5) has only little effect on cycling amplitude or timing in LD. (D–E) tim mRNA cycling in DD5 in sLNvs (D) and LNds (E). Control flies (black data points ± SEM, pooled GAL4 and UAS, n = 10) show high amplitude cycling with peak levels at the beginning of the night. Silencing the sLNvs (D) leads to an overall reduction of tim mRNA levels and a loss of rhythmicity. In the LNds (E) silencing did not decrease cycling amplitude or shifted peak mRNA expression.
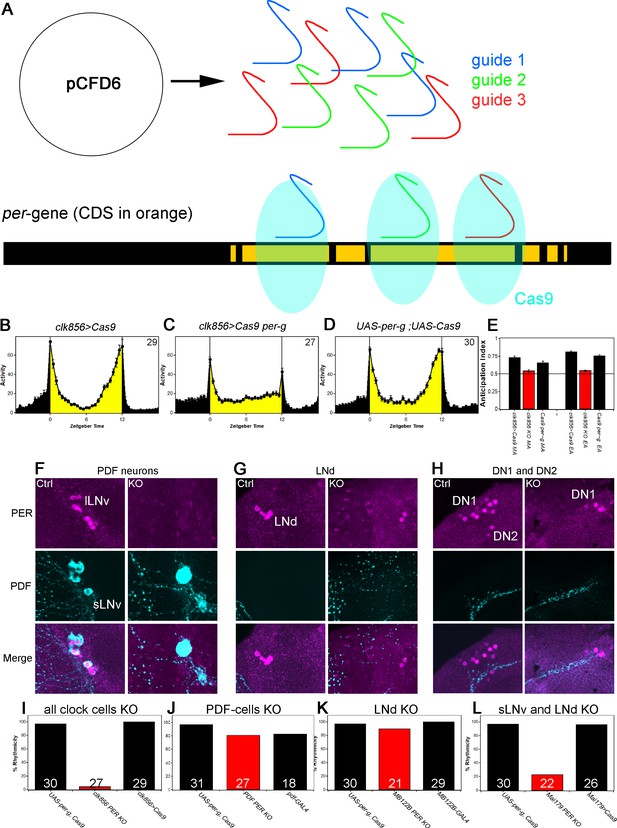
A clock in the LNds or the sLNvs can drive rhythmic behavior.
(A) Schematic model of cell-specific knockout (KO) strategy. We generated a UAS-per-g line using the pCFD6 vector, allowing us to express three guides under the control of one UAS promoter (after Port and Bullock, 2016). We cloned three guides targeting the per CDS with guide one targeting the second exon shared by all transcripts and guides 2 and 3 targeting the 4th commonly shared exon. The guides will recruit the Cas9 protein and induce double-strand breaks and thereby cause mutations which lead to a non-functional protein. (B–E) Behavior of perKO using clk856-GAL4 in LD 12:12 reproduces per01 phenotype. Flies expressing Cas9 in the majority of the clock neurons (B) and flies with both UAS-constructs (D) show bimodal activity with an M anticipation peak around lights-on and an E anticipation peak around lights-off. KO of per using clk856-GAL4 (C) abolishes M and E anticipation similar to per01 flies. (E) Morning and Evening Anticipation are significantly reduced in the clk856-GAL4 mediated Knockout (MA: F(2,85)=18.6895, p<0.0001, p<0.01 for both controls. EA: F(2,85)=114.6644, p<0.0001, p<0.01 for both controls). Most importantly, both indices of the knockout strain are indistinguishable from per01 flies (MA: p=0.6667, EA: p=0.4884) (F–H) Immunocytochemistry of Control (clk856>Cas9) and KO (clk856>Cas9, perG) staining against PER (magenta) and PDF (cyan). Control flies show PER staining in both, sLNvs and lLNvs, whereas there is no detectable PER signal in the PDF cells in the KO strain (E). Similarly, we see six LNds in the control and two LNds in the experimental flies, showing that some neurons can escape (F) The number of PER+ DN1s is strongly reduced in the KO strain and we do not detect PER in the DN2 neurons (G). (I–L) A clock in LNd or PDF neurons is necessary for rhythmic behavior. Clk856-GAL4 mediated KO reduces rhythmicity to less than 10% (H). KO in PDF neurons (pdf-GAL4) (I) or in the LNds (MB122B-split-GAL4) (J) had no effect on rhythmicity. KO in both places (Mai179-GAL4) significantly decreases rhythmicity (K).
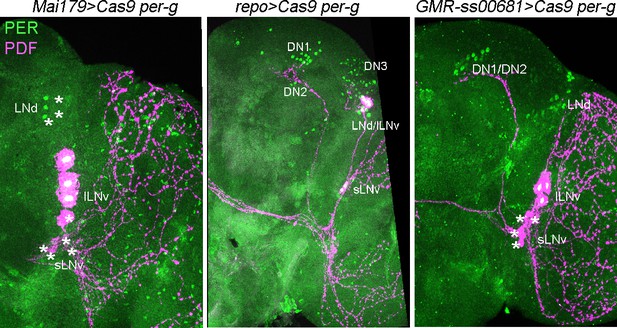
Immunolabeling of cell-specific per KO using Mai179-GAL4, repo-GAL4 and GMR-ss00681-split-GAL4.
Mai179-GAL4 is expressed in 3 out of 6 LNds, the sLNvs and shows weakly and variable expression in DN1 and lLNv neurons. We performed PER (green) and PDF (magenta) staining in Mai179>Cas9 per-g flies and found that 3 LNds were PER+, the sLNvs were PER- and one of the lLNvs was PER-. This nicely reproduces the expression pattern mentioned above. Asterix represents successful KO. Similarly, repo-mediated KO rendered glial cells PER-negative, whereas all clock neuron clusters remained PER-positive. GMR-ss00681-split-GAL4 drives expression in the sLNvs and specifically removes PER from these cells without affecting other clock neuron clusters.
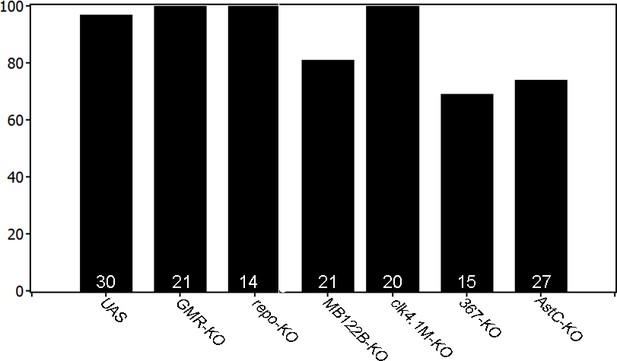
PER knockout in non-lateral neuron clusters does not affect rhythmicity levels.
All investigated genotypes showed high levels of rhythmicity, suggesting that the LNds and sLNvs are the key players in determining rhythmicity.
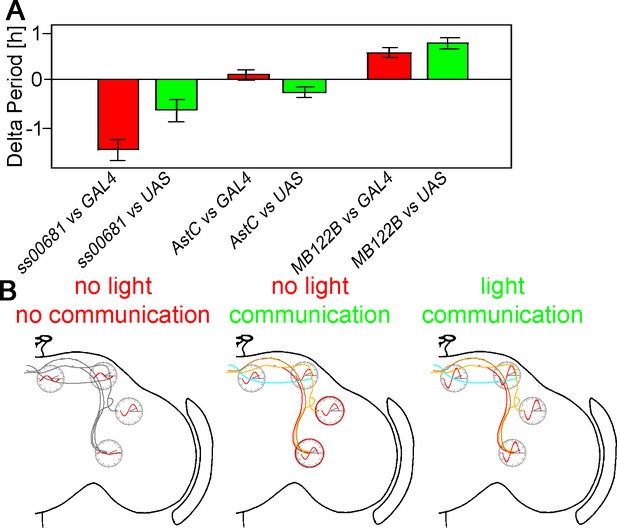
KO of PER in subsets of neurons changes free-running period.
(A) Changes of free-running period upon KO. Red bars represent the change of period between the KO line and the GAL4 control (± SEM), green bars represent the change of period between the KO line and the UAS control (± SEM). (B) Model of neuronal communication and light influencing the Drosophila clock machinery. Silencing the clock network in DD causes a damping of molecular oscillations and a drifting apart from the common phase as indicated by the red waves. If network communication is allowed, the different neuronal sub-clusters are mostly in sync and show robust cycling, suggesting neuronal communication is essential for molecular oscillations. In a normal LD cycle light drives high amplitude and synchronized cycling even in the absence of neuronal communication, establishing a hierarchy of synchronization cues with light on the top.
Tables
Changes in free-running period after PER knockout with different GAL4 drivers.
https://doi.org/10.7554/eLife.48301.013GAL4 line used | Number of flies | Change compared to GAL4 control | Change compared to UAS control |
---|---|---|---|
dv-PDF-GAL4 | 6 | −2.41 ± 0.37 | −0.79 ± 0.37 |
Mai179-GAL4 | 6 | −1.38 ± 0.68 | −1.07 ± 0.68 |
GMR-ss00681 | 12 | −1.39 ± 0.19 | −0.56 ± 0.19 |
GMR-ss00367 | 32 | −0.12 ± 0.13 | −0.14 ± 0.13 |
AstC-GAL4 | 16 | 0.11 ± 0.11 | −0.26 ± 0.11 |
GMR-ss1038 | 24 | 0.06 ± 0.11 | 0.06 ± 0.11 |
VGlut-GAL4 | 11 | 0.10 ± 0.11 | −0.26 ± 0.11 |
GMR-ss00849 | 22 | 0.55 ± 0.13 | 0.54 ± 0.13 |
MB122B | 21 | 0.57 ± 0.11 | 0.76 ± 0.11 |
Reagent type | Designation | Source or reference | Identifiers | Additional information |
---|---|---|---|---|
Genetic reagent (D. melanogaster) | clk856-GAL4 | Gummadova et al., 2009 | Flybase: FBtp0069616 | |
Genetic reagent (D. melanogaster) | UAS-Kir2.1 | Bloomington Drosophila Stock Center | BDSC_6595 | |
Genetic reagent (D. melanogaster) | pdf-GS-GAL4 | Depetris-Chauvin et al., 2011 | FBal0267534 | |
Genetic reagent (D. melanogaster) | per01 | Konopka and Benzer, 1971 | FBal0013649 | |
Genetic reagent (D. melanogaster) | mai179-GAL4 | Grima et al., 2004 | FBal0124017 | |
Genetic reagent (D. melanogaster) | MB122B-GAL4 | Guo et al., 2017 | ||
Genetic reagent (D. melanogaster) | pdf-GAL4 | Renn et al., 1999 | FBtp0011844 | |
Genetic reagent (D. melanogaster) | clk4.1M-GAL4 | Zhang et al., 2010 | FBtp0054012 | |
Genetic reagent (D. melanogaster) | UAS-Cas9.P2 | Bloomington Drosophila Stock Center | BDSC_58986 | |
Genetic reagent (D. melanogaster) | AstC-GAL4 | Bloomington Drosophila Stock Center | BDSC_52017 | |
Genetic reagent (D. melanogaster) | dvPDF-GAL4 | Guo et al., 2014 | FBtp0081543 | |
Genetic reagent (D. melanogaster) | w;CyO/Sco; MKRS/TM6B | Bloomington Drosophila Stock Center | BDSC_3703 | |
Genetic reagent (D. melanogaster) | VGlut-GAL4 | BloomingtonDrosophila Stock Center | BDSC_60312 | |
Genetic reagent (D. melanogaster) | GMR-ss00650-GAL4 | G Rubin, Janelia Research Campus | ||
Genetic reagent (D. melanogaster) | GMR-ss01038-GAL4 | G Rubin, Janelia Research Campus | ||
Genetic reagent (D. melanogaster) | GMR-ss00849-GAL4 | G Rubin, Janelia Research Campus | ||
Genetic reagent (D. melanogaster) | GMR-ss00367-GAL4 | G Rubin, Janelia Research Campus | ||
Genetic reagent (D. melanogaster) | GMR-ss00681-GAL4 | Liang et al. (2019) | ||
Genetic reagent (D. melanogaster) | GMR-ss00645-GAL4 | G Rubin, Janelia Research Campus | ||
Genetic reagent (D. melanogaster) | GMR-GAL4 | BloomingtonDrosophila Stock Center | BDSC_1104 | |
Genetic reagent (D. melanogaster) | repo-GAL4 | BloomingtonDrosophila Stock Center | BDSC_7415 | |
Genetic reagent (D. melanogaster) | tub-GAL80ts | Bloomington Drosophila Stock Center | BDSC_7018 | |
Genetic reagent (D. melanogaster) | UAS-per-g | This study | ||
Recombinant DNA reagent | pCFD6 | Addgene | RRID: Addgene73915 | |
Software | Fiji | https://fiji.sc/ | ||
Software | Stata SE15 | |||
Software | Microsoft Office Excel | |||
Software | ActogramJ | actogramj.neurofly.de | ||
Antibody | Anti-PER Rabbit polyclonal | Stanewsky et al., 1998 | 1:1000 | |
Antibody | Anti-PDF Mouse monoclonal | Developmental Studies Hybridoma Bank | AB_760350 | 1:500 |
Antibody | Anti-PDP1 Guinea pig polyclonal | Benito et al., 2007 | 1:2000 | |
Antibody | Goat anti-mouse polyclonal | ThermoFisher | A-31575 | 1:200 |
Antibody | Goat anti-rabbit polyclonal | ThermoFisher | A-11034 | 1:200 |
Antibody | Goat anti-rabbit polyclonal | ThermoFisher | A-32732 | 1:200 |
Antibody | Goat anti-guinea pig polyclonal | ThermoFisher | A-11073 | 1:200 |
Commercial assay or kit | MIDI-Prep Kit | Qiagen | 12143 | |
Commercial assay or kit | Q5 Polymerase | NEB | M0491S | |
Commercial assay or kit | BbSI-Enzyme | NEB | R0539S | |
Commercial assay or kit | Vectashield | Vectorlabs | H-1000 |
Additional files
-
Transparent reporting form
- https://doi.org/10.7554/eLife.48301.014