A new class of disordered elements controls DNA replication through initiator self-assembly
Figures
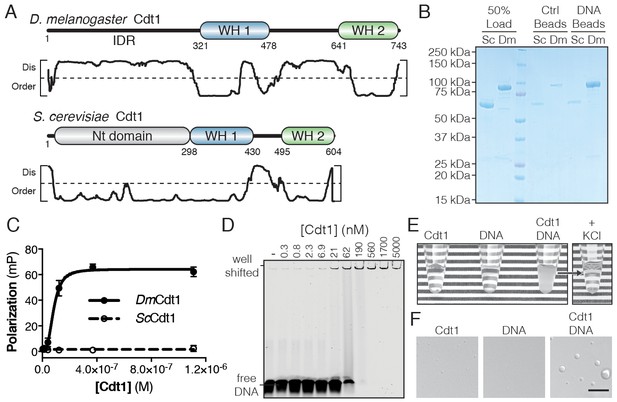
D. melanogaster Cdt1 undergoes DNA-dependent phase separation.
(A) Architecture of D. melanogaster and S. cerevisiae Cdt1. The per-residue DISOPRED (Jones and Cozzetto, 2015) disorder prediction score is shown in the plot below each gene, with a cutoff value of 0.5 indicated by the dashed line. Residues scored above this cutoff are predicted to be disordered. (B) dsDNA-coupled (‘DNA Beads’) and control (‘Ctrl Beads’) agarose beads were used to pull-down DmCdt1 and ScCdt1. DmCdt1 but not ScCdt1 bound to the DNA-coupled beads. (C) Fluorescence anisotropy measurements of Cdt1 binding to a Cy5-labeled duplex oligonucleotide. ScCdt1 showed no evidence of binding. DmCdt1 bound with a Kd, app = 83 ± 17 nM. (D) EMSA analysis of DmCdt1 binding to duplex DNA. The complex between DNA and DmCdt1 is heterogeneous and large, and is fully well-shifted at the highest concentrations. In good agreement with anisotropy measurements, the calculated Kd, app for DmCdt1 is ~100 nM. (E) Mixing concentrated DmCdt1 with duplex DNA results in a visible increase in solution turbidity that can be reversed with the addition of KCl. (F) DIC microscopy analysis of solutions of Cdt1, DNA, and a Cdt1/DNA mixture (scale bar = 5 µm). Phase-separated droplets were evident when Cdt1 was mixed with DNA. Gel images are representative from three independent experiments.
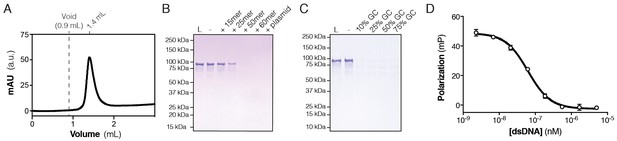
Sequence and length-dependence of Cdt1 phase separation.
(A) Cdt1 is monodisperse as assessed by analytical size-exclusion chromatography (SEC). 40 µM Cdt1 was run on a 5/150 Superdex S-200 in Tris/NaCl SEC Buffer (20 mM Tris pH 7.5, 150 mM NaCl, 10% glycerol, 1 mM BME). (B) Depletion assay to determine the effect of DNA oligonucleotide length on DNA-induced Cdt1 phase separation. Cdt1 phase separation is observed with oligonucleotides longer than 25 basepairs. (C) Depletion assay to determine the effect of DNA sequence on DNA-induced Cdt1 phase separation. Randomly generated 60 bp oligonucleotides containing between 10–75% GC content were assayed for the ability to induce Cdt1 phase separation. All are equally potent. (D) Fluorescence polarization-based competition assay. Unlabeled dsDNA is able to effectively compete with the DNA of a preformed Cdt1/Cy5-dsDNA complex (Kc, app = 64 nM, a value comparable to the observed Kd, app for DNA binding of 83 nM).
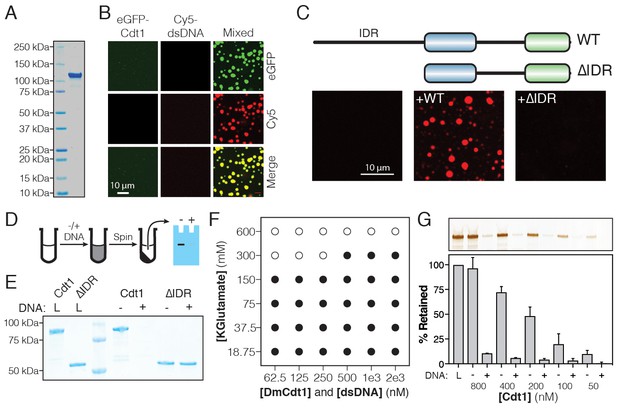
DmCdt1 phase separation is facilitated by an N-terminal IDR.
(A) SDS-PAGE analysis and Coomassie stain of purified eGFP-Cdt1. (B) Samples containing eGFP-Cdt1, Cy5-dsDNA (60 bp), and a mixture of eGFP-Cdt1 and Cy5-dsDNA (‘Mixed’) were prepared and analyzed by two-color fluorescent microscopy. Droplets were observed in the Mixed sample enriched for both protein (green) and nucleic acid (red). (C) Cy5-dsDNA was imaged alone or mixed with either wild-type Cdt1 (WT) or a Cdt1 construct lacking the N-terminal IDR (∆IDR). Only WT Cdt1 could induce droplet formation. (D) Schematic of a condensate depletion assay, a method for assessing phase separation (see Materials and methods for details). (E) The depletion assay was utilized to assess the role of the Cdt1 IDR in phase separation. DNA-induced the depletion of WT Cdt1 but not ∆IDR. (F) Phase diagram for Cdt1 in the presence of equimolar amounts of sixty basepair dsDNA (filled markers = phase separation observed, unfilled markers = phase separation not observed). (G) Depletion assay to assess phase separation at sub-physiological concentrations of Cdt1. DNA-induced phase separation of Cdt1 is seen at the lowest concentration tested (50 nM). Gel images are representative of three independent experiments.
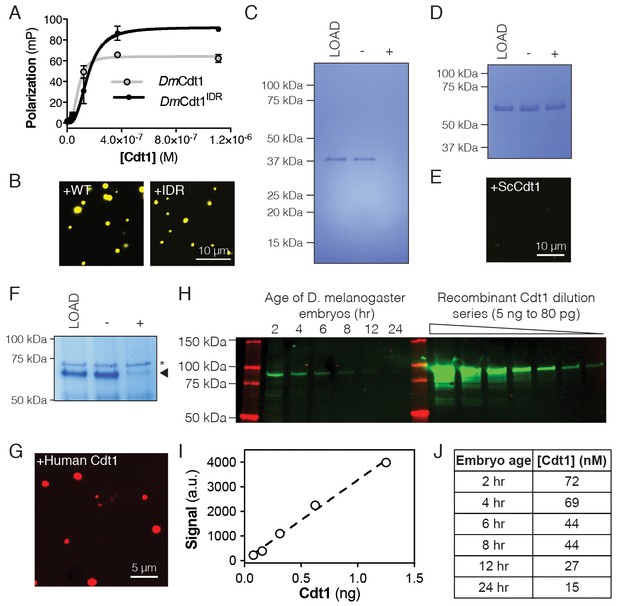
Properties of D. melanogaster Cdt1 phase separation.
(A) Fluorescence anisotropy measurements of the isolated D. melanogaster Cdt1 IDR (Cdt1IDR) binding to a Cy5-labeled duplex oligonucleotide. Cdt1IDR bound DNA with Kd, app = 158 ± 32 nM. WT Cdt1 is shown for comparison. (B–C) Cdt1IDR undergoes DNA-dependent phase separation as assessed by microscopy with a Cy3-labeled dsDNA (B, 5:5 µM protein:DNA) and by the depletion assay with unlabeled dsDNA (C, 2:2 µM protein:DNA). (D–E) S. cerevisiae Cdt1 (ScCdt1) shows no evidence of phase separation in either the depletion assay (D, 2:2 µM protein:DNA) or when mixed with Cy3-labeled dsDNA and imaged (E, 5:5 µM protein:DNA). (F) Human Cdt1 undergoes DNA-dependent phase separation as assessed by the depletion assay (F, 5:5 µM protein:DNA). The arrowhead indicates human Cdt1 and the asterisk a contaminating protein. (G) Human Cdt1 phase separates in the presence of Cy5-labeled dsDNA as assessed by fluorescence microscopy (10:10 µM protein:DNA). (H) Western blot analysis of DmCdt1 signal in embryos age 2–24 hr compared to a dilution series of purified recombinant Cdt1. (I) The five lowest-concentration western blot samples of recombinant Cdt1 (unsaturated signal) were plotted to generate a standard curve and fit by linear regression analysis. (J) Table of Cdt1 concentrations in embryos. The standard curve generated in (I) was used to quantitate Cdt1 concentration for each embryo time point in (H).
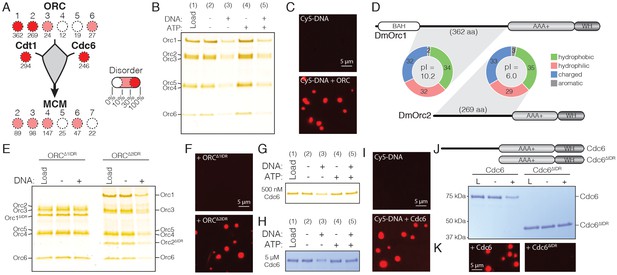
DmORC and DmCdc6 undergo DNA-dependent phase separation.
(A) Graphical comparison of the disorder for each Drosophila replication initiation factor. Orc1, Orc2, Cdc6, and Cdt1 each contain long IDRs (as denoted by the numbers under each circle) and a high percentage of overall disordered sequence (predicted >30%, depicted by color shading). (B) Analysis of ORC phase separation by the depletion assay. ORC (500 nM) phase separates in a DNA-dependent fashion in the presence and absence of ATP. (C) Cy5-dsDNA (2.5 µM) was imaged alone and as a mixture with ORC (2.5 µM). In the presence of ORC, large phase-separated droplets formed. (D) Two ORC subunits, Orc1 and Orc2, have large N-terminal IDRs. The IDR of Orc1 is longer and is enriched for positively-charged residues. (E) Analysis of ORC∆1IDR (500 nM) and ORC∆2IDR (500 nM) phase separation by depletion assay. Loss of the Orc1 IDR abolishes phase separation but loss of the Orc2 IDR has no effect. (F) Droplets form when Cy5-dsDNA (2.5 µM) is mixed with ORC∆2IDR (2.5 µM) but not when mixed with ORC∆1IDR (2.5 µM). (G) Cdc6 phase separation was assessed by depletion assay at 500 nM and (H) 5 µM concentrations. DNA induced Cdc6 phase separation but this was inhibited in the presence of 1 mM ATP. (I) Fluorescence imaging reveals phase-separated droplets when Cy5-dsDNA (2.5 µM) is mixed with Cdc6 (20 µM). (J) Phase separation analysis for a Cdc6 construct lacking the N-terminal IDR (Cdc6∆IDR). Cdc6∆IDR (500 nM) shows no depletion in the presence of DNA (500 nM). (K) Cdc6∆IDR (20 µM) is unable to induce droplet formation as assessed by fluorescence microscopy with Cy5-dsDNA (2.5 µM). Gel images are representative of three independent experiments.
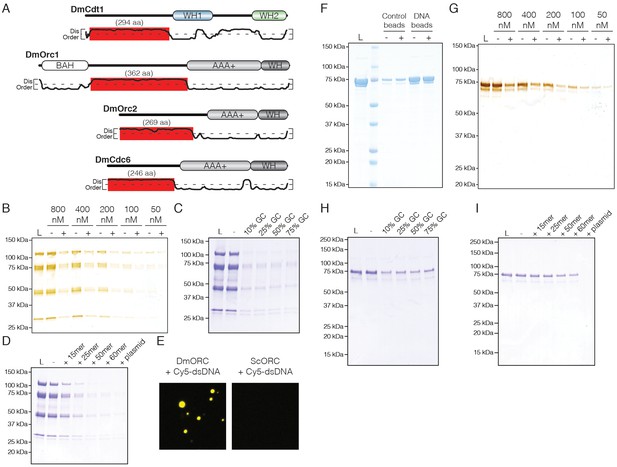
Analysis of ORC and Cdc6 phase separation.
(A) DISOPRED disorder predictions for the D. melanogaster initiators and location of their IDRs. (B) DNA-dependent phase separation was assessed for ORC over nanomolar concentrations. Depletion of ORC from the supernatant was observed at all concentrations tested. (C) Depletion assay to determine the effect of DNA sequence on DNA-induced ORC phase separation. Phase separation was induced with randomly generated 60 bp oligonucleotides containing between 10–75% GC content. No significant differences in potency are observed. (D) Depletion assay to determine the effect of DNA length on DNA-induced ORC phase separation. Phase separation is observed for all oligonucleotides tested; however, DNAs longer than 50 basepairs have a maximal effect. (E) D. melanogaster and S. cerevisiae ORC (DmORC and ScORC, respectively) were compared side-by-side for their ability to undergo DNA-dependent phase separation. 1.5 µM of each factor was mixed with stoichiometric amounts of Cy5-dsDNA and analyzed by fluorescence microscopy. DmORC but not ScORC was able to induce phase separation. (F) Analysis of DNA-binding by Cdc6 using a DNA pulldown assay. Binding was tested in the absence ("-") and presence ("+") of 1 mM ATP. Cdc6 bound specifically to DNA-coupled agarose beads in both the presence and absence of ATP. (G) DNA-dependent phase separation was assessed for Cdc6 at low nanomolar concentrations. Weak phase separating behavior, as evidenced by the depletion of Cdc6 from the supernatant, was observed at all concentrations tested. (H) Depletion assay to determine the effect of DNA sequence on DNA-induced Cdc6 phase separation. No significant differences in potency are observed. (I) Depletion assay to determine the effect of DNA length on DNA-induced Cdc6 phase separation. Phase separation is observed for oligonucleotides between 25–60 basepairs; however, plasmid DNA induces a maximal effect.
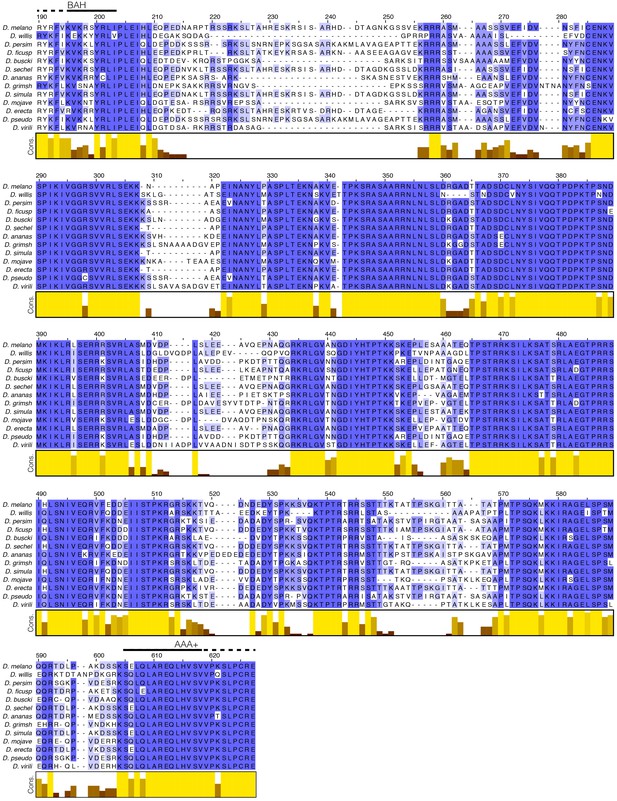
Multiple sequence alignment (MSA) of the Orc1 N-terminal IDR.
The Orc1 gene was aligned across multiple species in the Drosophila genus; only the region corresponding to the N-terminal IDR is displayed. Despite being unstructured, the N-terminal IDR is highly conserved across the Drosophila genus.
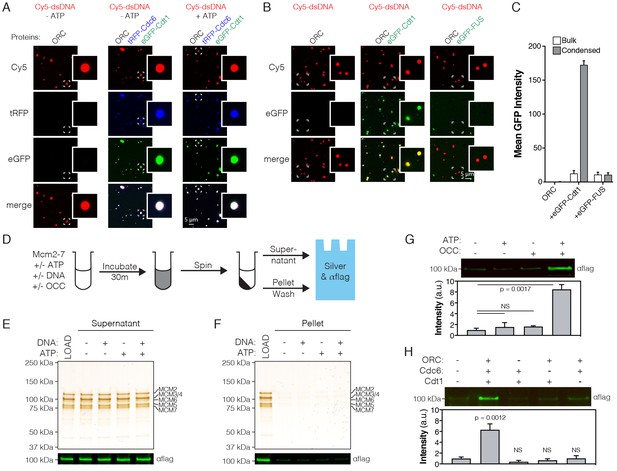
ATP-dependent recruitment of DmMcm2-7 into liquid phases containing DNA, Drosophila ORC, Cdc6 and Cdt1.
(A) Analysis of tRFP-Cdc6 (2.5 µM) and eGFP-Cdt1 (2.5 µM) recruitment to pre-formed ORC/Cy5-dsDNA (2.5/2.5 µM) droplets in the absence (‘-ATP’) and presence (‘+ATP’) of ATP (1 mM). (B) eGFP-Cdt1 (500 nM) or eGFP-FUS (500 nM) was added to reactions and assessed for the ability to co-localize with preformed ORC/Cy5-dsDNA droplets (2.5/2.5 µM). In samples containing eGFP-Cdt1, all ORC/Cy5-dsDNA droplets are enriched for eGFP signal. No enrichment is observed in samples containing eGFP-FUS.). (C) Quantitation of eGFP signal intensity within and outside of ORC/Cy5-dsDNA droplets for samples in panel (B). (D) Schematic of a depletion assay to assess Mcm2-7 phase partitioning; both the supernatant and pellet were assessed for either the loss or enrichment, respectively, of Mcm2-7. (E) Mcm2-7 (500 nM) was not depleted from the dilute phase and, consistently, was absent from the condensed phase (F). (G) Loading reactions were prepared that contained either ORC, Cdc6, and Cdt1 (OCC) or ATP, or both the OCC and ATP, and phase separation of Mcm2-7 was assessed by the depletion assay. In the presence of both the OCC and ATP, Mcm2-7 is significantly enriched in the condensed phase. (H) Loading reactions were set up that contained both ATP and DNA, but from which individual initiators were removed (ORC, Cdc6, or Cdt1), and the depletion assay performed. In the absence of any one initiator, Mcm2-7 no longer partitions to the pelleted phase.
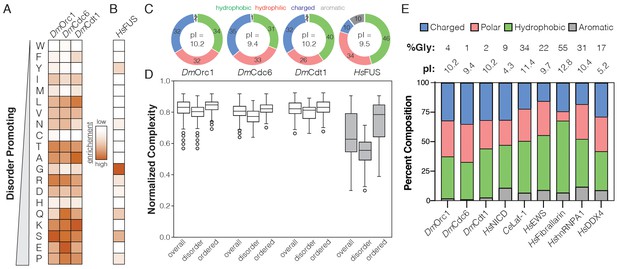
Replication initiation factors form a new class of phase separating IDRs.
(A) Heatmap analysis of the sequence composition for initiator N-terminal IDRs compared to (B) the human FUS (HsFUS) intrinsically disordered regions. The order of amino acids in the heatmap is arranged according to the propensity of each to promote disorder. (C) Classification of the disordered sequences in panels (A–B) by percent hydrophobic, hydrophilic, charged, and aromatic residues. The pI of each protein is displayed within the center of the pie chart. (D) For Orc1, Cdc6, Cdt1, and HsFUS, the per residue complexity score was calculated for each amino acid within a 20 amino acid window and plotted as box and whiskers. A plot was generated for all residues within the protein (overall) and compared to plots of the extracted ordered and disordered regions. (E) Categorical analysis of the D. melanogaster initiators compared to other known phase-separating proteins. The glycine composition (%Gly) and pI for each factor is indicated at the top of the chart.
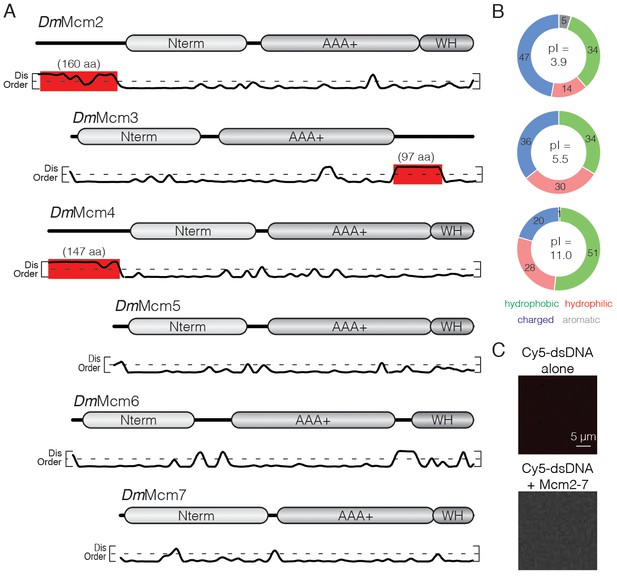
Analysis of D. melanogaster Mcm2-7 IDRs.
(A) The architecture of each Mcm2-7 gene is illustrated with the per-residue DISOPRED disorder prediction score plotted below. Only Mcm2, Mcm3, and Mcm4 contain extended regions of disorder (>75 aa, highlighted in red). (B) Categorical analysis of the IDRs of Mcm2 (upper), Mcm3 (middle), and Mcm4 (lower). (C) Cy5-dsDNA (1.5 µM) was imaged alone or mixed with Mcm2-7 (1.5 µM). No droplets were observed, demonstrating that Mcm2-7 does not phase separate on its own.
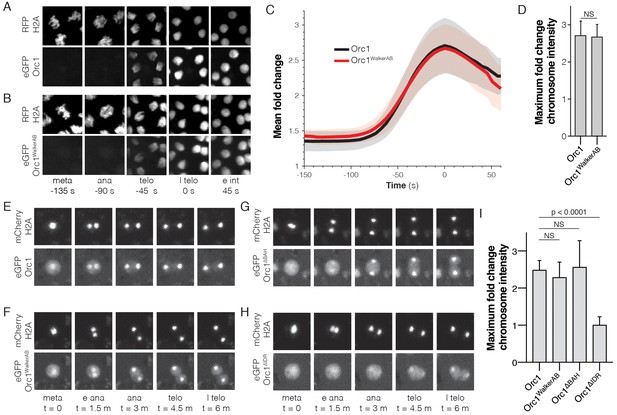
The Orc1 N-terminal IDR, but not ATP binding, is required for chromatin recruitment.
(A) Representative maximal intensity projections of time series images of a mitotic event in D. melanogaster embryos expressing eGFP-Orc1 and His2A-RFP (‘meta’=metaphase, ‘ana’=anaphase, ‘telo’=telophase, ‘l telo’=late telophase, and ‘e int’=early interphase). Loading of eGFP-Orc1 onto chromosomes reached a maximum in late telophase (t = 0). (B) As in (A), but with eGFP-Orc1WalkerAB and His2A-RFP embryos. (C) Quantitative analysis of the fold-change in eGFP signal intensity on chromosomes as cells progress through mitosis. Time is registered with respect to late telophase where maximum loading was observed. (D) No difference in maximum fold intensity was observed between eGFP-Orc1 and eGFP-Orc1WalkerAB. (E–H) Analysis of Orc1 chromatin association in S2 cells transiently transfected with mCherry-His2A and either wild-type Orc1 (E), Orc1WalkerAB (F), Orc1∆BAH (G), or Orc1∆IDR (H) (‘meta’=metaphase, ‘e ana’=early anaphase, ‘ana’=anaphase, ‘telo’=telophase, and ‘l telo’=late telophase). (I) Quantitative analysis of the fold-change in eGFP signal intensity observed on telophase chromosomes for each of the Orc1 constructs (E–H). No significant difference was observed between WT, Orc1WalkerAB, and Orc1∆BAH, but Orc1∆IDR was not recruited to chromosomes.
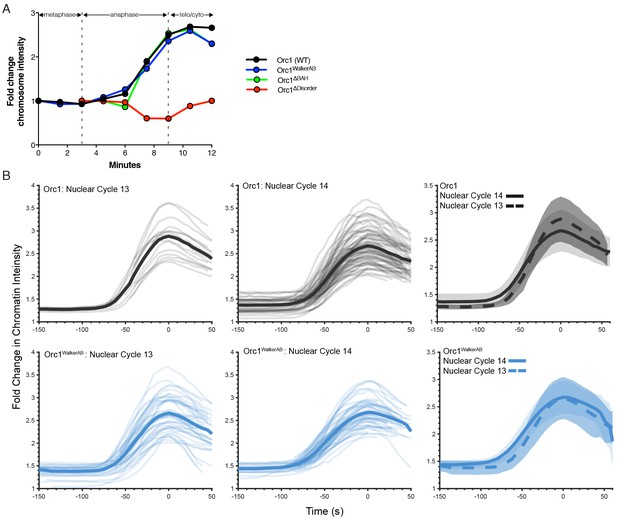
Dynamics of Orc1 chromosome recruitment in tissue culture cells and embryos.
(A) Quantitative analysis of the fold-change in eGFP-Orc1 (black), eGFP-Orc1WalkerAB (blue), eGFP-Orc1∆BAH (green) and eGFP-Orc1∆IDR (red) signal intensity on chromosomes as S2 tissue culture cells progress through mitosis. Each line corresponds to a representative trace from a single mitotic event for each construct. (B) Quantitative analysis of eGFP-Orc1 enrichment for nuclear cycles 13 and 14. The fold-change in chromatin signal intensity for eGFP-Orc1 (top three graphs) and eGFP-Orc1WalkerAB (bottom three graphs) was quantitated and compared between nuclear cycle 13 (left column graphs) and nuclear cycle 14 (middle column graphs). The fold change in chromatin intensity is not statistically different between these cycles (right column graphs).
Mitotic dynamics of eGFP-Orc1. eGFP-Orc1 (green) loads onto chromosomes (His2A-RFP, white) beginning in anaphase and reaches a maximum in telophase.
Slowing the playback speed highlights the precise mitotic stage at which Orc1 begins loading.
Mitotic dynamics of eGFP-Orc1WalkerAB.
As seen for WT Orc1, eGFP-Orc1WalkerAB (green) loads onto chromosomes (His2A-RFP, white) beginning in anaphase and reaches a maximum in telophase. Slowing the playback speed highlights the precise mitotic stage at which Orc1WalkerAB begins loading.
Example of chromatin segmentation from the His2A-RFP signal.
https://doi.org/10.7554/eLife.48562.020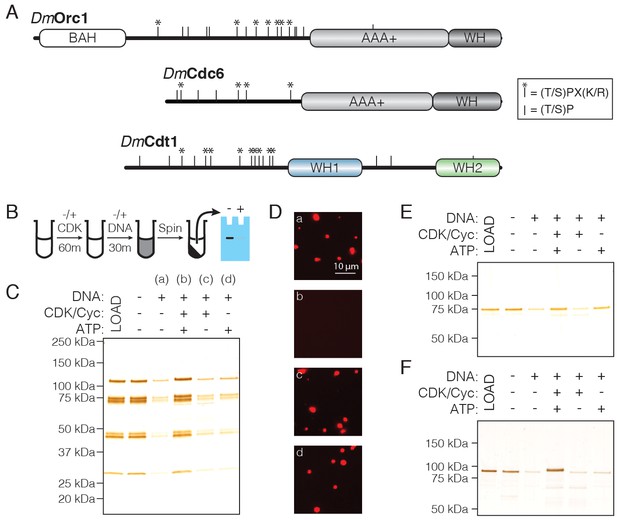
CDK/Cyclin-dependent phosphorylation of initiators regulates phase separation.
(A) Schematic of CDK/Cyc phosphorylation consensus sequences across the DmOrc1, DmCdc6, and DmCdt1 proteins. Tic marks along each sequence represent the minimum consensus sequence ([T/S]P); those denoted with an asterisk (*) indicate the full consensus sequence ([T/S]PX[K/R]). (B) Schematic of the depletion assay used to assess the effect of phosphorylation on phase separation. (C) Depletion assay with untreated ORC or ORC treated with CDK1/CycA/ATP, CDK1/CycA alone, or ATP alone. No depletion is observed when ORC is pre-treated with CDK1/CycA/ATP, but either reagent alone has no effect. (D) Fluorescence microscopy analysis of samples in (C) confirms that phosphorylated ORC is unable to induce phase separation (‘a’, untreated ORC; ‘b’, ORC treated with CDK1/CycA/ATP; ‘c’, ORC treated with CDK1/CycA; ‘d’, ORC treated with ATP). (E) Analysis of Cdc6 depletion under conditions described in (C). Cdc6 phase separation is inhibited in the presence of CDK1/CycA/ATP, as well as by ATP alone. (F) Analysis of Cdt1 depletion under conditions described in (C). Cdt1 phase separation is fully inhibited when treated with CDK1/CycA/ATP. A slight decrease in Cdt1 mobility is seen in the phosphorylated reaction.
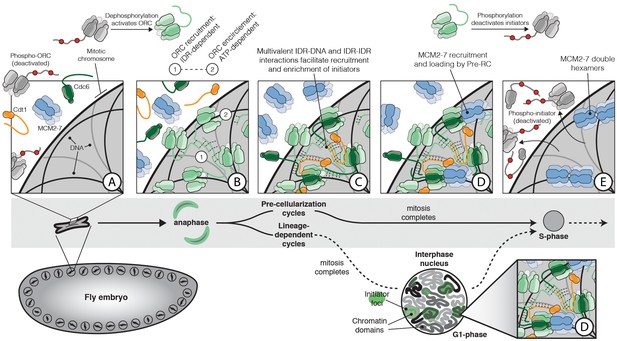
The role of IDR- and DNA-dependent initiator co-association in replication initiation.
Prior to mitosis, ORC exists in an inhibited state incompetent for chromatin recruitment (A). At the metaphase to anaphase transition (A–B), D. melanogaster ORC is activated, possibly in part through IDR dephosphorylation, thereby driving the condensation of ORC onto the surface of chromosomes (B). The Orc1 IDR likely plays a key role in this event, both interacting with DNA and also participating in intermolecular IDR-IDR interactions that drive ORC enrichment on chromosomes. The spatial patterning of ORC is developmentally regulated and appears correlated with the establishment of chromatin territories and TADs. Before cellularization (top, ‘Pre-cellularization cycles’), the multi-nucleate fly embryo undergoes synchronous divisions that lack a G1 phase. At this stage, ORC shows homogenous chromosome binding. Conversely, ORC is non-uniformly distributed in differentiated cells (bottom, ‘Lineage-dependent cycles’) where replication domain boundaries coincide with underlying features of chromatin architecture. Once ORC is bound to chromosomes, Cdc6 and Cdt1 are co-recruited through direct interactions with DNA and through inter-initiator IDR-IDR interactions (C). When the full suite of helicase loading factors are present, the Pre-RC forms and Mcm2-7 loading commences (D). Loading is terminated by the phosphorylation of initiator IDRs which displace them from chromatin and inhibit re-association (E).
Tables
Conservation of initiator IDR sequence features.
Analysis of eukaryotic initiator homologs for the presence of an N-terminal IDR, as well as IDR sequence features (‘pI’=isoelectric point; ‘FCR’=fraction charged residues).
Orc1 | Cdc6 | Cdt1 | |
---|---|---|---|
D. melanogaster N-term IDR length: pI: FCR: | 362 aa 10.2 0.32 | 246 aa 9.4 0.35 | 294 aa 10.1 0.32 |
Human N-term IDR length: pI: FCR: | 300 aa 10.7 0.33 | 136 aa 10.6 0.29 | 175 aa 10.6 0.29 |
Mouse N-term IDR length: pI: FCR: | 298 aa 10.2 0.34 | 141 aa 10.0 0.31 | 178 aa 9.8 0.24 |
X. laevis N-term IDR length: pI: FCR: | 327 aa 9.7 0.33 | 140 aa 10.8 0.26 | 248 aa 10.1 0.31 |
C. elegans N-term IDR length: pI: FCR: | 242 aa 9.1 0.39 | 172 aa 9.9 0.38 | 194 aa 10.3 0.35 |
D. rerio N-term IDR length: pI: FCR: | 391 aa 9.8 0.31 | 156 aa 11.1 0.22 | 309 aa 9.9 0.31 |
S. cerevisiae N-term IDR length: pI: FCR: | 143 aa 4.7 0.49 | 31 aa 6.2 0.32 | N/A N/A N/A |
S. pombe N-term IDR length: pI: FCR: | 117 aa 10.6 0.36 | 133 aa 10.2 0.22 | N/A N/A N/A |
Rescue of Orc1 null allele (orc14739) by WT Orc1 and WalkerAB mutant transgenes.
https://doi.org/10.7554/eLife.48562.015orc14739/Cyo; tg/tg | orc14739/Cyo; tg/TM3 | orc14739/orc14739; tg/tg | orc14739/orc14739; tg/TM3 | |
---|---|---|---|---|
WT eGFP-Orc1 | 223♀ 209♂ | 347♀ 428♂ | 86♀ 80♂ | 196♀ 186♂ |
eGFP-Orc1WalkerAB | 413♀ 396♂ | 622♀ 847♂ | 0♀ 0♂ | 0♀ 0♂ |
eGFP-Orc1∆IDR | 360♀ 365♂ | 617♀ 655♂ | 0♀ 0♂ | 0♀ 0♂ |
Reagent type (species) or resource | Designation | Source or reference | Identifiers | Additional information |
---|---|---|---|---|
Recombinant DNA reagent | 2Cc-T | QB3 Macrolab (UC Berkeley) | RRID:Addgene_37237 | Ligation independent cloning (LIC); E. coli expression vector |
Recombinant DNA reagent | 1GFP | QB3 Macrolab (UC Berkeley) | RRID:Addgene_29663 | LIC cloning; E. coli expression vector |
Recombinant DNA reagent | 1b | QB3 Macrolab (UC Berkeley) | RRID:Addgene_29653 | LIC cloning; E. coli expression vector |
Recombinant DNA reagent | pFastbac1 | ThermoFisher | Insect cell expression vector | |
Recombinant DNA reagent | 438A | QB3 Macrolab (UC Berkeley) | RRID:Addgene_55218 | LIC cloning; insect cell expression vector |
Recombinant DNA reagent | 438B | QB3 Macrolab (UC Berkeley) | RRID:Addgene_55219 | LIC cloning; insect cell expression vector |
Recombinant DNA reagent | 4C | QB3 Macrolab (UC Berkeley) | RRID:Addgene_30116 | LIC cloning; insect cell expression vector |
Recombinant DNA reagent | pattB | Fragments inserted into MCS by restriction enzyme cloning; transgene vector | ||
Recombinant DNA reagent | pCopia-LIC | This paper | LIC cloning; D. melanogaster cell culture expression vector | |
Peptide, recombinant protein | TEV | QB3 Macrolab (UC Berkeley) | Used at 1/10 (weight/weight) TEV/substrate ratio | |
Peptide, recombinant protein | Flag peptide | Sigma-Aldrich | ||
Strain, strain background (Escherichia coli) | Rosetta 2(DE3)pLysS | QB3 Macrolab (UC Berkeley) | Chemically competent cells | |
Strain, strain background (Escherichia coli) | DH10bac | QB3 Macrolab (UC Berkeley) | Chemically competent cells | |
Cell line (D. melanogaster) | S2 | UC Berkeley Cell Culture Facility | ||
Cell line (Spodoptera frugiperda) | Sf9 | UC Berkeley Cell Culture Facility | ||
Cell line (Trichoplusia ni) | High5 | This paper; UC Berkeley Cell Culture Facility | ||
Commercial assay or kit | ANTI-FLAG M2 Affinity Agarose Gel | Sigma-Aldrich | A2220 (RRID:AB_10063035) | 0.5 mL resin per 1 L expression |
Commercial assay or kit | Amylose Resin | NEB | E8021L | 5 mL of resin; column format |
Commercial assay or kit | High Capacity Strepatavidin Agarose | ThermoFisher Scientific | 20357 | |
Commercial assay or kit | Effectene | Qiagen | 301425 | |
Antibody | Anti-Cdt1 affinity purified antibody (rabbit polyclonal) | M. Botchan | 1/1,000 dilution | |
Antibody | IRDye800 CW Donkey anti-Rabbit (donkey polyclonal) | LI-COR | 926–32213 (RRID:AB_621848) | 1/10,000 dilution |
Antibody | Anti-FLAG (rabbit monoclonal) | Sigma-Aldrich | F7425 | 1/1,000 |
Additional files
-
Transparent reporting form
- https://doi.org/10.7554/eLife.48562.021